New perspectives and challenges in abdominal 3T MR imaging
Images
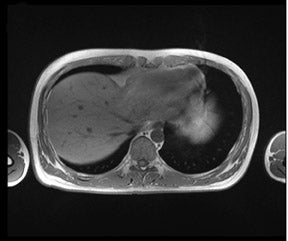
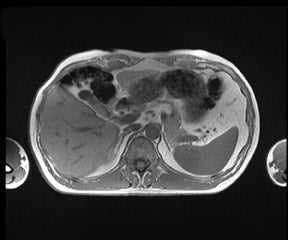
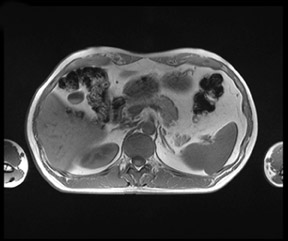
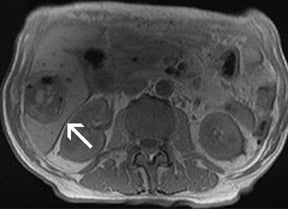
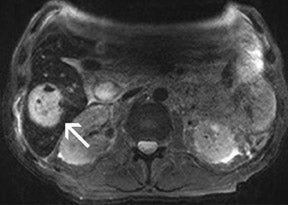
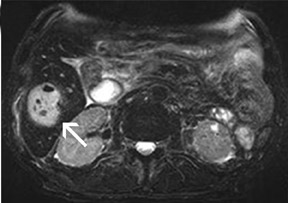
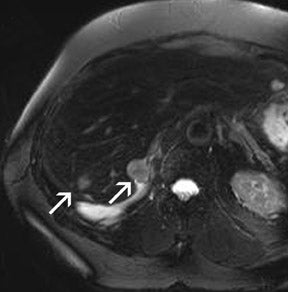
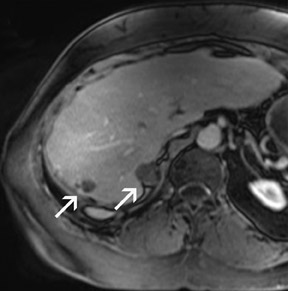
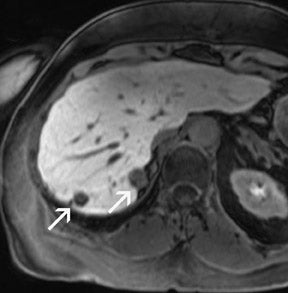
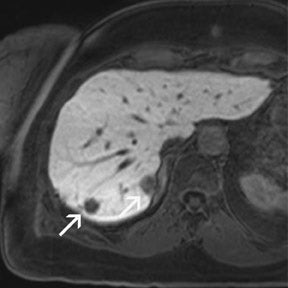
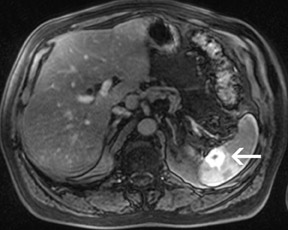
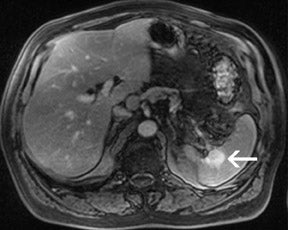
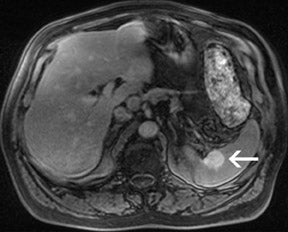
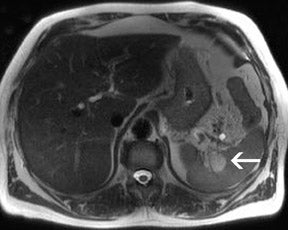
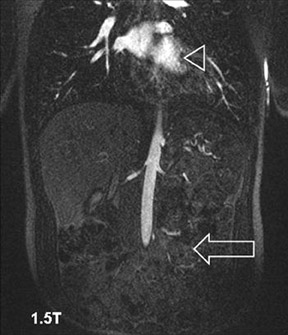
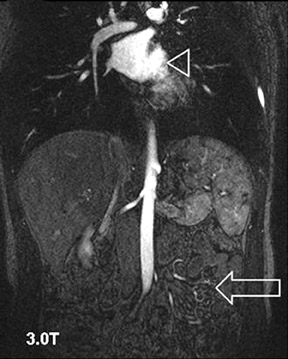
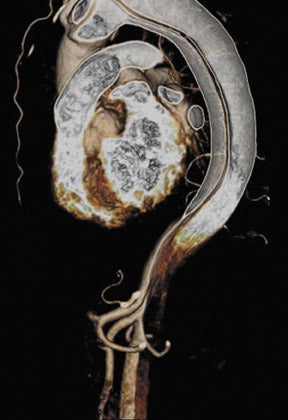
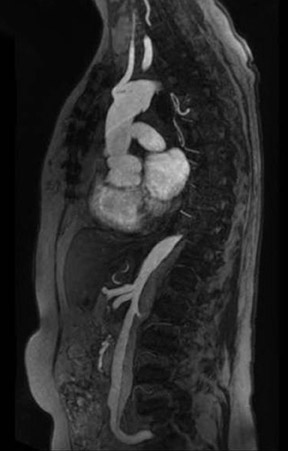
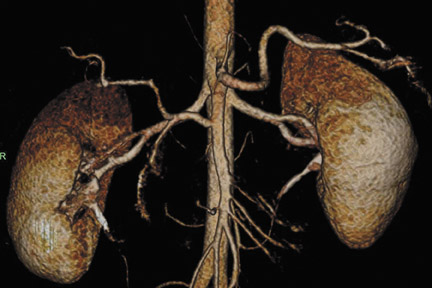
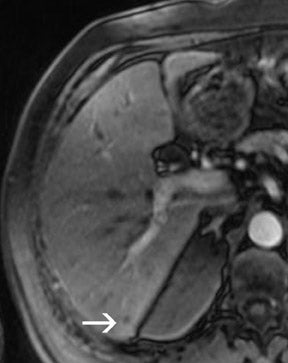
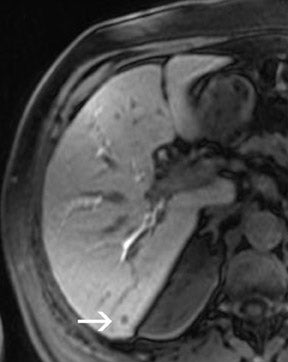
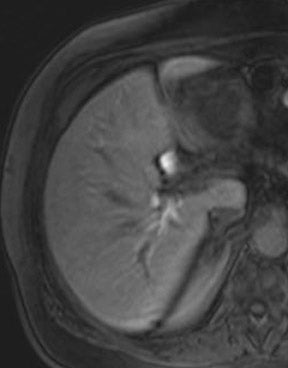
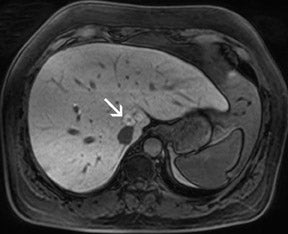
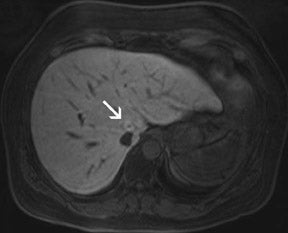
Dr. Schoenberg is an Associate Professor of Radiology, an Associate Chair for Clinical Operations, and the Section Chief, Magnetic Resonance Imaging; Dr. Zech is a Fellow of Radiology and Vice Section Chief, Abdominal Imaging; Dr. Panteleon is a Resident; and Dr. Michaely is a Resident; Institute of Clinical Radiology, University Hospitals-Grosshadern, Ludwig-Maximilians-University, Munich, Germany. Dr. Reiser is a Professor of Radiology and the Chairman, Institute of Clinical Radiology, University Hospitals-Grosshadern and Innenstadt, Ludwig-Maximilians-University, Munich, Germany. Dr. Finn is Professor of Radiology, Cardiovascular Research, Department of Radiology, David Geffen School of Medicine at the University of California,Los Angeles, CA.
Currently, the challenges for abdominal magnetic resonance imaging (MRI) are confined within a magic triangle of spatial resolution, signal-to-noise ratio (SNR), and artifacts from respiratory motion or vascular pulsation. Within the last few years, a number of strategies have been developed to overcome these limitations. The use of parallel acquisition techniques on MRI systems with multiple receiver channels now allows the increase of spatial resolution at almost no cost in scan time. 1,2 Motion artifacts can successfully be avoided by the application of navigator-based correction techniques, thereby synchronizing the image acquisition with the position of the diaphragm. 2,3 Lastly, SNR constraints can now be effectively overcome by the introduction of 3T MRI systems into the clinical routine. To truly assess the additional value of 3T systems for abdominal MRI, a fair comparison must be made to current state-of-the-art 1.5T MRI systems. This way it is possible to evaluate the benefit of 3T in view of the higher hardware costs and the substantial increase in technical difficulties.
At the present time, state-of-the-art 1.5T MRI scanners are equipped with at least 8 to 32 independent receiver channels, allowing the use of parallel acquisition techniques (PATs) in all 3 directions with acceleration factors of up to 6. For PATs, essentially 2 different algorithms are applied, which perform the reconstruction either in the image domain (sensitivity-encoded [SENSE]-based algorithms) 4 or k-space domain (simultaneous acquisition of spatial harmonics [SMASH]-based algorithms). 5-8 Dependent on the vendor-specific implementation, the information of the coil sensitivity profiles is either acquired within a SENSE sequence or by acquisition of additional reference lines within the same scan (modified SENSE [mSENSE] and generalized autocalibrating partially parallel acquisition [GRAPPA]). 9 The latter implementation is known as an integrated parallel acquisition technique (iPAT).
Current state-of-the-art imaging at 1.5T
With state-of-the-art 1.5T scanners, a slice thickness of 4 to 6 mm for abdominal imaging can routinely be applied with 2-dimensional sequences and 2 to 4 mm with 3-dimensional (3D) sequences.Acqui-sition of a (320) 2 matrix at a (350 mm) 2 field of view allows obtaining an in-plane resolution of approximately 1 mm 2 routinely. By combining PATs with prospective navigator correction (eg, in T2- weighted [T2W] turbo spin-echo [TSE]) sequences, the image acquisition of larger organs, such as the liver, is feasible in 3 breath-holds with a breath-hold time of only 13 seconds. 2 For free-breathing acquisitions, the total acquisition time can be effectively reduced by PATs to approximately 4 minutes. Studies have shown that the use of PAT does not result in a substantially larger number of artifacts from aliasing, while at the same time, motion artifacts are decreased because of the shorter overall scan times. 2 With these techniques, liver lesions can now be reliably detected with T2W sequences at a size of 4 to 5 mm or less after the administration of superparamagnetic iron oxides (SPIO). It has been shown that the combination of dynamic gadolinium-enhanced imaging using thin-slice 3D T1-weighted (T1W) gradient-echo (GRE) sequences with SPIO imaging further improves the detection of small lesions (<10 mm). 10
Higher PAT acceleration factors can be applied in sequence types that have an intrinsically high SNR, such as steady-state free-precession [SSFE] techniques (eg, true fast imaging with steady-state precession [TrueFISP], balanced fast-field echo [FFE], and fast imaging employing steady-state acquisition [FIESTA]). These techniques are particularly favorable for single-breath-hold 3D imaging of the small and large bowel after intraluminal water distension with acceleration in 2 phase-encoding directions. A total PAT factor of 6 allows the acquisition of an entire data set with 1.5-mm isotropic voxel length in a single breath-hold of <20 seconds. This data can be individually reformatted to show the exact extent of small or large bowel pathology.
Currently, pancreatic imaging can also be performed at very high resolutions by the use of PAT, (320) 2 to (384) 2 matrix size, and a slice thickness of 4 mm after the administration of manganese-containing contrast agents such as Teslascan (Amersham Health, Ismaning, Germany). 11 Similarly, the advent of PAT has revolutionized the use of contrast-enhanced 3D MR angiography (MRA) in the abdomen. Voxel sizes of <1 mm 3 are now achievable within a single breath-hold. 12-14 These isotropic data sets can also be reformatted in any plane, which offers the possibility to assess the area stenosis rather than only the diameter stenosis. These area stenosis measurements have shown good correlation to intravascular ultrasound in preliminary studies on renal arteries and appear to be significantly more accurate in terms of the measurement of the true degree of stenosis. 14
Capabilities at 3T
As already outlined, the current achievements at 1.5T apply a high standard in abdominal MRI. Thus, the initial step at 3T was to reproduce similar image parameters as compared with 1.5T. The problem is that there are a number of challenges for abdominal MRI at 3T despite the obvious advantage of a higher SNR. First, the specific absorption rate (SAR) increases quadratically with field strength, resulting in a major limitation, particularly for turbo spin-echo sequences. 15 The shorter wavelength at 3T results in substantial dielectric resonance effects, causing significant signal inhomogeneities due to eddy currents in the tissue, with the result of focal-field cancellation. 15 Relaxation times are substantially longer, which necessitates the adjustment of several imaging parameters (eg, repetition time [TR], echo time [TE]).
Therefore, the second step for abdominal imaging at 3T has been to effectively address these limitations by new technical strategies. The signal inhomogeneities due to the dielectric resonance effects can be substantially reduced by the application of external gel pads placed on the surface of the patient. These radiofrequency (RF) homogenization pads are not visible in the images, nevertheless they alter the dielectric properties of the imaging volume, improving signal homogeneity. 16 The SAR can be effectively reduced by new techniques (such as the hyperecho technique and the variable flip angle technique) 17-19 or parallel imaging. 20 In the third step, these new techniques have been effectively implemented on clinical scanners to further push the limits. At the present, matrix sizes of (512) 2 and slice thicknesses of <4 mm appear feasible, while at the same time breath-hold scans of <12 seconds or total scan times for free-breathing acquisitions of <2 minutes can be achieved using PAT.
Image optimization
The following sections discuss the individual steps for the optimization of image acquisition for abdominal 3T MRI.
Optimization of image contrast
As the T1 relaxation time increases with the field strength B 0 (with a typical increase of 30% to 50% of T1 at 3T compared with 1.5T), the sequence parameters-particularly the TR-has to be adapted. 21 The effects on the T2 relaxation are less prominent; T2 is effectively unchanged at 3T, but T2* is shortened. 22 The adjustment of the TR strongly depends on the flip angle. Therefore, GRE sequences with small flip angles might require only minor changes in TR compared with sequences with a full 90° flip angle. 21 In daily practice at our institution, we choose a decreased flip angle of only 50° for T1W GRE sequences because this flip angle has been shown to produce optimal image contrast with no adaptations of the TR. Figure 1 depicts an example of the excellent image quality in this T1W sequence.
Changes of TEs for in-phase/ opposed-phase imaging at 3T
Due to the change of the resonance frequency, TEs for in-phase and opposed-phase conditions at 3T have to be adjusted accordingly. The values are almost inverted compared with the conditions at 1.5T. In-phase conditions are found at TEs of 2.3 msec and 4.6 msec, while opposed-phase conditions are present at 1.1 msec, 3.5 msec, and 5.75 msec. Therefore, dual-echo sequences are problematic, since the echo spacing between the initial opposed-phase echo at 1.1 msec and the following in-phase echo of 2.3 msec are too close for acquisition within a single readout.
Optimization of fat suppression at 3T
There are opposite effects at 3T that improve as well as deteriorate the quality of fat suppression. The different resonance frequency at 3T produces a stronger chemical shift between fat and water, thus facilitating the use of spectral fat suppression. At the same time, B 1 homogeneity decreases with B 0 field strength, which results in a more pronounced variation of the flip angle over the field of view. This might result in a less optimal fat suppression, which is particularly problematic for TSE sequences, while this effect is less problematic with GRE techniques. In addition, as the field increases, stronger eddy currents are generated that further reduce the quality of fat suppression. In daily practice, fat suppression remains inhomogeneous; nevertheless, this does not lower the accuracy of imaging evaluation of liver diseases, since the inhomogenous fat suppression mostly affects the structures of the abdominal wall only (Figure 2). A recent study the authors conducted showed that, despite the inhomogeneous fat suppression and other specific artifacts at 3T, the delineation of liver lesions had no significant difference between 3T and 1.5T images (Figure 3). 23 Further solutions for optimized fat suppression are improved gradient-coil design (generating less eddy currents) as well as an alternative, non-RF-dependent type of fat suppression. This includes, for example, the Dixon method, which uses several in-phase and opposed-phase images or inversion recovery-type techniques. 24
Increased chemical shift at 3T
As mentioned above, the different resonance frequency at 3T produces a stronger chemical shift between fat and water. This can be advantageous for the use of spectral fat suppression and for MR spectroscopy or chemical shift imaging; however, chemical-shift artifacts are also more pronounced at fat/water interfaces. This increase of chemical-shift artifacts can be addressed by using a higher receiver bandwidth, with the consequence of losing some of the SNR advantages gained by 3T.
Reduction of SAR
As mentioned earlier, the 3 most important techniques for reduction of SAR are new pulse sequences that apply the variable flip angle-technique (VFA) or the hyperecho technique (which is a modification of the former), and parallel imaging (which is discussed below). The VFA technique was initially described by Mugler et al 25 and is particularly used for SSFP sequences that offer high SNR efficiency if large flip angles are applied (α = 60°). However, the SAR also increases quadratically with the flip angle. Since the image contrast is mainly affected by the center of k-space, the flip angle is varied along the phase-encoding direction, ensuring a large flip angle in the central parts of k-space, while the flip angle is continuously decreased for the other segments of k-space. This results in a reduction of SAR by at least 50%. The main disadvantage of this technique is that SNR is also reduced by approximately 30%, thus limiting the benefit of the normally twofold SNR gain at 3T compared with 1.5T. A further refinement of the VFA technique is the hyperecho technique, which is used for multiecho sequences such as TSE sequences with long echo trains or single-shot acquisitions (eg, half-Fourier acquisition single-shot turbo spin echo [HASTE], rapid acquisition with relaxation enhancement [RARE]). In this technique, magnetization can be completely rewound after any arbitrary sequence of RF pulses. 18,19 Only the echoes that encode for the center of k-space are refocused by 180° RF pulses. Stimulated echoes are also obtained because of a series of RF pulses with low flip angles. These stimulated echoes are used for the image reconstruction together with the conventional echoes as long as they are in-phase with the latter. This technique results in the reduction of SAR by 60% to 80%, while the reduction of SNR is virtually negligible. Simpler techniques to reduce the SAR include the global decrease of the refocusing flip angle in TSE sequences from 180° to a value between 100° and 120°. Compared with the hyperecho approach, however, a larger SNR decrease must be accepted. In fast GRE sequences, the SAR can be reduced by choosing a longer RF pulse, since the RF pulse power scales inversely with the square of the pulse length. The primary parameters used in our institution are T2W fat-saturated TSE with breath-hold, TR of 3500, TE of 103l, and a flip angle of 140°. For a respiratory-triggered T2W fat-saturated TSE, we recommend the use of the following parameters: TR according to the respiratory cycle, TE of 100, and a flip angle of 140°. Only rarely do these parameters have to be changed (namely, decrease of the flip angle) because of SAR limitations.
Reduction of dielectric resonance effects
Dielectric resonance effects are caused by local eddy currents because of the increased conductivity of the tissue. These effects are more enhanced at 3T, since the RF waves have a shorter length as compared with 1.5T. This causes local inhomogeneous areas, particularly in larger anatomic areas such as the abdomen, where the wavelength is approximately only half the diameter of the body, particularly for patients with low fat content. Nevertheless, this does not necessarily influence the diagnostic value of the examination, as recent studies with excellent image quality at 3T and 1.5T have shown. Zech et al 23 stated that despite dielectric artifacts, there has been no relevant difference in the diagnosis of liver lesions. More precisely, the delineation of liver lesions in the left lobe, where the dialectic artifact is frequently present, has been as accurate as the delineation at 1.5T. 23 In the MR images, dielectric effects are predominantly noticeable in the ventral aspects of the abdomen as focal areas of varied signal intensity. They can be effectively reduced by the placement of an external dielectric gel pad (see above) on the anterior body surface, which increases the resistance for focal eddy currents. 16 For standard abdominal imaging, this improvement is usually sufficient; however, there are certain virtually unavoidable conditions in which substantial artifacts lead to nondiagnostic image quality. These include physiologic or pathologic conditions that involve large amounts of intra-abdominal or intrapelvic fluid, such as pregnancy with a large amount of amniotic fluid or intra-abdominal ascites. In those cases, images can be almost nondiagnostic because of focal signal intensity drop-outs. 26
Parallel acquisition techniques
With PAT, the SNR is reduced by the square root of the acceleration factor times the so-called g-factor, which represents a measure of the local noise amplification, mostly related to array coil design characteristics. 4,7,8 Thus with higher acceleration factors of 3, for example, the SNR is reduced to at least 58% of the original value. This causes a visible increase of image noise in the images at 1.5T. Because of the higher SNR at 3T, image noise at comparable acceleration factors is barely visible in the images. Further development can be expected from dedicated multichannel MR systems, such as the Magnetom TRIO with TIM (Siemens Medical Solutions, Erlangen, Germany and Malvern, PA). An initial study has shown that the new multielement array (matrix) coils allow for the application of acceleration factors of up to 4 without noticeable loss of image quality from increased image noise. 20
With all of these optimization strategies at 3T, a robust image quality can be obtained in the majority of patients with slice thickness between 4 and 6 mm, matrix sizes between (320) 2 and (384) 2 , and PAT acceleration factors between 2 and 3. With multi-breath-hold imaging, the larger abdominal organs, such as the liver, can be covered in 3 breath-holds, each lasting 11 seconds, using PAT. For single-shot TSE techniques, such as HASTE (Figure 4), the effective reduction of SAR by the hyperecho technique allows the acquisition of 15 to 20 slices in a single breath-hold with a (384) 2 matrix without exceeding the SAR limitations. Slice thicknesses down to 4 mm are feasible even when PAT is used. The overall results for high-resolution images with a decreased amount of blurring is better with the hyperecho technique, as it allows for the use of higher flip angles compared with the standard VFA technique with reduced flip angles (down to 60°) for the refocusing pulse and, therefore, alternated image contrast. For free-breathing techniques, the use of PAT for high-resolution images with matrix sizes of (384) 2 allows for total acquisition times of <1.5 minutes.
The combination of several of these described techniques can be used to optimize dedicated techniques for abdominal imaging, such as 3D TSE MR cholangiopancreatography (MRCP). For this type of sequence, a free-breathing acquisition is performed with navigator correction. To effectively reduce SAR in view of the long echo trains, a variable flip angle technique is applied. For maintaining high SNR, the remaining magnetization after the readout is restored into the longitudinal direction by a dedicated 90° restore pulse. The SNR gain from those restore-pulses in combination with the higher field-strengths allows the application of PAT factors of up to 4. Thus, 3D data sets with an isotropic voxel size of (0.9 mm) 3 can be acquired in only 2 minutes, allowing 3D assessment of the entire biliary tree by a variety of postprocessing possibilities, such as multiplanar reformats (MPR), maximum-intensity projections (MIP), surface-shading techniques (SSD), or volume-rendering techniques (VRT).
Abdominal and renal MRA requires high spatial resolution acquired within a short scan time to accurately grade stenoses and to display subtle vessel wall changes such as fibromuscular dysplasia. 27 At 3T, high-resolution MRA of the abdominal vessels is feasible with voxel sizes of 0.9 × >0.8 × 0.9 mm 3 with a PAT acceleration factor of 3 in 18 seconds on a routine basis. 28 Despite the small voxel sizes (which can be reduced by about 20% compared with high-resolution MRA at 1.5T 29,30 ) and despite the application of a PAT acceleration factor of 3, sufficient SNR is still present at 3T. 31 Apart from the increased SNR at 3T, MRA also benefits from the prolonged T1-times of most tissues, while the relaxivities of the contrast agents are only slightly lowered. Overall, this leads to an increased contrast agent efficacy at 3T. Preliminary data suggest that the administered amount of contrast agent can be cut back without impairing the image quality. 29 In addition, the high SNR at 3T in combination with new acquisition techniques, such as time-resolved imaging of contrast kinetics (TRICKS), 32 may foster the widespread use of time-resolved MRA studies at 3T.
Problems for MRA at 3T are twofold. First, the typically short TRs of <4 msec may result in a high RF power deposition, which easily exceeds the SAR limits or the stimulation threshold. One possible approach is to lengthen the RF pulse and the TR and to use PAT to compensate for the consecutive increase in scan time; however, this changes the contrast of the image, since a longer repetition time increases the background signal. This might decrease the vessel-background contrast and limit the use of MIPs for the diagnostic evaluation of the MRA. Similarly, decreasing the flip angle, which may be necessary to stay within the SAR limits, might also increase the background signal. Fortunately, GRE sequences used for MRA are not susceptible to dielectric artifacts, which are often encountered in T2-weighted sequences. Nevertheless, in the clinical routine, a compromise can usually be found by carefully adjusting these parameters such that high-resolution submillimeter MRA is feasible with good image quality. 27,28,31 This is of high importance, since intermediate spatial resolution in MRA might lead to insufficient diagnostic accuracy, as shown by a recent publication on MRA at 1.5T 33 (Figures 5 through 7).
Clinical value of 3T for abdominal MRI
In general, those applications for abdominal MRI seem to profit from the higher field-strength that critically depends on spatial resolution. One promising application appears to be the assessment of multicystic abdominal masses, in which the delineation of enhancing septa is important for the detection of malignancy. 34 This includes multicystic masses of the kidney and ovaries, in which small nodular thickening of the septa with increased enhancement after gadolinium administration is indicative of early malignancy. 35 With an optimal spatial resolution, the differentiation between cysts and renal cell carcinoma in von Hippel-Lindau disease, the differentiation between cystadenoma and cystadenocarcinoma in cystic ovarian lesions, and the early detection of renal cell carcinoma in acquired polycystic disease can be facilitated. 34-36
For the assessment of focal liver le-sions, there is still no consensus whether the high resolution truly converts into a better detection of small lesions (<1 cm) (Figure 8). However, it has already been shown in small, noncontrolled studies that a high spatial resolution (eg, with 3D GRE sequences) helps to improve the detection of small lesions and the characterization of lesions in terms of better delineation of morphological features, such as the vascular architecture or a central scar 37,38 (Figure 9). As high-field MRI (with its high spatial resolution) is not limited by a decreased liver-to-lesion contrast (as is the case with computed tomography), it can be expected that the high resolution should improve overall lesion detection. 39,40 The dilemma at this point, however, is that because of the reduced availability of 3T in the clinical routine, no larger systematic studies comparing 1.5 and 3T correlated with an invasive gold standard, such as biopsy or surgery, have been performed.
An area that holds great promise is the staging of malignant lesions of the uterine cervix and the rectum. 41 MRI at 1.5T has been notoriously weak in differentiation between stage IIA cervical carcinoma and stage IB, with an accuracy of only approximately 80%. 42 This question is highly relevant, since differentiation between those 2 stages affects the decision for either radical hysterectomy or primary radiation therapy. Since the extension of the tumor beyond the cervical stroma, which is sometimes <1 mm in width, is the discriminator between those 2 stages, one might expect an improved staging with matrix sizes higher than (512) 2 . Similar improved performance could be expected for staging rectal carcinoma, in which MRI is known to overstage lesions of TNM stage T1 as T2 lesions, since reliable differentiation between the submucosa and muscularis propria layers is difficult at the current maximum matrix size of (512) 2 . 43,44 Recent publications also re-port that increased spatial resolution is helpful for the evaluation of the nodal staging in colorectal cancer because the better visualization of morphologic features help distinguish malignant from benign lymph nodes. 45
One of the most promising applications is high-resolution 3D MRCP for diseases of the biliary tree, which predominantly manifest in the high-order branches in their early stages, such as primary sclerosing cholangitis (PSC). 46 Reliable visualization of the biliary tree with MRCP at 1.5T is possible only up to the second-order branches with current techniques. 47 Compared with endoscopic retrograde cholangiopancreatography (ERCP), MRCP has been proven to be cost-effective, especially because of its lack of any side effects and complete noninvasiveness. Despite this, it has not established a role for the initial detection of PSC disease manifestation. 48 This patient group, however, particularly benefits from the noninvasive techniques, since they are frequently young patients and early treatment can substantially affect the cause of their disease.
Untreated patients may experience severe complications, such as multiple strictures of the intra- and extrahepatic bile ducts, which ultimately may progress to cirrhosis and liver failure. 49 Moreover, in these patients, ERCP for initial diagnosis can lead to substantial side effects such as pancreatitis or common bile duct perforation, which is observed more frequently than in other patients undergoing ERCP. 50 With voxel sizes of >0.9 × 0.9 × 0.9 mm 3 spatial resolution and images free of motion artifacts with scan times of roughly 2 minutes, 3D MRCP at 3T now allows for the detection of high-order branches of the biliary tree in volunteers without biliary disease. Our own preliminary data show that focal dilatations and stenoses of third- and fourth-order branches, which are often the early manifestation of PSC, can be well detected with good correlation to ERCP. The acceleration of the acquisition by a PAT factor up to 4 makes this an easily feasible technique for these patients. MR cholangiopancreatography can also be combined with high-resolution liver imaging at 3T with a slice thickness of only 3 mm, which may allow for the earlier detection of malignant tumors of the bile duct, such as cholangiocarcinoma.
Ultra-high-resolution contrast-enhanced breath-hold MRA with 0.7- to 0.8-mm isotropic resolution might eliminate the need for diagnostic digital subtraction angiography (DSA) of the mesenteric and renal arteries. A recent publication in the Annals of Internal Medicine has shown that MRA at 1.5T with a current spatial resolution of only 1.5 mm or even worse is not sufficient to accurately diagnose and stage the degree of renal artery stenosis. 33 Initial results at 1.5T with submillimeter (0.8 × 0.8 × 0.9 mm 3 ) isotropic spatial resolution and reformats of the vessel area perpendicular to the vessel axis show that assessment of the area stenosis is a much more reliable parameter for exact grading of the degree of stenosis than for grading the diameter stenosis in-plane. 14 It is expected that this improvement will further increase at 3T.
Conclusion
One has to accept that imaging of the abdomen at 1.5T on a state-of-the-art system with parallel imaging, navigator correction, and an advanced sequence design is a tough competitor for the evolving field of 3T. Nevertheless, it can be stated that it is now feasible to acquire single-shot TSE images with PAT and hyperecho technique at a very high resolution with minimal blurring and within the SAR limits. Those images appear to be superior to those currently acquired at 1.5T (as shown in Figures 8 and 9). Parallel acquisition techniques are generally applicable without major SNR constraints, allowing for the use of higher acceleration factors for shorter breath-holds. The fat suppression appears acceptable despite problematic dielectric resonance effects. For techniques such as 3D TSE MRCP, ultra-high-resolution submillimeter studies with minimal acquisition times are feasible and may also eliminate the use of diagnostic ERCP for diseases, such as primary sclerosing cholangitis, in the future. No larger systematic studies have yet been performed for the detection and characterization of small focal liver lesions. It appears, however, that there is improvement at 3T, since in MRI the lesion delineation is primarily limited by the spatial resolution rather than by the liver-to-lesion contrast because of the excellent soft-tissue contrast. The resolution of contrast-enhanced 3D MRA approximates that of DSA for the first time, which should eliminate the use of diagnostic renal or mesenteric DSA other than for rare diseases, such as small vessel vasculitis. In the pelvis, ultra-high-resolution imaging with SE and TSE sequences might further improve the staging of early T1 and T2 tumors of the cervix, uterus, and rectum. The true gain, however, in clinical value is still speculative at the present, since no large comparative studies have yet been performed or published.