Current and future applications of catheter-based interventional magnetic resonance imaging for diagnosis and therapy of vascula
Images
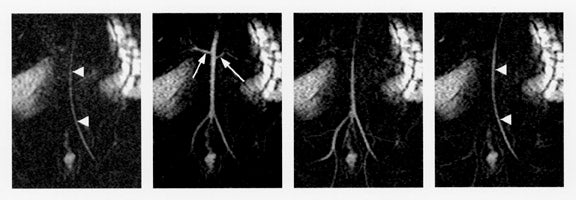
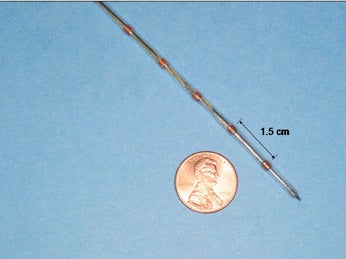
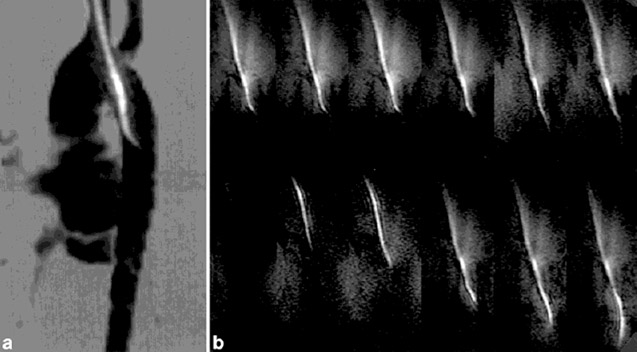

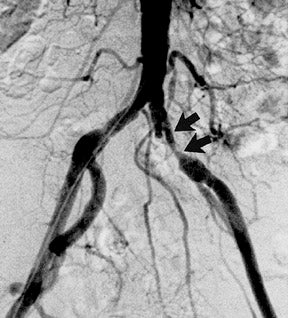
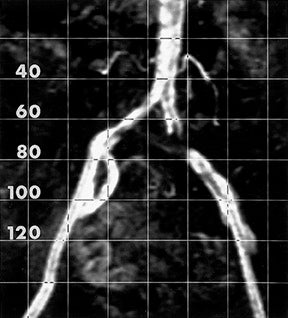
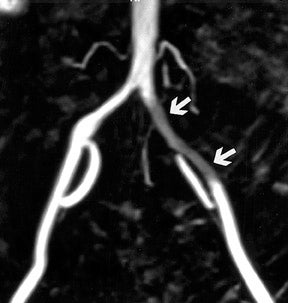
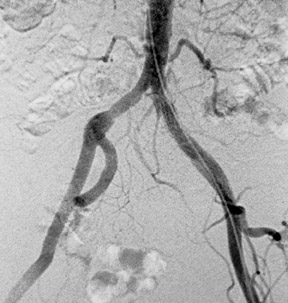
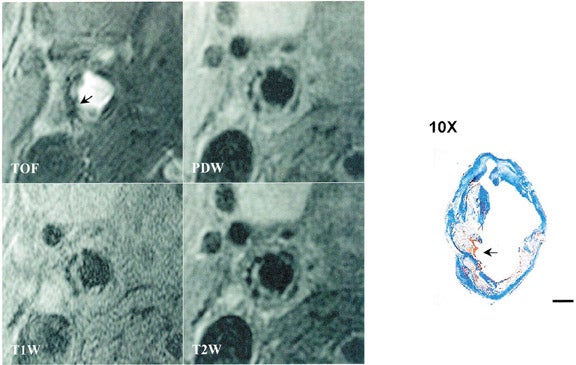
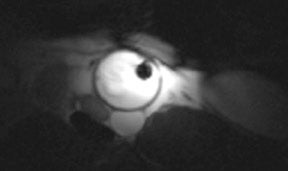
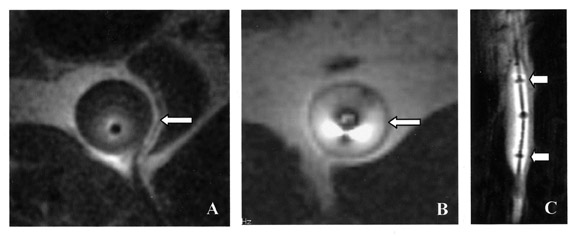
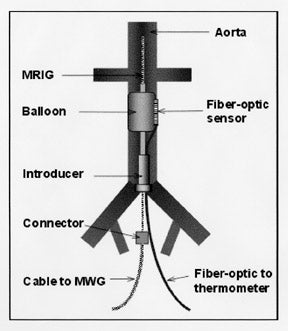
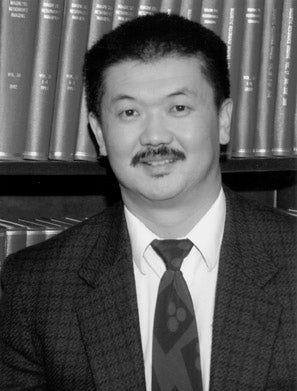
Dr. Morimoto is currently a 4th-year Radiology Resident at Vanderbilt University Medical Center, Nashville, TN. He received his MD from the University of New Mexico in 1999. Prior to obtaining his MD, he was involved in robotics research for 18 years, with the later years focused on biomedical technology development. He owns four U.S. patents. He will begin a 2-year Neuroradiology Fellowship at the University of Utah, Salt Lake City in 2004.
Catheter-based interventional magnetic resonance imaging (CBiMRI) is an emerging area of intense interest whereby intravascular catheters are manipulated under magnetic resonance (MR) imaging guidance for a variety of diagnostic and therapeutic indications. Applications range from the treatment of patent foramen ovale to gene therapies for cardiovascular disease.
CBiMRI not only allows visualization of the lumen of the blood vessel, the vessel wall, and all aspects of the anatomy within the field of view (FOV), 1,2 but also evaluates flow through the vessels 3-6 and function of the solid organs 7 supplied by the vessels. For example, blood-oxygenation-leveldependent (BOLD) functional MRI takes advantage of deoxyhemoglobin in areas of ischemia to establish activation maps for investigating motor function recovery. 8 Although positron emission tomography is the gold standard, it is limited by accessibility and it cannot be implemented for real-time applications of catheter intervention. Computed tomography (CT) can also be utilized for catheter interventions; however, radiation exposure limits its use in the pediatric population. CT is also limited in its ability to characterize soft tissue as compared with MRI. In comparison, conventional catheter angiography shows the vessel lumen well but provides little extravascular anatomical detail--it has been described as lumenography.
Prior work
Passive catheter tracking
CBiMRI is necessarily dependent on tracking the catheter tip, as is conventional angiography. Passive localizing and tracking a catheter is best accomplished by using MR projection imaging in a fashion analogous to conventional X-ray angiography. 9 This is accomplished by using a thick imaging slice, thus optimizing the probability that the catheter or vessel will fall within the imaging FOV. One method of catheter tracking is accomplished using a two-dimensional (2D) inversion recovery (IR)prepared fast low-angle shot (FLASH) spoiled gradient-echo sequence (Figure 1). Several methods for passively visualizing the catheter itself include filling the catheter with contrast, filling a balloon on the catheter tip with carbon dioxide to generate a void, or placing contrast within the balloon.
Active catheter tracking
Active catheter tracking is implemented by incorporating a
radiofrequency coil or antenna into an interventional device, such
as a guidewire or catheter.
10,11
An example of a multicoil catheter is provided in Figure 2. The
term "active" implies that the catheter and/or guidewire are
generating a signal as a direct result of the increased signal
generated from the guidewire or radiofrequency (RF) coil. Catheter
position within the 2D projection slab can be "visually" monitored
based on the strong signal detected by
this coil (Figure 3).
Active tracking can also have a very different meaning; rather than just allowing visualization of the catheter, one can generate spatial coordinates for the catheter position in three-dimensional (3D) space and register with the MR image set for visualization. 13 The small MRI receive coils incorporated into the catheter generate transverse spin magnetization when the transmit coil (typically, the body coil) emits a nonselective RF pulse. The resultant MRI signal from the small tracking coils is detected. The MRI signal is then processed to determine the X, Y, and Z coordinates of the coils. This information can then be registered with the MR image.
Safety concerns Although active catheter tracking produces a strong MR signal and high temporal resolution, there are several practical limitations. Adding a coil to the tip of a catheter can alter its mechanical properties, 14 which can impede catheter movements within tortuous blood vessels. Only the portion of the catheter or guidewire possessing the RF receiver coil will be imaged, and buckling of the nonvisualized portions of the catheter may impede manipulation or may cause injury. Active methods may also cause tissue heating due to the RF coils placed within the body. 15 Theory and experiments have shown an electric-fielddependent heating of the ends of long wires of as much as 6°C. 16 Other researchers 17 found increases in the guidewire tip temperature from 26°C to 74°C after 30 seconds of scanning in a 1.5T magnet, presumably resulting from resonant waves within the wire. Still others found a 17°C increase in temperature with nitinol wires in a 1.5T magnet. 18 Temperature increases of this magnitude can injure tissue and cause blood coagulation, which is why many researchers have investigated alternatives to metallic wires. Quarter-wavelength chokes are proposed to remedy the heating problem. 19
Clinical applications
Clinical experience with CBiMRI has primarily taken place within the European research community. Two such examples follow.
Congenital heart disease diagnosis and therapy
Congenital heart anomalies have a reported incidence varying from 3 to 12 per 1000 live births, depending on the methods used for detection and diagnosis. Ventricular septal defects are the most common at 29%. A child who is born with cyanosis will be diagnosed promptly with a congenital heart defect because of clinical presentation. Heart disease may go undiagnosed for years in asymptomatic patients. The most common defect with associated cyanosis is tetrology of Fallot. 20
Current methods of diagnosis rely on conventional angiographic techniques that can result in substantial radiation exposure. Recent research has shown the need to reduce radiation exposure, especially in children to minimize the probability of developing radiation-related neoplasms later in life. 21,22 One pooled study reported a linear relationship between exposure and relative risk of thyroid cancer in children younger than 15 years of age. 23 Research also showed that radiologic studies as low as 0.1 Gy result in a statistically significant increase in relative risk for subsequent thyroid cancer development.
Razavi 24 used a combined X-ray fluoroscopy and MR imaging (XMR) to carry out cardiac catheterization on children and adults with congenital heart disease, with the goal of decreasing radiation exposure and utilizing flow and cardiac motion information provided by MRI to improve on diagnostics and therapy. Eight diagnostic cardiac catheterizations, 1 device closure of atrial septal defect, 1 intracardiac coil occlusion, and 2 radiofrequency ablations for tachycardia were performed. Catheters were placed in all chambers of the heart and great vessels by MR guidance, with the tip of the catheter visualized by means of passive contrast from a carbon dioxide balloon. Using a movable tabletop, researchers moved the patients between a 1.5T Philips Intera 1-T MRI scanner and a Philips Pulsera cardiac X-ray unit (both products from Philips Medical Systems, Bothell, WA) that were co-located. Because the MR flexible coil arrays are sufficiently radiotranslucent, they could be left in place during X-ray imaging without image quality degradation. The Intera MRI system provided 10 to 20 frames-per-second imaging for passive catheter tracking with a carbon-dioxidefilled balloon at the catheter tip by using MR-compatible catheters without guidewires. Three-dimensional imaging, flow quantification, and myocardial motion assessment provided clinically useful information not otherwise available by using conventional angiographic techniques. Figure 4 shows passive catheter manipulation under MR guidance. Razavi 24 found that radiation exposure was significantly decreased while clinically useful information regarding flow and cardiac motion was provided that would otherwise not be available using conventional techniques.
Diagnosis and therapy of atherosclerosis Heart disease and stroke, the principal sequelae of cardiovascular disease, are the first and third leading causes of death in the United States, accounting for >40% of all deaths. Approximately 950,000 Americans die of heart disease or stroke each year, which amounts to 1 death every 33 seconds. Atherosclerosis is by far the most common cause of arterial stenosis. Although new developments continue to shed light on the pathogenesis, one of the most widely accepted hypotheses, formulated by Ross, 25 explains atherosclerosis as a response to injury. Atherosclerosis produces symptoms through blood flow reduction, thrombotic occlusion, plaque ulceration with distal embolization, and, rarely, by penetration into the media.
Atherosclerotic disease of the iliac arteries is typically diffuse and bilateral with clinically significant disease in the distal aorta extending into the common iliac arteries. The disease is unusual in people younger than 40 years, and men are more frequently affected than women. 26
Current methods of therapy include medical management for patients with mild to moderate claudication or interventional methods. Interventional methods include conventional balloon angioplasty and stenting or surgical graft placement. The choice between percutaneous methods or surgery depends on the nature and location of the stenosis. Studies have shown that iliac stenosis can be treated with percutaneous angioplasty with a 95% technical success rate and 1- and 3-year patency rates of 80% and 70%, respectively. With conventional stent placement, the patency rates improve to 90% and 85%, respectively. 26
To assess the feasibility of MR-guided stent placement, Manke 27 studied the treatment of focal iliac stenoses in patients with chronic limb ischemia by using passive catheter-tracking in a conventional 1.5T magnet. Even though the mean degree-of-stenosis after stent placement was significantly higher at contrast-enhanced MR angiography than at digital subtraction angiography (DSA) (24.6% versus 6.2%) and the mean procedure time was 74 minutes, Manke 27 successfully treated the iliac stenoses in 10 of 13 patients by using MR imaging-guided intervention alone. The other 3 patients were treated with additional fluoroscopic guidance, because complications (ie, panic attack, subintimal recanalization, and stent misplacement) occurred with MR guidance. Postprocedure stent placement evaluation using MR angiography was expectedly limited owing to stent-induced signal loss of the lumen (Figure 5).
Manke et al 27 used a 1.5T MR imaging unit (Magnetom Symphony; Siemens, Erlangen, Germany) with a circular polarized, phased-array body coil. Coronal contrast-enhanced 3D fast imaging with steady-state precession MR angiography was used to verify the intraluminal position of the catheter and localize the stenosis (Figure 6), as well as for post-interventional evaluation. Stent placement and balloon angioplasty were monitored with a 2D fast low-angle shot (FLASH) sequence with flow compensation. The acquisition time for a single section was 1.88 seconds per image, with a delay of approximately 0.5 second between acquisition and display.
Future work
One promising area for future CBiMRI research is in diagnosis and treatment of atherosclerotic disease. A substantial amount of work has already been undertaken to improve imaging of the disease with using conventional and catheter-based imaging, although this research will not be discussed here.
Neovascularity is thought to be involved in the pathogenesis of atherosclerotic plaque. 28,29 New theories, based on pathologic studies, have shown an association between the degree of neovascularity and the presence of inflammatory cells. 30 With neovascularity, inflammatory cells are recruited to form the lipid core of a plaque. 31 The presence and degree of neovascularity within unstable plaque 32-35 are potential markers for vulnerability.
Contrast-enhanced MRI of the carotid arteries provides significant differentiation of neovascularity from other nonvascular regions within unstable carotid plaques. 36 In addition, MRI identification of fibrous cap rupture (Figure 7) is strongly associated with patients who have a recent history of transient ischemic attack or stroke. 37 Using a modified American Heart Association classification for carotid plaque, researchers have demonstrated that high-resolution MRI can detect characteristics of vulnerable plaque with a high degree of sensitivity and specificity, 38 such as: a thin or ruptured fibrous cap, a large lipid or necrotic core, and intraplaque hemorrhage. Quantification of the degree of neovascularity, 39 based on dynamic contrast enhanced studies, provides a means for prospectively studying the link between neovasculature and plaque vulnerability.
Conventional MR imaging has some limitations because most arteries are internal to the body at a distance from the skin surface, where surface coils provide limited benefit. To address this issue, investigators such as Hillenbrand et al 40 (Figure 8), Qiu et al 41 (Figure 9), and others have studied high-resolution imaging of the vessel walls with intravascular imaging coils incorporated into a conventional catheter. Promising improvements can be seen in vessel-wall definition with resolution on the order of several hundred microns.
Various options for therapy exist to treat carotid
atherosclerosis. Medical management, including cholesterol-lowering
therapies, has been explored.
42
In a prospective study of 18 patients, atherosclerotic plaques
showed regression in size after Simvastatin (Merck Pharmaceuticals,
Darmstadt, Germany) therapy by using high-resolution MR. Reduction
in plaques occurred after
12 months of therapy without significant change in the lumen
diameter. This supports the hypothesis that lipid-lowering
medications help to stabilize the plaque. This study also helps to
explain why prior conventional catheter angiographic studies showed
minimal change in lumen diameter in patients after
cholesterol-lowering therapy.
43
The physiologic changes were occurring within the vessel wall, not
visualized with conventional angiography.
Gene therapy is also being investigated to treat atherosclerosis. 41 Using catheter-based delivery, researchers are performing efficient, high-dose transfer of genes into the endothelial cells and smooth muscle cells of the target vessels during balloon angioplasty while minimizing any undesirable systemic transfection. 44
Prior in vitro studies showed improved transfection by using a specialized catheter with application of heat. 45-47 The intravascular heating coils also provide for high-resolution intravascular imag-ing as has been shown by Qiu et al. 41 Researchers positioned a 0.032-inch MRI guidewire along with a 5F balloon and a 0.6-mm fiber-optic temperature sensor, into the aorta of each of 6 New Zealand white rabbits (Figure 10). Then, while inflating the balloon with saline, researchers heated the targeted aorta for 20 minutes by operating the catheter microwave generator at 20 to 25 W, resulting in a temperature increase to 41°C at the target aortic wall. High-resolution axial and sagittal images were obtained in a 1.5T MRI system (GE Medical System, Milwaukee, WI). During MR imaging, the MRI was connected to the MRI preamplifier and operated in the receive-only mode for high-resolution imaging. In early studies using gene therapy, Qiu et al 41 have demonstrated longer-term vessel patency in the gene-therapy treated iliac arteries with atherosclerosis as compared with the contralateral iliac artery treated with angioplasty alone.
Conclusion
Catheter-based interventional MRI is proving to be a powerful tool in the diagnosis and therapy of vascular and cardiac diseases. Treatment of congenital heart disease in the pediatric population, in particular, is one application that provides a great benefit while minimizing the hazards of ionizing radiation. Recent developments in high-resolution imaging of atherosclerotic disease are creating avenues for evaluation of disease progression and therapeutic options. In addition, new developments in gene therapy may provide an option that could augment conventional medical and interventional methods by providing local genetic transfection to reverse atherosclerosis. As has been shown, CBiMRI can be implemented with conventional MRI systems (without the need for the open-MRI magnets), allowing for widespread use. Increased research, technology development, and clinical application can be expected in the future. More research into safety issues will help to ease the technology into clinical application.
Acknowledgments
The author wishes to thank Drs. Joseph M. Aulino, Peter Bream, Thomas Dina, and Theodore Larson for their valued, thoughtful review of the manuscript. Special thanks are extended to Charles Dumoulin for providing a figure, and to Martha Tanner and John Bobbitt for providing assistance with the figures.