The role of positron emission mammography in breast cancer imaging and management
Images
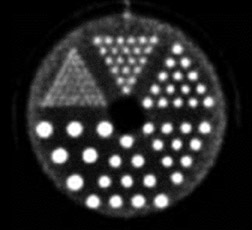
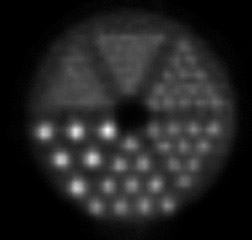
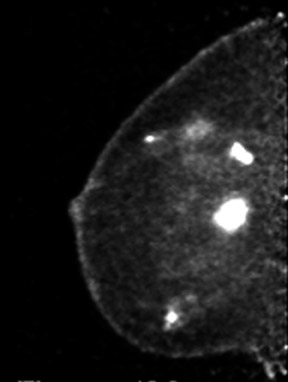
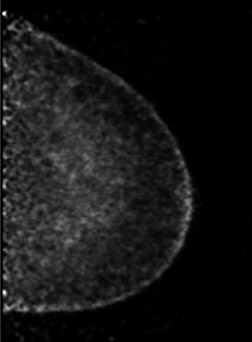
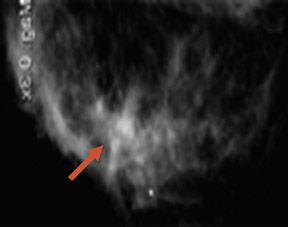
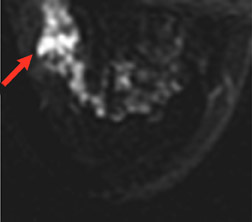
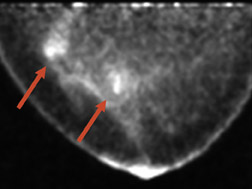
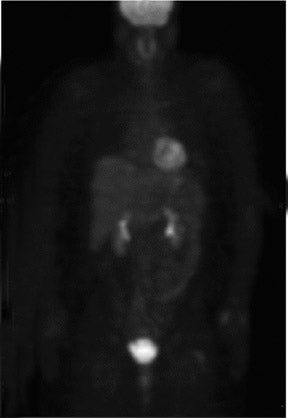
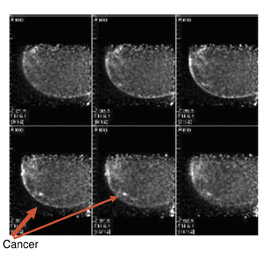
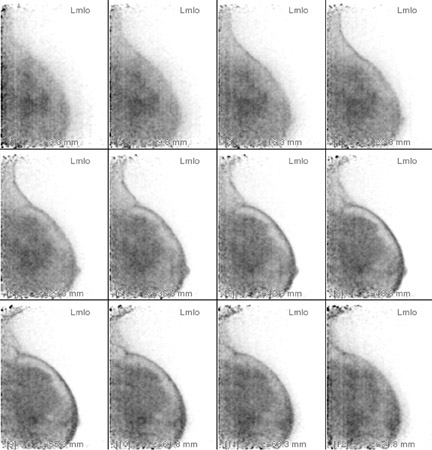
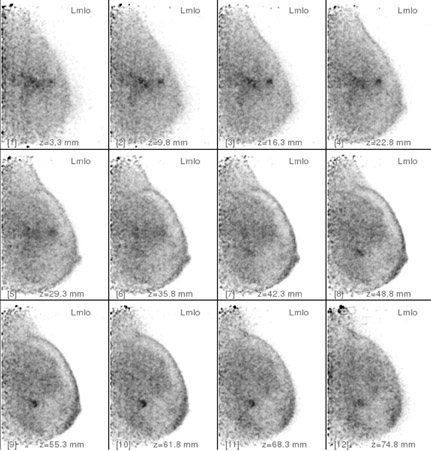
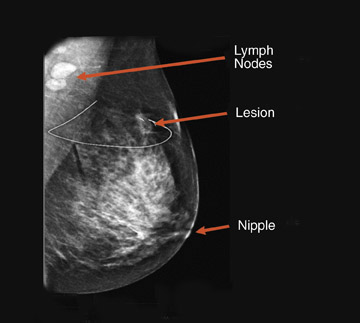
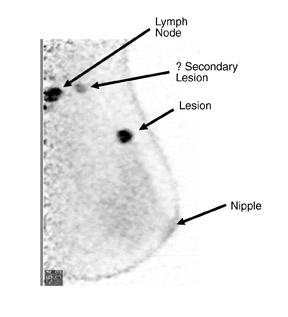
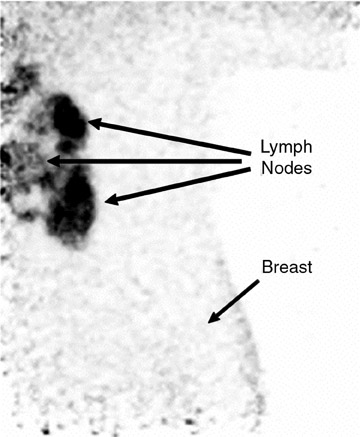
Dr. Schilling is the Medical Director of Imaging & Intervention, Center for Breast Care, Boca Raton Community Hospital, Boca Raton, FL. Dr. Conti is an Associate Professor of Radiology, Division of Nuclear Medicine, and the Clinical Pharmacy and Biomedical Engineering Director, PET Imaging Science Center, Los Angeles, CA. Dr. Adler is the Medical Director, Adler Institute for Advanced Imaging, Jenkintown, PA. Dr. Tafra is the Medical Director, Anne Arundle Medical Center Breast Center, Annapolis, MD. Disclosures: Dr. Schilling and Dr. Tafra are site Principal Investigators on a multicenter trial sponsored by the National Institutes of Health (NIH) and Naviscan PET Systems (San Diego, CA). Dr. Adler has done some consulting work for Naviscan. Dr. Conti is a consultant on the Scientific Advisory Board of Naviscan.
Breast cancer is one of the most frequently diagnosed cancers in women, touching the lives of roughly 213,000 women in 2006.1 In addition to invasive breast cancer, approximately 62,000 cases of in situ breast cancer were projected to occur in 2006, with 85% of these being ductal carcinoma in situ. Even with these increased numbers, mortality is decreasing. Screening mammography has been the gold standard for breast cancer surveillance for 3 decades and is credited with decreasing mortality by 33%.2 Because mammography has resulted in earlier detection of breast cancer, more patients today are candidates for, and choose, breast-conserving surgery instead of mastectomy. However, mammography is frequently inadequate as a planning tool for lumpectomy. In addition, it has very limited value in women with dense breasts. This may explain the finding that residual cancer can be found in as many as 30% to 60% of patients after lumpectomy, resulting in a second trip to the operating room.3
One goal of molecular imaging is to preoperatively identify which patients are best served by lumpectomy and define the margins for surgery by identifying metabolic abnormalities in tissue, potentially decreasing the number of second surgeries needed for resection of residual disease. Any technology that could more precisely map the extent of both invasive and noninvasive disease would lead to more precise surgery. This article will review the history of molecular imaging in breast cancer with a special emphasis on the use of the new technology positron emission mamography (PEM), in pre- and postoperative breast cancer management.
Scintimammography
Nuclear imaging of breast cancer is not a new approach. Thallium 201 (Tl-201) was first shown to be an effective breast tumor-avid agent in 1978.4 Waxman et al5 later compared Tl-201 with technetium (Tc)-99m sestamibi in 1993 and reported that sestamibi had a higher sensitivity in detecting breast cancer. In 1994, Kao et al6 used Tc-99m sestamibi to examine palpable breast masses. Of the 32 cases of breast cancer found, 27 were detected by Tc-99m sestamibi, with a sensitivity of 84%, a specificity of 100%, and an accuracy of 87%.6 This was a small but promising result that prompted 2 large multicenter studies to evaluate scintimammography in more than 2500 patients. These studies found a sensitivity and specificity of scintimammography in the detection of malignant breast tumors of approximately 85%.7,8 Further analysis revealed that sensitivity fell significantly with decreasing tumor size. This was further investigated by Tofani et al,9 who found that scintimammography had a sensitivity of only 48% for tumors ≤1 cm in size. It is important to note that all of these initial studies were completed using conventional whole-body (WB) gamma cameras; during this scanning, the patient is recumbent on an imaging table.
More recently, it was thought that the sensitivity and specificity of sestamibi imaging could be increased with the use of a small field-of-view (SFOV) gamma camera in which the detector is in close proximity to breast tissue. Recent results using this type of camera were reported by the Mayo clinic group.10 In this 100-patient study, they found a detection sensitivity of 29% for tumors <5 mm, 86% for tumors between 6 and 10 mm, and 97% for tumors >11 mm in diameter. The investigators thought that the technique may be an important adjunct to mammography and that greater sensitivity might be achieved with the optimization of collimation and the addition of a second detector. This evolution of technology from WB imaging to SFOV or organ-specific imaging in scintimammography was also seen with positron emission tomography (PET), as described in the next section. The characteristics of WB versus SFOV cameras for scintimammography and PET are compared in Table 1.
Whole-body PET in breast cancer imaging
Whole-body PET and PEM use the radiopharmaceutical 2-deoxy-2-[18F] fluoro-D-glucose (FDG), a positron-emitting analog of glucose, to detect metabolic alterations within cells. This radiotracer takes advantage of the fact that malignant cells express higher levels of the glucose transport protein GLUT-1 and have increased glucose needs. Since 18F-FDG is taken up like glucose but is not metabolized, it becomes metabolically trapped within the cancer cell. The first study to use WB PET imaging for breast cancer was published in 1989.11 This study evaluated 17 patients with metastatic disease who had primary tumors >5 cm in size. They reported that 14 (82%) of the tumors were detected with the 18F-FDG. The positive results of this study prompted Wahl et al12 in 1991 to evaluate the efficacy of WB PET imaging for less advanced breast cancer. They reported that FDG PET identified 10 of 10 primary breast cancers >3 cm. Although the use of PET has been shown to be sensitive and effective for the detection of advanced breast cancer and distant metastatic disease in numerous studies,13,14 it is known to have limitations in detecting small, well-differentiated tumors and in-situ lesions.15 Summarizing a number of studies, the detection rate of WB PET in primary breast cancer has a sensitivity range of 80% to 100% and a specificity range of 75% to 100%, with an accuracy of 70% to 97%, a positive predictive value (PPV) of 81% to 100%, and a negative predictive value (NPV) of 52% to 89%.12,16-21 As would be expected from prior study results, the reports of the highest sensitivities for breast cancer detection have been studies that included patients with large tumors.
A recent study by Mavi et al22 examined the use of dual–time-point imaging as a way to increase sensitivity of WB PET in primary breast cancer detection. In general, this approach involves measuring the FDG uptake in a lesion at 2 separate times, such as 60 and 120 minutes after tracer injection. Cancer cells typically show continued FDG accumulation over this time interval, while normal or nonmalignant cells typically do not. Mavi et al22 reported detection sensitivities of 90.1% for lesions >10 mm (excluding 2 indeterminate cases), 82.7% for lesions 4 to 10 mm (excluding 1 indeterminate case), and 76.9% for ductal carcinoma in situ (DCIS) (excluding 4 indeterminate cases). Thus, although the authors concluded that dual–time-point imaging can improve the sensitivity and accuracy of 18F-FDG in assessing patients with primary breast cancer, there still appears to be room for improvement. Finally, this approach does not address the need for greater accuracy in presurgical planning.
PEM in breast cancer imaging
High-resolution PEM is a new technology that is designed for the imaging of specific small body parts where high-resolution detection of FDG uptake is needed. An available dual-detector system (Naviscan PET Systems, Inc., San Diego, CA) consists of 2 flat, high-resolution detector heads mounted directly to compression paddles that can be rotated to optimize imaging, such as in acquiring mediolateral oblique and craniocaudal breast views. By lightly compressing the breast tissue during acquisition, the image can be acquired in positions that are analogous to those used in mammography, which allows for image coregistration and comparison. The close proximity of the 13mm crystal detectors and limited angle tomographic reconstruction results in an in-plane spatial resolution of 1.5 mm full width at half maximum, compared with the 4.2 to 6.5-mm axial resolution found in commercially available WB PET scanners. The difference in image resolution between WB PET imaging and PEM can be seen in Figure 1. These images were acquired using a phantom filled with ~100 uCi 18F-FDG imaged on the high-resolution PEM system (standard 10-minute acquisition) and a WB PET scanner using a “Brain-mode” 356 × 356 field of view matrix, 10-minute acquisition (Biograph PET scanner, Siemens Medical Solutions USA, Inc., Malven, PA). It is important to remember that the spatial resolution in normal breast tissue in the WB PET scanner would be negatively impacted by recumbent imaging because of normal respiratory motion that was not replicated in this phantom WB PET acquisition. Respiratory motion is not an issue with PEM imaging, as the breast is immobilized (Table 1).
The results of the first PEM pilot study in breast cancer, which used a 10-mm crystal, were published in 2005.23 Of the 44 women with confirmed breast cancers, 39 of the 44 primary index tumors were seen. In addition, of the 19 patients who were undergoing breast-conserving surgery, PEM correctly predicted 75% of patients with positive margins and 100% with negative margins. Figure 2 provides an example of a PEM image that highlights its utility in detecting multicentric disease. Positron emission tomography also detected 4 of 5 histologically proven incidental breast cancers, 3 of which were not seen by any other imaging modality. One such example case is shown in Figure 3. The authors concluded that PEM showed promise in detecting breast malignancies and assisted in planning breast-conserving surgery.
The results of a second, larger multi-center study that examined the performance efficacy of the PEM in women with known breast cancer or suspicious mammography findings were published in 2006.24 In nondiabetic patients with proven breast cancer, PEM was found to have a cancer detection sensitivity of 91%, specificity of 93%, NPV of 88%, and an accuracy of 92%. Most importantly, PEM accurately identified 91% of the cases of DCIS preoperatively. In this study, 36 of 73 biopsies (49%) prompted by conventional imaging alone proved to be benign; however, combining conventional imaging with PEM resulted in few false positives, with a PPV of 95%. This finding highlights the advantage of combining anatomic and metabolic characterization in cancer detection.
The advantage of highly sensitive metabolic imaging is further highlighted in a patient in whom DCIS was seen only with the PEM imaging but missed with magnetic resonance imaging (MRI) and WB PET (Figure 4). This patient was part of a PEM, MRI, WB PET trial being undertaken by Schilling.25 Preliminary findings of this ongoing trial were presented at the Society of Nuclear Medicine meeting in June 200725 and are summarized in Table 2. In addition to the benign findings listed, pathologic analysis identified 39 distinct cancerous or in situ cancerous lesions in the 28 study subjects. With a sensitivity of 92.3%, positron emission mammography had the greatest sensitivity, while WB PET had a sensitivity of only 39%. At the current interim analysis of this small study, the results suggest that PEM is superior to WB PET and is at least as sensitive as MRI in identifying malignant breast disease. In addition, the author has found that the metabolic imaging provided by PEM is helpful in premenopausal women being evaluated for breast cancer because the lesion-to-back-ground FDG uptake ratio does not appear to be affected by hormonal changes in the menstrual cycle. Like WB PET, however, FDG is not recommended for use in pregnant women.
In an attempt to improve the detection sensitivity and specificity of PEM further, Adler et al26 investigated the application of dual–time-point imaging using PEM in a pilot study of 11 patients. They found a median increase in lesion-to-background ratio of 36% (range 16% to 85%). This improved ratio appeared to be due to a reduction in mean background FDG levels (Figure 5). Of interest was the finding that 3 of 3 benign lesions showed a decrease in lesion-to-background ratio at the second measurement. These results are promising and suggest that delayed image comparison may prove helpful in discriminating benign from malignant lesions. Additional, large-scale studies will be needed to test this hypothesis.
PEM and WB PET/CT in surgical planning and breast cancer recurrence
An ideal goal for any molecular imaging approach would be to provide a map of the extent of both invasive and noninvasive disease to assist the surgeon in undertaking more precise excision of involved breast tissue and to more accurately monitor for recurrence. An advantage of the PEM technology is that it uses mammographic positioning, which allows for direct correlation of PEM images with mammography for both initial and recurrence imaging (Figure 6). Positron emission mammography can also provide a tomographic image that may further assist the surgeon in determining the ideal approach to ensure negative margins. Another molecular imaging goal would be to provide assistance in determining the extent of disease (eg, lymph node involvement). While it is too early to determine the sensitivity and specificity of the PEM technology for this goal, some commercial sites are obtaining useful images of lymph node involvement (Figure 7).
Approximately 16% to 30% of patients with local-regional recurrence have been found to have metastatic disease when evaluated with WB27-30 In addition, 24% of patients with breast cancer recurrence locally will develop a site of distant disease within 18 months.29 Although this highlights the importance of WB PET in the detection of metastatic disease, an important question remains: whether the use of PEM imaging for routine surveillance in women treated for breast cancer might allow detection of local-regional recurrence at an earlier stage. The corollary is, if detected earlier, could earlier therapy prevent or decrease the incidence of cancer metastasis?
Whole-body PET has been used to predict response to neoadjuvant chemotherapy in women with advanced breast cancer. It has been shown to have a sensitivity range of 80% to 90% and a specificity range of 50% to 80% in predicting pathological response (eg, the decrease in FDG uptake).31,32 The wide range of values reflects the differences in the definition of response among different centers as well as the diminished sensitivity of WB PET in smaller lesions. Although it appears that PET can frequently determine an early response before other forms of imaging,33 the question of whether PEM imaging will offer an even greater advantage in the management of neoadjuvant chemotherapy patients will need to await the results of a clinical trial.
New molecular imaging agents for PEM
Although the introduction of 18F-FDG in PET imaging has changed patient management in a variety of cancers (including breast), the ultimate goal in molecular imaging is to image the in vivo cancer biology of an individual to allow therapy to be personalized. The introduction of new positron-emitting imaging agents such as the cell proliferation markers [F-18]-fluoro-L-thymidine (FLT)34 and F-18 or C-11-2'-fluoro-5-methyl-1-beta-d-arabinofuranosyluracil (FMAU),35 and [F-18]-fluoromisonidazole,36 a radiotracer marker for tumor hypoxia, offers new opportunities for evaluating breast cancer and might help achieve this goal. In addition, 16a-[F-18]-fluoroestradiol-17b (FES)37 ap-pears to be a promising estradiol analog and provides an imaging approach to monitor and predict clinical response to hormonal therapy in vivo. Positron emission mammographic technology should be equally effective at imaging the localization of these radiotracers, as it has been for 18F-FDG. The current data would suggest that positron radiotracer development and PET/PEM imaging technologies are in their infancy; however, combined, they are bringing us closer to personalized cancer therapy.
Acknowledgment
The authors would like to thank Ms. Mindy McKinstry of EPIC Imaging, Portland, OR, for the axillary lymph node imaging and Judith E. Kalinyak, MD, PhD, Medical Director, Naviscan PET Systems, Inc., for providing assistance in preparing this manuscript.
REFERENCES
- Cancer Facts and Figures 2006. Atlanta, GA: American Cancer Society; 2006.
- Mammography Accounting Office. GAO/HEHS-96-17. Initial impact of new federal law has been positive. Report to Congressional Committees, Washington, DC; U.S. Government Accounting Office; October 27, 1995.
- Gwin JL, Eisenberg BL, Hoffman JP, et al. Incidence of gross and microscopic carcinoma in specimens from patients with breast cancer after re-excision lumpectomy. Ann Surg. 1993;218:729-734.
- Hisadi K, Tonami N, Miyamae T, et al. Clinical evaluation of tumor imaging with 201 Tl chloride. Radiology. 1978;129:497-500.
- Waxman AD, Ramanna L, Memsic LD, et al. Thallium scintigraphy in the evaluation of mass abnormalities of the breast. J Nucl Med. 1993;34:18-23.
- Kao CH, Wang SJ, and Liu TJ. The use of technetium-99m methoxyisobutylisonitrile breast scintigraphy to evaluate palpable breast masses. Eur J Nucl Med. 1994;21:432-436.
- Taillefer R. The role of 99mTc sestamibi and other conventional radiopharmaceuticals in breast cancer diagnosis. Semin Nucl Med. 1999;29:16-40.
- Waxman AD. The role of (99m)Tc methoxyisobutylisonitrile in imaging breast cancer. Semin Nucl Med. 1997;27(1):40-54.
- Tofani A, Sciuto R, Semprebene A, et al. 99Tcm-MIBI scintimammography in 300 consecutive patients: Factors that may affect accuracy. Nucl Med Commun. 1999;20:1113-1121.
- O’Connor MK, Phillips SW, Hruska CB, et al. Molecular breast imaging: Advantages and limitations of a scintimammographic technique in patients with small breast tumors. Breast J. 2007;13(1):3-11. Comment in: Breast J. 2007;13(1):1-2.
- Minn H, Soini I. [18F]fluorodeoxyglucose scintigraphy in diagnosis and follow up of treatment in advanced breast cancer. Eur J Nucl Med. 1989:15: 61-66.
- Wahl RL, Cody RL, Hutchins GD, Mudgett EE. Primary and metastatic breast carcinoma: Initial clinical evaluation with PET with the radiolabeled glucose analogue 2-[F-18]-fluoro-2-deoxy-D-glucose. Radiology. 1991;179:765-770.
- Schirrmeister H, Kühn T, Guhlmann A, et al. Fluorine-18 2-deoxy-2-fluoro-D-glucose PET in the preoperative staging of breast cancer: Comparison with standard staging procedures. Eur J Nucl Med. 2001;28:351-358.
- Rosé C, Dose J, Avril N. Positron emission tomography for the diagnosis of breast cancer. Nucl Med Commun. 2002;23:613-618.
- Eubank WB, Mankoff DA. Evolving role of positron emission tomography in breast cancer imaging. Semin Nucl Med. 2005;35:84-99.
- Scheidhauer K, Walter C, Seemann MD. FDG PET and other imaging modalities in the primary diagnosis of suspicious breast lesions. Eur J Nucl Med Mol Imaging. 2004;31(suppl 1):S70-S79.
- Adler LP, Crowe JP, al-Kaisi NK, Sunshine JL. Evaluation of breast masses and axillary lymph nodes with [F-18] 2-deoxy-2-fluoro-D-glucose PET. Radiology. 1993;187:743-750.
- Hoh CK, Hawkins RA, Glaspy JA, et al. Cancer detection with whole-body PET using 2-[18F]fluoro-2-deoxy-D-glucose. J Comput Assist Tomogr. 1993;17:582-589.
- Avril N, Dose J, Jänicke F, et al. Metabolic characterization of breast tumors with positron emission tomography using F-18 fluorodeoxyglucose. J Clin Oncol. 1996;14:1848-1857.
- Avril N, Rosé CA, Schelling M, et al. Breast imaging with positron emission tomography and fluorine-18 fluorodeoxyglucose: Use and limitations. J Clin Oncol. 2000;18:3495-3502.
- Scheidhauer K, Schari A, Pietrzyk U, et al. Quantitative [18F]FDG positron emission tomography in primary breast cancer: Clinical relevance and practicability. Eur J Nucl Med. 1996 23:618-623.
- Mavi A, Urhan M, Yu JQ, et al. Dual time point 18F-FDG PET imaging detects breast cancer with high sensitivity and correlates well with histologic subtypes. J Nucl Med. 2006;47:1440-1446.
- Tafra L, Cheng Z, Uddo J, et al. Pilot clinical trial of 18F-fluorodeoxyglucose positron-emission mammography in the surgical management of breast cancer. Am J Surg. 2005;190:628-632.
- Berg WA, Weinberg IN, Narayanan D, et al. High-resolution fluorodeoxyglucose positron emission tomography with compression (“positron emission mammography”) is highly accurate in depicting primary breast cancer. Breast J. 2006;12(4):309-323.
- Schilling KJ. High-resolution positron emission mammography in breast cancer. J Nuc Med. 2007; 48 (suppl 2):139P.
- Adler, LP, Narayanan D, Gammage L, et al. Quantitative improvement in breast lesion detectability on delayed images using high resolution positron emission mammography. J Nuc Med. 2007;48 (suppl 2):369P.
- Danforth DN Jr, Aloj L, Carrasquillo JA, et al. The role of 18F-FDG-PET in the local/regional evaluation of women with breast cancer. Breast Cancer Res Treat. 2002;75(2):135-146.
- Goerres GW, Michel SC, Fehr MK, et al. Follow-up of women with breast cancer: Comparison between MRI and FDG PET. Eur Radiol. 2003;13: 1635-1644.
- van Oost FJ, van der Hoeven JJ, Hoeckstra OS, et al. Staging in patients with locoregionally recurrent breast cancer: Current practice and prospects for positron emission tomography. Eur J Cancer. 2004; 40:1545-1553.
- Weir L, Worsley D, Bernstein V. The values of FDG positron emission tomography in the management of patients with breast cancer. Breast J. 2005; 11:204-209.
- Kim SJ, Kim SK, Lee ES, et al. Predictive value of [18F]FDG PET for pathological response of breast cancer to neo-adjuvant chemotherapy. Ann Oncol. 2004;15:1352-1357.
- Smith IC, Welch AE, Hutcheon AW, et al. Positron emission tomography using [(18)F]-fluorodeoxy-D-glucose to predict the pathologic response of breast cancer to primary chemotherapy. J Clin Oncol. 2000;18:1676-1688.
- Chen X, Moore MO, Lehman CD, et al. Combined use of MRI and PET to monitor response and assess residual disease for locally advanced breast cancer treated with neoadjuvant chemotherapy. Acad Radiol. 2004;11:1115-1124.
- Shields AF. Positron emission tomography measurement of tumor metabolism and growth: Its expanding role in oncology. Mol Imaging Biol. 2006; 8(3):141-150.
- Conti PS, Alauddin MM, Fissekis JR, et al. Synthesis of 2'-fluoro-5-[11C]-methyl-1-beta-D-arabinofuranosyluracil ([11C]-FMAU): A potential nucleoside analogue for in vivo study of cellular proliferation with PET. Nucl Med Biol. 1995;22:783-789.
- Alauddin MM, Conti PS, Fissekis JD. Synthesis of [18F]-labeled 2'-deoxy-2'-fluoro-5-methyl-1-beta-D-arabinofuranosyluracil. J Label Compd Radiopharm. 2002;45:583-590.
- Kumar P, Mercer J, Doerkson C, et al. Clinical production, stability studies and PET imaging with 16-alpha-[18F]fluoroestradiol ([18F]FES) in ER positive breast cancer patients. J Pharm Pharm Sci. 2007;10:256S-265S.