Perfusion CT in the assessment of cerebrovascular disease
Images
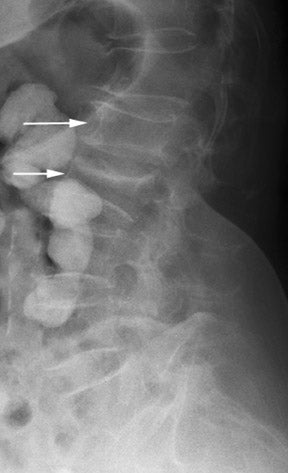
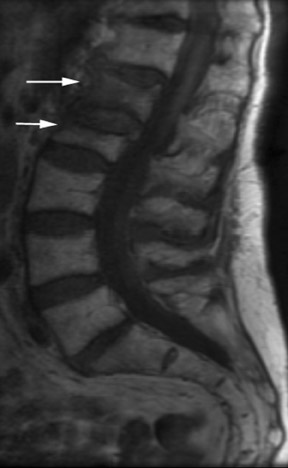
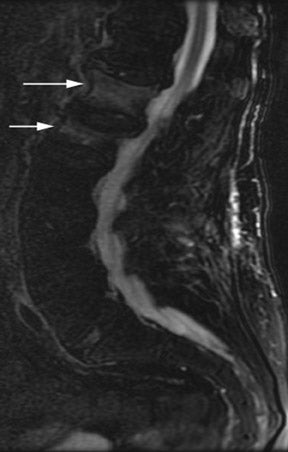
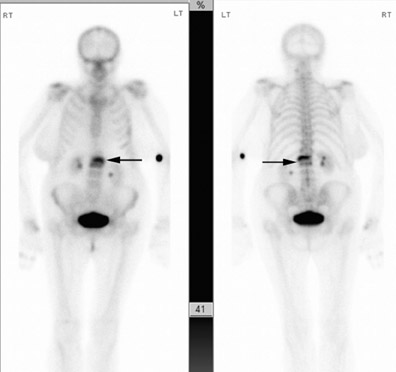
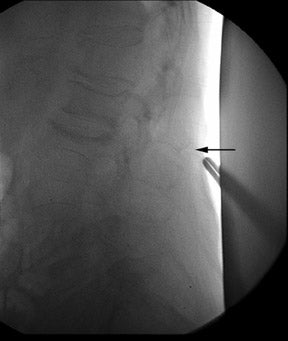
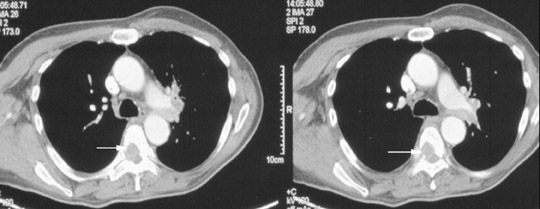
Dr. Kim is Chief Resident and Dr. Ortiz is Chairman of the Department of Radiology, Winthrop-University Hospital, Mineola, NY.
Radiologic evaluation of patients with cerebrovascular disease has evolved with the introduction of new technology. Specifically, multislice computed tomography (MSCT) has emerged as an imaging modality that can assess the extent and severity of cerebral ischemia. 1 Perfusion CT (pCT) can provide rapid, high-resolution information that can be used to assess and stratify treatment of patients with acute stroke. In this article, the authors discuss the use of pCT in the assessment of acute stroke and other cerebrovascular disorders.
Acute stroke
Cerebral perfusion is defined as the steady-state delivery of blood to cerebral tissue through capillaries. Perfusion is measured in mL/100 g tissue/minute and under normal conditions cerebral perfusion is 50 to 60 mL/100 g/minute. An acute stroke is caused by an abrupt localized reduction in cerebral blood flow that causes a reduction in cerebral perfusion. This results in cerebral ischemia and, ultimately, infarction of the compromised cerebral tissue. However, there is often viable tissue surrounding the ischemic core or penumbra. Although the penumbra has a limited blood supply, metabolism is preserved and this tissue can recover if the insult is reversed within a narrow time frame. 2-4 Currently, thrombolysis remains the treatment of choice for acute ischemic stroke within 3 hours after onset of symptoms. 5 Beyond this 3-hour window, no single trial has proven the efficacy of thrombolytic therapy; however, meta-analysis suggests that there is a significant reduction in mortality and disability if treatment occurs within a 3- to 6-hour window. 6 However, thrombolysis carries a risk-as high as 10%-of intracranial hemor-rhage. 7 Therefore, further stratifying patients who will benefit from thrombolytic therapy is vital for optimal management of patients with acute stroke.
Patients presenting with acute stroke are first identified based on neurologic evaluation. The radiologic work-up of acute stroke begins with an unenhanced CT of the brain. A major purpose of cranial CT is to assess for the presence of intracranial hemorrhage. The presence of an intracranial hemorrhage classifies the acute neurologic event as a hemorrhagic stroke and essentially excludes the patient from obtaining thrombolytic therapy. CT is also used to exclude other lesions, such as tumors or abscesses, which can mimic the clinical presentation of stroke. Most importantly, CT is used to detect the suspected area of ischemia. Unfortunately, despite the search for CT signs of acute cerebral infarction, such as a hyperdense middle cerebral or basilar artery, obscuration of the lentiform nucleus or loss of the gray-white junction along the cerebral cortex, the initial CT study is normal in 60% of cases. 8 A technique that can improve the sensitivity of CT for the detection of acute stroke and further stratify patients who will benefit from thrombolytic therapy is necessary and is now available.
Evaluation of cerebral blood flow using pCT
There are various techniques available to evaluate cerebral blood flow and perfusion. Previous investigators have established that cerebral blood flow can be accurately determined by intravascular injection of a bolus of contrast agent followed by rapid image acquisition. 9-11 With respect to CT, cerebral blood flow and perfusion can be measured with the use of a nondiffusible agent, such as an iodine contrast agent, or the use of a diffusible inert gas indicator, such as xenon. Imaging is based upon measuring steady-state delivery of a blood-borne indicator to a given region of cerebral tissue. 12 Perfusion CT utilizes an iodinated contrast agent, which passes through the cerebral arteries and vascular bed. Temporal changes in the CT attenuation are recorded. With this bolus-tracking technique, analysis of time versus concentration curves can provide information regarding cerebral blood flow (CBF), mean transit time (MTT), time to peak enhancement (TTP), and cerebral blood volume (CBV). These parameters can be derived wtih postprocessing software that uses either a maximum slope model to calculate CBF or a deconvolution analysis to calculate MTT and CBV. 13 In the latter case, CBF can be calculated, according to the central volume principle, from the quotient of CBV and MTT (CBF = CBV/MTT).
Perfusion CT has been shown to accurately predict final cerebral infarct size in the acute phase. 14,15 In addition, pCT has been shown to be both safe and feasible; the median time to complete the exam was 23 minutes in one study of 53 consecutive patients. 16 The accuracy of pCT can be further enhanced by using a vascular pixel elimination technique. 17 This method excludes contrast enhancement within large blood vessels that would ordinarily result in overestimation of the quantitative value of cerebral blood flow.
A pCT study begins with an unenhanced CT of the brain (Figure 1). Provided there are no contraindications to further evaluation, the radiologist then localizes a level of the brain for further study based upon the neurologic evaluation of the patient. Current CT scanner technology allows for longitudinal coverage of 2 to 3 cm of the brain during each infusion of contrast. An extended anatomic area can be evaluated using the "toggling table" technique; however, there is loss of temporal resolution because less data is acquired at each location. Increased signal-to-noise and lower-quality images may compromise results with this technique. 18,19 Although the area to be localized depends upon the clinical symptoms and the suspected area of involvement, generally one of the levels includes the basal ganglia, which allows evaluation of the anterior, middle, and posterior cerebral distributions. 19 With a 4-detector-row scanner, scanning is initiated, and repetitive sections are sequentially obtained through the 4 contiguous 5-mm thick slice planes, which span 20 mm of cerebral tissue. The injector is started at the same time as the scanner, and 50 mL of low osmolar nonionic contrast agent (300 mg/mL iodine concentration) is injected at a rate of 5 mL/sec. This rate requires 18- to 20-gauge intravenous access. The acquisition continues for 40 seconds. After 5 minutes, a second axial acquisition is performed, again using 50 mL of contrast agent, which allows an additional 20 mm of cerebral tissue to be studied. 20 Thus, a total of 4 cm of brain parenchyma is covered along the vertical axis, or z-axis.
The first step in processing the information obtained from the acquisition consists of selecting a region of interest (ROI) on a magnified view of one image. An arterial enhancement function or curve for the selected ROI is calculated using commercially available automated perfusion function software. A composite image is then created using all four axial slices, and a color-coded version of the composite image is generated to assist the operator in identifying the ROIs. The operator draws a line to separate the right and left cerebral hemispheres. This line allows the program to identify a mirrored ROI on the contralateral side for comparison. In bolus tracking, the time course of the changing attenuation reflects the changes in iodine contrast agent and is plotted on a time versus concentration curve. A relative value for CBF can be calculated by integrating the area under this curve, which is proportional to the fractional vascular volume of cerebral tissue. The simultaneous measurement of cerebral tissue and arterial attenuation as a function of time enables the calculation of MTT. According to the central volume principle, perfusion, represented by CBF, is equal to the CBV divided by the MTT. Data, including CBF, CBV, MTT, and TTP, are calculated for each ROI. 21 Once the above values are calculated, areas of infarction and the penumbra can be identified. Previous investigators have established that a CBV drop below 2.5 mL/100 g is indicative of infarcted tissue, as is CBF <34% when compared with the opposite (unaffected) side; values that are higher than this threshold but still abnormally low indicate the penumbra. 3,16,19,22 Hunter et al 23 have proposed a probability curve for regional cerebral infarction using normalized pCBV in patients who present with acute stroke. Using this curve can help predict the likelihood of infarction for any given area of cerebral tissue. 23
pCT versus other perfusion modalities
Perfusion techniques have been used for many years to gain information regarding tissue physiology. Xenon, a small, biologically inert gas, has been used in its radioactive form as a freely diffusible agent to measure cerebral perfusion for more than 40 years. 19 More recently, xenon has been used as a contrast agent to measure tissue perfusion, as it can attenuate X-rays in a similar fashion as iodine. Xenon-enhanced CT has been shown to be accurate in the measurement of cerebral perfusion. 24 In fact, xenon-enhanced CT was used to validate perfusion CT data, which demonstrated accurate and reliable results. 25,26 However, xenon-enhanced CT is available in only a limited number of institutions and, therefore, is not a diagnostic option for most patients. Moreover, xenon-enhanced CT requires the use of special equipment for administering the xenon gas. This can be a cumbersome procedure and requires a fairly cooperative patient.
Positron emission tomography (PET) is also a very accurate procedure for assessing cerebral perfusion. This technology is not widely available, however, and its longer imaging time makes it less efficacious in the evaluation of acute cerebrovascular events. Magnetic resonance imaging (MRI) using diffusion-weighted sequences has been shown to be highly accurate in the evaluation of acute stroke, with sensitivities ranging from 94% to 100%. 27-29 Diffusion-weighted imaging has been shown to be superior to both conventional MR and unenhanced CT in the evaluation of acute stroke. 30 When using MRI to identify the penumbra, a combination of perfusion MRI and diffusion MRI yields the greatest accuracy, using the diffusion-weighted studies to identify the region of infarction and subtracting these areas from the perfusion MR images to identify the penumbra. 20,27 When compared with diffusion and perfusion MRI, pCT has been shown to be equivalent in identifying the cerebral penumbra in patients with acute stroke. 22
There are advantages and disadvantages of pCT when compared with perfusion MRI. The greatest advantage of pCT is that, in addition to being widely available, all patients who are candidates for thrombolysis will require an unenhanced CT to exclude intracranial hemorrhage. Perfusion CT adds only a small amount of additional time, requires no specialized hardware or equipment, and generates additional information within the same visit. While MRI is also increasingly available in most institutions, it is still difficult to perform an emergent MRI study on a critically ill patient. The greatest disadvantages of pCT are the exposure of the patient to an additional dose of ionizing radiation, the requirement for the injection of a nonionic intravenous contrast agent, and, currently, the inability to evaluate the entire brain.
Other pCT applications in cerebrovascular disease
Only a few studies have examined the use of pCT in the evaluation of patients with chronic cerebral ischemia. MR techniques to evaluate chronic ischemia are well-established. 31 However, patients with pacemaker devices and aneurysm clips are precluded from MR imaging, and these techniques require rapid scanner gradients and MR-compatible contrast injection devices that are not widely available. 3 Perfusion studies (such as PET and xenon-enhanced CT) coupled with reactivity to an acetazolamide challenge has been used to identify patients who would benefit from invasive interventions to increase regional blood flow.
Perfusion CT has been shown to be a useful tool in the evaluation of chronic cerebral ischemia (Figure 2). Furukawa et al 26 found that, when compared with xenon-enhanced CT combined with an acetazolamide challenge, pCT achieves similar values for relative cerebral blood flow and can serve as an alternative method for the evaluation of chronic cerebral ischemia. This may have utility in the evaluation of flow disturbances that are associated with carotid stenosis in patients with recurrent transient ischemic attacks. Alternatively, it may be possible to assess the status of the collateral circulation in patients with vascular occlusive disease. Perfusion CT and CTA can be used in combination to study patients with intracranial vasospasm following subarachnoid hemorrhage. Other potential applications for pCT include the evaluation of vascular steal phenomena, such as in subclavian steal, arteriovenous malformations, and direct or indirect carotid cavernous fistulas. With pCT, it may be possible to study the sequelae of intracranial mass effect, such as those observed in patients with subdural hematomas or other intracranial masses. Certainly, pCT can be used as a postprocedural probe to assess the efficacy of cerebrovascular revascularization procedures, such as external carotid artery bypass, intra- or extracranial arterial stenting, angioplasty, papaverine infusion, or encephaloduroarteriosynangiosis.
Conclusion
Perfusion CT is a convenient, safe, and feasible imaging modality that provides a wealth of information about cerebrovascular perfusion, including identification of the penumbra. It promises to afford clinicians an efficient means to further stratify patients, thereby improving upon the existing algorithm in the management of patients with both acute and chronic cerebrovascular diseases. Many questions still remain to be answered, and although further research is required before making its application widespread, preliminary results made by the principle investigators and the enthusiasm that the radiologic community has shown for pCT predict that these questions will soon be answered.