Prostate MRI: Update and current roles
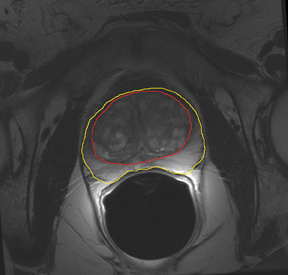
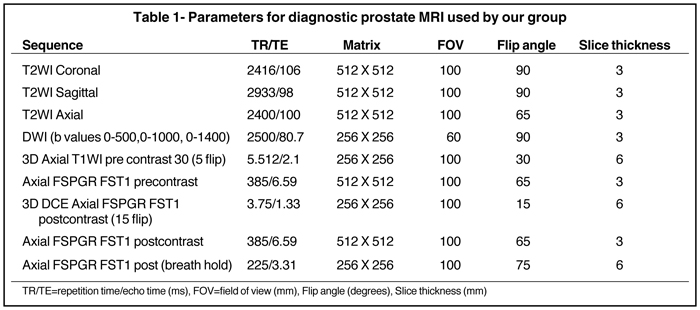
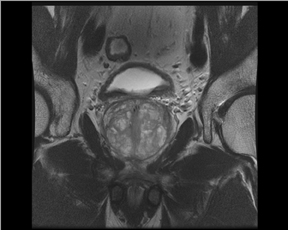
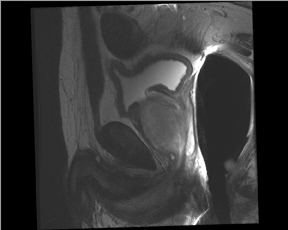
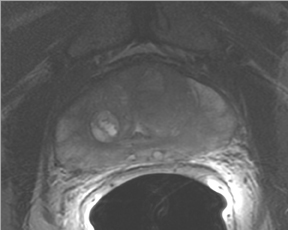
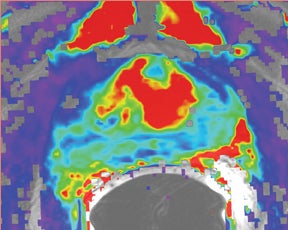
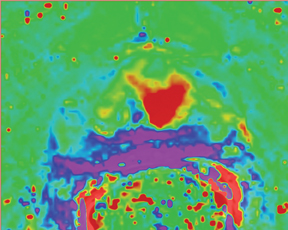
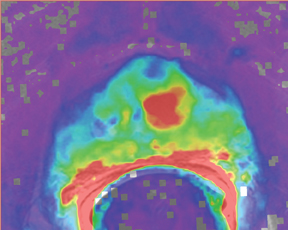
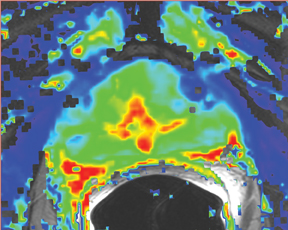
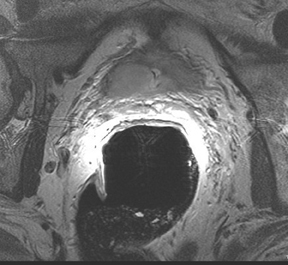
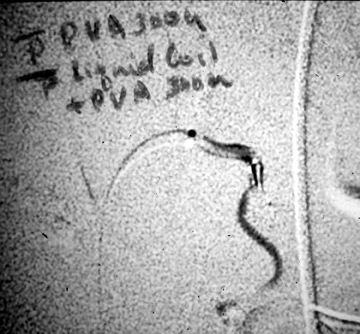
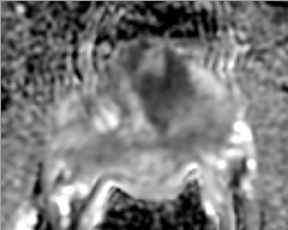
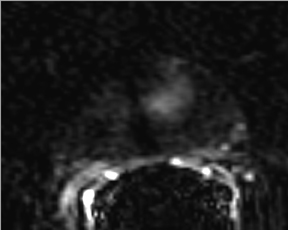
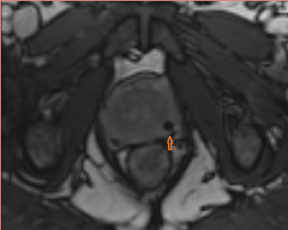
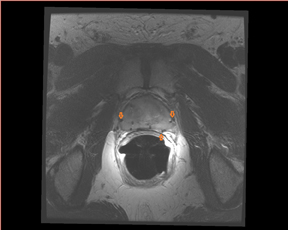
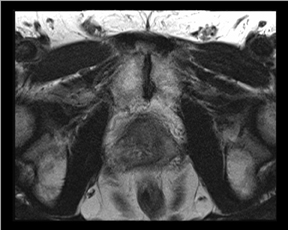
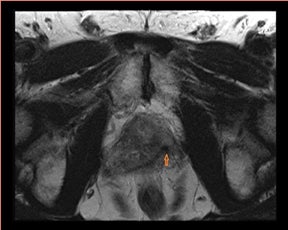
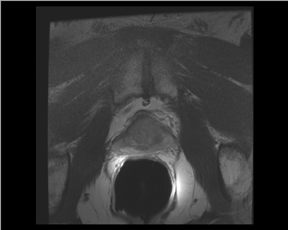
Dr. Tempany is the Ferenc Jolesz Distinguished Chair of Radiology Research, Professor of Radiology Harvard Medical School, Department of Radiology, Brigham and Women’s Hospital, Boston, MA; and Mr. Franco is a Medical Student from the University of Sao Paulo, Sao Paulo Brazil.
Prostate diseases affect millions of men every year around the world. Congenital malformations, prostatitis (acute, chronic bacterial and chronic abacterial), benign prostatic hyperplasia (BPH), and prostate cancer comprise most of the abnormalities in the gland. However, special attention is given to prostate cancer, as it is a leading cause of morbidity and mortality among men, especially in western countries.
Within the United States, the number of newly diagnosed prostate cancer patients in 2010 was estimated to be 217,730, and the number of deaths estimated to be 32,050.1 As with other forms of cancer, prostate cancer is most effectively treated when diagnosed early in its progression. Improved diagnostic methods, therefore, are critical to effective treatment and better patient outcome.2
Today the detection of prostate cancer begins with prostate-specific-antigen (PSA) levels and/or digital rectal examination (DRE). If either of these are abnormal, a transrectal ultrasound (TRUS)-guided biopsy is often the next step. Thus, a prostate cancer diagnosis is typically made through TRUS-guided sextant biopsy and histopathological examination. The false negative rate of TRUS-guided biopsies is estimated to be between 15% and 34%. Problems arise when, despite a high degree of suspicion for cancer (based uponPSA/DRE), a pathological diagnosis cannot be confirmed. In such patients, magnetic resonance imaging (MRI)can help in one of 3 ways that are discussed below.MRI is accepted as the best imaging modality for displaying anatomical details of the prostate. MRI has typically been incorporated as a staging tool after a diagnosis is made through a transrectal biopsy.3 Recently, the authors and others have described a new role for MRI involving detection of suspicious foci and MRI-guided biopsy of these areas. This article reviews the current impact of MRI on choice of therapy and treatment planning.
The selection of therapy is especially important when there is a risk for stage-T3 disease with extraglandular extension (EGE)4 In these cases,MR imaging with an endorectal coil can achieve positive predictive values between 85% and 97% for extracapsular extension (ECE) and seminal vesicle invasion (SVI), respectively.5,6 Not surprisingly, it is more accurate in localizing tumors than either TRUS-guided biopsy or digital rectal examination. New multiparametric MRI (Mp-MRI) at 3.0 tesla (T) using the endorectal coil offers a much improved examination to detect a tumor, localize it accurately, and characterize the tissue to correlate with the Gleason biopsy score. With the introduction of new treatment strategies, such as cryotherapy, brachytherapy, and focused ultrasound, more precise information regarding tumor extent is needed.7-9 MRI offers a new opportunity to patients with negative TRUS-guided biopsies. For example, an MRI evaluation even before the TRUS-guided biopsy could be beneficial, especially for patients at risk for high-grade tumors or tumors in the transitional zone (TZ) or central gland.10 Thus, MRI of the prostate is fulfilling multiple roles in regard to prostate cancer, including improving diagnostic accuracy; enabling risk stratification, initial staging, surveillance of cancer recurrence and treatment response; characterization of prostatic tissue; and, more recently, guidance of focal therapy or biopsy for diagnosis.11
Prostate anatomy
The prostate resembles an inverted cone located right under the bladder, lying anterior to the rectum and posterior to the pubic bone, postero-laterally surrounded by the neurovascular bundle (NVB). The seminal vesicles are located postero-superiorly to the gland and, together with the NVB, make up the preferential paths for tumor spread once the tumor has penetrated the prostatic capsule. The gland is divided into 3 zones: the peripheral (PZ), transition (TZ) and central (CZ) zones.The first constitutes most of the glandular tissue within the organ and is the origin of most tumors of glandular origin (approximately 70%), followed by 20% and 10% in the TZ and CZ, respectively. It is important to point out that the boundaries between theCZ and TZ are difficult to find through imaging; therefore, both are frequently dominated by the central gland (Figures 1 and 2).
For localization purposes, the prostate gland is usually arbitrarily divided into the apex, middle gland, and base. This can define the traditional prostatic sextants used for TRUS-guided biopsy and correlation with histology samples.
Current techniques
Previous reports and experience have established the feasibility, reliability, and ease of prostate MRI at 1.5T that is being rapidly overshadowed by the shift to 3T high-field MRI scanners. Studies have shown that higher-field scanners using T1-weighted (T1W) and T2-weighted(T2W) sequences allow for better spatial and temporal resolution with a higher signal-to-noise ratio (SNR). The improved SNR has led to the introduction of new sequences, such as diffusion-weighted imaging (DWI) and dynamic contrast-enhanced sequences. Moreover, with shorter acquisition times, such powerful magnets, enable Mp-MRI studies of the prostate to be run in only one session without major complications or patient discomfort.
In addition to the use of 3T magnets, another advance in MRI with respect to prostate cancer is the use of an endorectal coil with a pelvic coil.This approach has the advantage of increasing SNR with fewer artifacts and better image resolution than MRI without the endorectal coil. On the other hand, it creates discomfort for the patient and deforms the gland. However, one recent study indicated that the combination provides higher accuracy; thus, the use of the endorectal and pelvic coils is suggested for most studies.12 It has been said that a prostate exam at 1.5Twith an endorectal coil is equivalent to one at 3.0T without the coil without further improvement or gain from 3.0T. Thus, many groups continue to use the endorectal coil and at the same time maximize the advantages of the higher field strength. If the endorectal coil is used, the patient should have IV/IM administration of buscopolamyne or glucagon to decrease the intestinal peristaltic activity during the examination.
The endorectal coil is lubricated with a topical anesthetic (usually xylocaine or lidocaine), inserted and inflated with 60 ml to 80 ml of either air or liquid per fluorocarbon, which can reduce air associated artifacts (Figure 3). The waiting time after biopsy to perform a scan is controversial. In general, clinicians advocate waiting about 4 weeks after biopsy with some advocating up to 8 weeks to prevent artifacts from hematoma or prostatic inflammation. Interestingly, some studies have pointed out that the artifact may not resolve for months, creating anxiety for the patient during the interval between biopsy and the MRI study waiting for a definitive diagnosis.
MRI sequences for the prostate
T2W MRI is a very well established and essential sequence, compared with other techniques, as it provides an excellent display of the prostate and its substructure anatomy. Focal tumor usually appears as an area of low signal surrounded by the high signal of the normalPZ. It is sometimes challenging to detect carcinoma in the PZ due to several factors that may mimic malignant foci, such as postbiopsy hemorrhage, benign prostatic hypertrophy, scars, fibromuscular tissue, calcifications, prostatitis, and the effects of radiation treatment.Even more challenging may be the detection of neoplastic tissue in the central gland where nodules appear with mixed signal intensities (Figure 4). If there is a homogeneous lenticular shape with low signal on T2W, a central gland focal cancer should be suspected.
Due to its limitations, T2W alone does not achieve adequate sensitivity and specificity for prostate cancer. High signal areas in T1W overlapping with low-signal areas on T2W are likely to produce artifacts due to postbiopsy hemorrhage. To avoid this error and enable higher diagnostic accuracy, Mp-MRI techniques are now widely used. DWI, magnetic resonance spectroscopy imaging (MRSI), dynamic contrast enhancement (DCE) and its postprocessed maps are now part of a state-of-the-art MR imaging set and can increase the detection of significant prostate cancer, markedly improving diagnostic capability. In the DCE series, many images are obtained at different phases after the bolus of gadolinium has circulated through the prostate. The raw data can demonstrate areas of enhancement and can be used to estimate the presence of focal cancer. However, the raw data can be analyzed in various ways, one of which is in a contrast kinetics software program that models blood flow and tissue reaction to produce individual sets of images in color illustrating different pharmo-kinetic parameters. The postprocessed values acquired with these new techniques can be validated quantitatively using color maps that are visually clear and through objective metrics,such as apparent diffusion coefficient (ADC) maps as well as with parameters from 2 compartment pharmacokinetic models, such as ktrans (wash-in), kep (wash-out), maximum slope for wash-in and wash-out, and Ve (extravascular–extracellular volume fraction) (Figure 5). Mp-MRI combined with traditional T2W can be used not only to analyze the presence or absence of prostate carcinoma and to plan therapy but also to characterize the histological features of tumors. One study has shown that Mp-MRI is correlated to tissue composition for tumors and benign tissue,13 and it can allow for differentiation between BPH and prostate cancer in the central gland.14 Mp-MRI can also be used with good accuracy for determining recurrence after local salvage therapy.15 An ideal combination of all these sequences and postprocessed data must still be established, but it will certainly rely on the combination of several different sequences to reach the highest levels of accuracy in cancer detection and characterization.16
DWI MRI
First described to assess stroke and ischemia in the brain, diffusion-weighted imaging (DWI) measures the water diffusion within tissue. It is well known that neoplasia, due to its local neoangiogenesis, usually affects the diffusion capacity of water molecules; therefore, this technique can be used in prostate imaging, allowing for short acquisition times and no need for IV contrast medium administration.17 DWI sequences are acquired using a range of b values (500, 1000, and 1400) to generate ADC maps. More recently the higher b values—over 1000 —have shown great promise for the detection and characterization of focal tumors. Tumors show a lower ADC value than benign regions, both in PZ and the central gland (Figure 6). One study has shown that the lower the ADC value, the higher the Gleason score and the more aggressive the tumor is—with higher clinical risk—for those tumors in the PZ.18
The addition of an ADC map to T2W images can improve the diagnostic performance of MR imaging in prostate cancer detection,16 helping to distinguish malignant from benign tissues. Accounting for a finite water exchange rate between cells and their environment may also aid staging accuracy and the ability to monitor response to treatment.19 The combination of ADC and T2W can be used to differentiate cell density both in cancerous and noncancerous tissue and, therefore it plays an important role in the estimation of the Gleason score at 3T.20
DCE and pharmacokinetic models—applied color maps
DCE (dynamic contrast-enhanced) imaging was introduced to effectively visualize the pharmacokinetics of gadolinium uptake in tissue as tumors’ angiogenesis differs from that of benign tissue. DCE imaging acquires data on tissue perfusion characteristics and tumor wash-in and washout contrast that are variables which rely on the pathophysiologic principle that tumors display increased angiogenesis, and, thus, are expected to show early and increased enhancement.
DCE imaging for prostate is evaluated by means of the direct raw interpretation of T1W images played in cine mode (Figure 6) and from color maps generated from 2 compartment pharmacokinetic models. The following general kinetic models are usually selected for processing: ktrans, kep, maximum slope for wash-in and wash-out, and Ve (extravascular–extracellular volume fraction) (Figure 5). The mean peak values of ktrans (forward value transfer constant), kep (reverse reflux rate constant between extracellular space and plasma), time to peak (TTP) and maximum slope (MaxS) are currently the parameters of most interest. These processed data can be analyzed either visually (by generating color maps) or quantitatively through straight values.
Quantitative measurements reflect some exciting results and can play an even greater role in the future of prostate care. Prostate MRI methods may one day substitute for some of the in vivo assessments done today by histology or, perhaps more importantly, guide focal therapy and enable the careful monitoring of therapy after delivery. Though the difference in impact is only significant for evaluating the PZ, quantitative dynamicMRI is more accurate than T2W imaging for tumor localization of nonpalpable cancer greater than 0.2 cm3. Above this volume, correlation between tumor volume measured on dynamic MRI and that on the specimen is poor.14, 21 With that said, DCE imaging is a proven method to help localize tumors within the prostate.
Spectroscopy
MR spectroscopy is another technique that allows one to noninvasively assess metabolites present in biological tissue. Given that the use of an MRI body coil alone does not reach sufficient resolution, clinical practice improved once the endorectal coil was introduced, as it provided3D MRSI of the prostate with increased sensitivity. Currently, the resolution of MRSI with 1.5T scanners is a voxel size of approximately 0.3 cm,3 while for 3T, voxels smaller than 0.2 cm3 are feasible. The levels of citrate, choline, and creatine are useful for the evaluation of prostate cancer, as it is known that tumors have an elevated level of choline and a decreased level of citrate. Though it is important to point out that the graphical analysis of creatine and choline is usually not separable, the ratio (choline + creatine/citrate) can be used for the prediction of malignancy. Several studies have tried to suggest levels of these substances as predictors for prostate cancer. In a recent study, Kumar et al showed a good prediction for tumor detection when a cutoff of 1.2 in (citrate/choline+creatine) ratio was used to assess the likelihood of malignancy in the PZ. However, there is still no agreement among studies about concentrations of metabolites in diagnosing cancer. This lack of consensus is probably due to differences in technique for data acquisition and interpretation; indeed, no standard has been reached. Studies have shown specificities of 49% to 88% with accompanying sensitivities of 63% to 98%, respectively, for MRSI. Unfortunately, most of these good results excluded analysis of the central gland.4 Due to signal overlapping from the PZ, MRSI still does not obtain highly accurate results in the innerregions of the prostate. Moreover, a recent multi-institutional prospective study demonstrated that MRSI combined with MRI achieves the same levels of accuracy for detecting tumors in the PZ compared to MRI alone.22
Current suggested protocol
Current state-of-the-art MRI techniques for prostate care are performed on 3T magnets and are based on the following sequences: T2W fast spin echo in 3 orthogonal orientations; axial unenhanced T1W; and dynamic axial 3-dimensional fast spoiled gradient echo T1W for 90sec after injection of contrast. These data are then processed by specialized software to yield color maps for the DCE series and ADC maps from generated raw DWI. This protocol has been satisfactorily used for the last 5 years for prostate cancer detection, staging, and assessment of radiation therapy at the Brigham and Women’s Hospital in Boston. Moreover, the protocol has been endorsed by 16 European experts at a recent meeting.23
Staging, localization, and treatment planning
Overall staging is based on whether tumor is organ confined (T1/T2) or beyond the prostatic tissue (T3/T4). SVI (seminal vesicle invasion)and extracapsular extension (ECE) are important factors for staging disease (Figure 7). For SVI, the accepted criteria are low signal intensity in 1 or both SV (usually high signal in T2W) or disruption or loss of the normal architecture in the ducts or the glands, whereas the criteria forECE are tumor extension into the periprostatic fat tissue, focal capsular bulge, irregularity, retraction, and rectoprostatic angle obliteration.24 ECE initially occurs most commonly at the 5 and 7 o’clock locations in the axial plane. Wang et al17 showed that MRI has better accuracy for predicting SVI than clinical variables. Moreover, the endorectal MRI has shown the most promising results in detecting SVI with specificity up to 99% and sensitivity up to 80%. For assessing ECE, MRI (T2W) has a reported accuracy of between 50% and 90%.24 The typical workflow for the staging of prostate cancer by MRI is shown in Table 1.
Even though MRI techniques, such as DCE and MRS, cannot detect precancerous lesions, such as prostatic intraepithelial neoplasia, MRI is useful for predicting a tumor’s grade. Treatment choice, including active surveillance, relies on MRI to assist in the prediction of a tumor’s behavior; one important aspect of this is the tumor size and prostate volume. For example, MRI is known to be better than TRUS for assessing prostate volume, which is very important to know for radiation therapy to optimize outcomes. For instance, patients must be defined as having a prostate smaller than 60 cc for them to be deemed suitable for external beam radiation therapy or brachytherapy.
The criteria for staging prostate carcinoma not only involves defining ECE and SVI but it also should concomitantly evaluate pelvic lymph nodes and osseous structures to detect all sites of possible metastases in a single examination.11
Conclusion
Based upon the published literature, we believe that MRI will become a more requested exam for prostate evaluation and will play a more central role in the diagnosis of prostate cancer. It could significantly reduce the degree of diagnostic uncertainty that now plagues many clinicians and their patients, provide an excellent “biomarker” for men undergoing active surveillance for nonsignificant disease, and allow for improved selection of treatment for those with significant tumors. Current research includes the development of new MRI sequences for 3Tmagnets that offer the undervalued benefit of decreasing patient examination time.
Moreover, research by several groups using interventional techniques (such as biopsy) under MRI guidance (Figure 8) show improved diagnostic accuracy and allow for novel treatment options, such as targeted therapy with focused ultrasound, cryotherapy, or laser.25 Anatomical detail, only depicted by MRI, ensures the feasibility of focal treatments, such as external beam radiation, seed placement during brachytherapy, and focal ablation by high-intensity focused ultrasound (HIFU) (Figure 9). Moreover, software, such as the open source 3D Slicer (www. slicer.org) with a prostate module called ProstateNav, provides image registration methods and offers guidance to the interventional radiologist,showing details, such as prostate movement and intraprocedure deformation, enabling accurate biopsy needle placement (Figure 10).26
Acknowledgments: The authors thank Kimberly Lawson for help in editing, preparing, and overseeing this work, and Darcell McKenzie for administrative assistance.
References
- National Cancer Institute. Prosate cancer. http://www.cancer.gov/cancertopics/types/prostate. Accessed February 7, 2011.
- McLeod DG. Success and failure of single-modality treatment for early prostate cancer. Rev Urol. 2004;6 Suppl 2:S13-19.
- Thompson I, Thrasher JB, Aus G, et al. Guideline for the management of clinically localized prostate cancer: 2007 update. J Urol. 2007; 177:2106-2131.
- Umbehr M, Bachmann LM, Held U, et al. Combined magnetic resonance imaging and magnetic resonance spectroscopy imaging in the diagnosis of prostate cancer: A systematic review and meta-analysis. Eur Urol. 2009;55:575-590.
- Ogura K, Maekawa S, Okubo K, et al. Dynamic endorectal magnetic resonance imaging for local staging and detection of neurovascular bundle involvement of prostate cancer: Correlation with histopathologic results. Urology. 2001;57:721-726.
- Wang L, Hricak H, Kattan MW, et al. Prediction of organ-confined prostate cancer: Incremental value of MR imaging and MR spectroscopic imaging to staging nomograms. Radiology. 2006;238:597-603.
- Haker SJ, Mulkern RV, Roebuck JR, et al. Magnetic resonance-guided prostate interventions. Top Magn Reson Imaging. 2005; 16:355-368.
- Jolesz FA, Hynynen K, McDannold N, Tempany C. MR imaging-controlled focused ultrasound ablation: A noninvasive image-guided surgery. Magn Reson Imaging Clin N Am. 2005;13:545-560.
- Akduman B, Barqawi AB, Crawford ED. Minimally invasive surgery in prostate cancer: Current and future perspectives. Cancer. 2005;11:355-361.
- Ahmed HU, Kirkham A, Arya M, et al. Is it time to consider a role for MRI before prostate biopsy? Nat Rev Clin Oncol. 2009;6:197-206.
- Kelloff GJ, Choyke P, Coffey DS. Challenges in clinical prostate cancer: Role of imaging. AJR Am J Roentgenol. 2009; 192:1455-1470.
- Heijmink SW, Futterer JJ, Hambrock T, et al. Prostate cancer: Body-array versus endorectal coil MR imaging at 3T--comparison of image quality, localization, and staging performance. Radiology. 2007;244:184-195.
- Langer DL, van der Kwast TH, Evans AJ, et al. Prostate tissue composition and MR measurements: Investigating the relationships between ADC, T2, K(trans), v(e), and corresponding histologic features. Radiology. 2010;255:485-494.
- Oto A, Kayhan A, Jiang Y, et al. Prostate tissue composition and MR measurements: Investigating the relationships between ADC, T2, K(trans) MR imaging. Radiology. 2010;257:715-723.
- Arumainayagam N, Kumaar S, Ahmed HU, et al. Accuracy of multiparametric magnetic resonance imaging in detecting recurrent prostate cancer after radiotherapy. BJU Int. 2010;106:991-997.
- Turkbey B, Pinto PA, Mani H, et al. Prostate cancer: Value of multiparametric MR imaging at 3T for detection—histopathologic correlation. Radiology. 2010;255:89-99.
- Wang L, Hricak H, Kattan MW, et al. Prediction of seminal vesicle invasion in prostate cancer: Incremental value of adding endorectal MR imaging to the Kattan nomogram. Radiology. 2007;242:182-188.
- Turkbey B, Shah VP, Pang Y, et al. Is apparent diffusion coefficient associated with clinical risk scores for prostate cancers that are visible on 3-T MR images? Radiology. 2011;258:488-495.
- Lowry M, Zelhof B, Liney GP, et al. Analysis of prostate DCE-MRI: Comparison of fast exchange limit and fast exchange regimen pharmacokinetic models in the discrimination of malignant from normal tissue. Invest Radiol. 2009;44:577-584.
- Gibbs P, Liney GP, Pickles MD, et al. Correlation of ADC and T2 measurements with cell density in prostate cancer at 3.0 Tesla. Invest Radiol. 2009;44:572-576.
- Mullerad M, Hricak H, Kuroiwa K, et al. Comparison of endorectal magnetic resonance imaging, guided prostate biopsy and digital rectal examination in the preoperative anatomical localization of prostate cancer. J Urol. 2005;174:2158-2163.
- Weinreb JC, Blume JD, Coakley FV, et al. Prostate cancer: Sextant localization at MR imaging and MR spectroscopic imaging before prostatectomy--results of ACRIN prospective multi-institutional clinicopathologic study. Radiology. 2009; 251:122-133.
- Dickinson L, Ahmed HU, Allen C, et al. Magnetic resonance imaging for the detection, localisation, and characterisation of prostate cancer: Recommendations from a European consensus meeting. Eur Urol. 2011;59:477-494.
- Futterer JJ. MR imaging in local staging of prostate cancer. Eur J Radiol. 2007;63:328-334.
- Tempany C, Straus S, Hata N, Haker S. MR-guided prostate interventions. J Magn Reson Imaging. 2008;27:356-367.
- Tokuda J, Fischer GS, Csoma C, et al. Software strategy for robotic transperineal prostate therapy in closed-bore MRI. Med Image Comput Comput Assist Interv. 2008;11:701-709.