Myocardial viability
Images
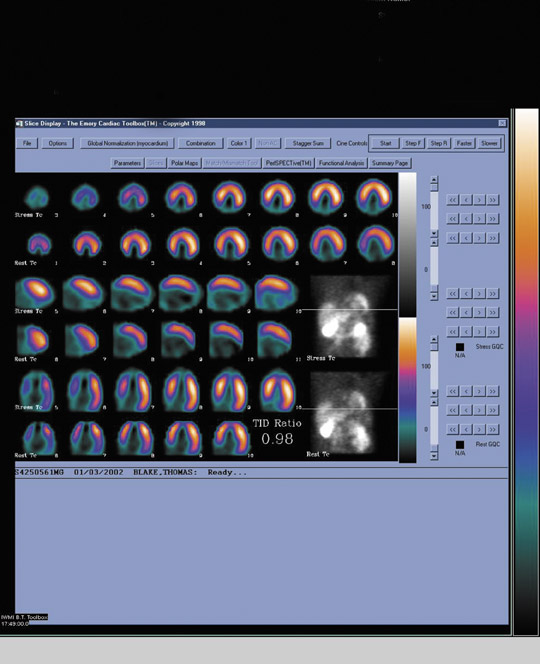
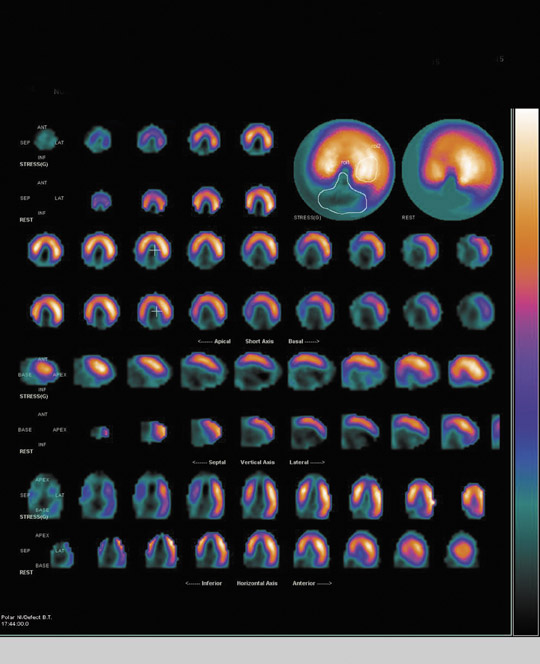
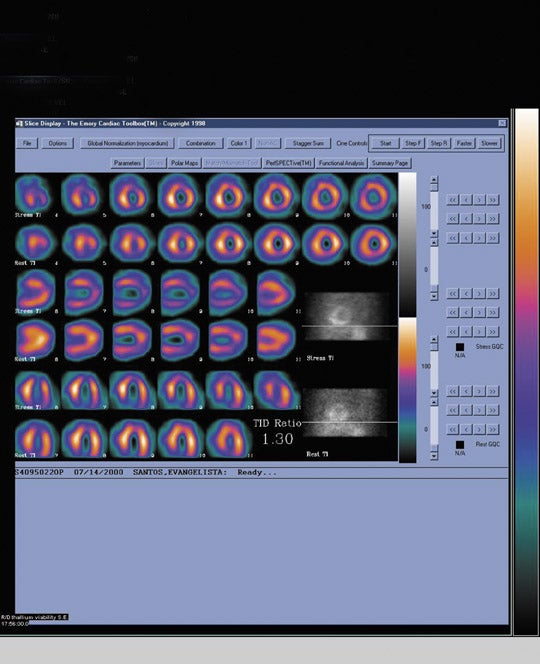
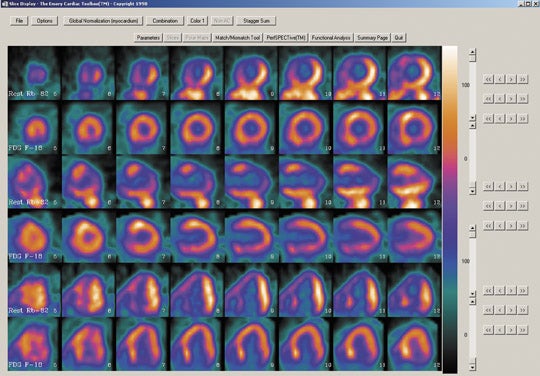
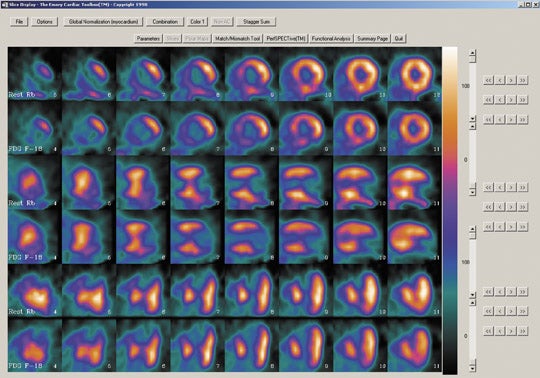
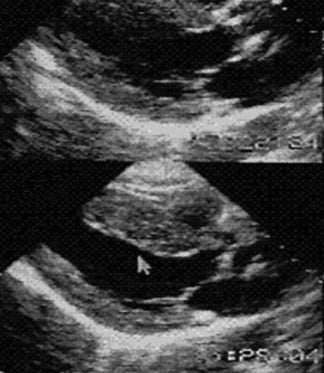
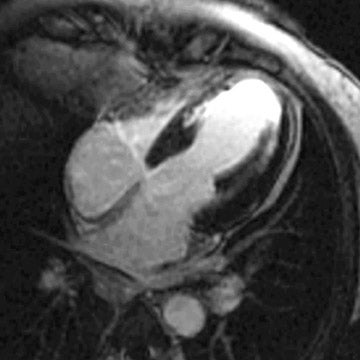
Dr. DePuey is the Director and Dr. Ghesani is the Roosevelt site Director, in the Division of Nuclear Medicine, Department of Radiology, St. Luke's-Roosevelt Hospital and Columbia University College of Physicians and Surgeons, New York, NY.
Heart failure may be a consequence of either nonischemic or ischemic cardiomyopathy. Bonow et al 1 pooled 13 randomized multicenter heart failure trials and observed that coronary artery disease was present in approximately 70% of >20,000 enrolled patients. Coronary disease may result in repetitively stunned and/or hibernating myocardium, both potentially reversible causes of heart failure. Whereas patients with heart failure due to noncoronary etiologies may best benefit from medical therapy or heart transplantation, coronary revascularization has the potential to improve ventricular function, symptomatology, and long-term survival in those patients with heart failure attributable to repetitive stunning and/or hibernation.
Viable myocardium may be characterized by several attributes, including cell membrane integrity, intact mitochondria, preserved glucose metabolism, preserved fatty acid metabolism, intact resting perfusion, and inotropic reserve. 2-5 Not all of these are present in every viable myocardial segment. Therefore, a combination of techniques to evaluate several of these parameters is generally used clinically in considering if repetitively stunned or hibernating myocardium exists as a cause of reversible left ventricular dysfunction, warranting coronary revascularization. Cell membrane integrity can be evaluated by assessing delayed thallium-201 uptake 1,6 or the exclusion of magnetic resonance imaging (MRI) contrast in delayed images 7-9 (absence of delayed hyperenhancement). Technetium (Tc)-99m sestamibi and Tc-99m tetrofosmin are radiotracers whose uptake is related to the presence of intact mitochondria. 10-12 F-18 fluorodeoxyglucose positron emission tomography (FDG-PET) and single-photon emission computed tomography (SPECT) assess glucose metabolism. 13-23 Free fatty acid metabolism can be evaluated with I-123 IPPA 24 and I-123 BMIPP. Resting perfusion can be evaluated by a variety of methods, including Tc-99m sestamibi and Tc-99m tetrofosmin uptake and immediate thallium-201 uptake as well as the transit of echocardiographic, MRI, and radiographic contrast material. Coronary flow reserve may be estimated by a comparison of nitrate-enhanced Tc-99m sestamibi uptake with baseline uptake. Inotropic reserve can be determined echocardiographically as an in-crease in regional left ventricular function after low-dose dobutamine infusion. 25
Myocardial SPECT with Tc-99m cardiac flow tracers
Theoretically, the volume of viable myocardium and, consequently, the degree of regional functional recovery should be directly related to resting myocardial perfusion. Therefore, functional recovery should be proportional to the amount of uptake of resting flow tracers, such as Tc-99m sestamibi, in regions of left ventricular dysfunction shown by gated perfusion SPECT, echocardiography, or contrast ventriculography. Indeed, published reports 14,26-28 have shown that regional functional recovery following revascularization increases in proportion to Tc-99m sestamibi uptake. In those segments with >55% maximal uptake the likelihood of functional recovery is >70% (Figure 1). However, there are several drawbacks to this approach. First, factors such as normal physiological variations in tracer distribution (eg, decreased in-ferior count density in men) confound this type of semiquantitative analysis. Secondly, in actual clinical practice, there are many segments in which viability is questioned when relative uptake falls between 50% and 60%, rendering the results equivocal. Finally, and perhaps most importantly, there are 2 different conditions that may result in a mild-to-moderate decrease in uptake of radionuclide flow tracers: 1) chronically decreased blood flow in hibernating, viable myocardium, and 2) a nontransmural infarct supplied by either a patent or an occluded proximal coronary vessel. Whereas revascularization will likely be beneficial in the former situation, it will not be likely to improve regional function in the latter.
Because of these limitations of semiquantitative analysis of a single SPECT image acquired after the injection of a radionuclide flow tracer, several investigators, particularly from Italy, 10,11 have used improvement in the regional concentration of a flow tracer injected after intravenous, oral, or sublingual nitrate administration as a marker of viability. Tracer uptake should increase in the distribution of hibernating myocardium supplied by a patent coronary artery. Such enhancement in tracer uptake will not be observed in the presence of baseline-perfusion abnormalities due to attenuation artifact, physiologic regional variations in tracer distribution, or nontransmural myocardial infarction.
Myocardial viability with Tl-201
The most widely used radionuclide method to assess myocardial viability is rest/delayed thallium-201 SPECT. When Tl-201 is injected at rest, it is distributed to the myocardium proportionally to coronary arterial blood flow. At a delayed 3- to 5-hour interval, the radiotracer washes out and redistributes. The pattern of redistribution is dependent upon an intact Na-K ATPase pump and thus myocardial cell viability. Myocardial scars will demonstrate a nonreversible defect in the immediate and delayed images, whereas regions of resting ischemia of viable myocardium will demonstrate reversibility in the delayed scan (Figure 2). Although the degree of defect reversibility necessary to identify viable tissue that will recover function following revascularization remains somewhat controversial, regions that demonstrate >15% reversibility may generally be considered to contain viable tissue.
The performance of a viability scan using Tl-201 is straightforward. The patient receives an intravenous injection of 3 to 4 mCi of Tl-201 at rest. SPECT images are acquired 10 minutes after injection and again at a 3- to 5-hour delayed interval. It is important that the patient is not reinjected with a "booster" dose of Tl-201 prior to the delayed image. Some laboratories acquire an additional 24-hour delayed image (also without tracer reinjection) in patients who demonstrate no defect reversibility at 4 hours, although we have not found this technique to be of particular additional value in assessing viability. Although stress/delayed Tl-201 imaging to detect coronary ischemia has, by and large, been replaced with separate stress and rest imaging using the Tc-99m- labeled radiopharmaceuticals, myocardial viability may be assessed in conjunction with a stress/rest Tl-201 study. Delayed images can be acquired at 24 hours when Tl-201 redistribution will demonstrate viability. Alternately, patients can be injected with a "booster" dose of Tl-201 1 hour prior to the 4-hour delayed resting image acquisition. The booster dose is distributed proportionally to resting perfusion, and the delay between injection and imaging allows for redistribution to assess viability. However, this latter "hybrid" method is not as physiologically straightforward as the former 2 methods that use Tl-201.
A number of studies have evaluated the accuracy of delayed thallium uptake in predicting functional recovery. More than half of these studies used rest-redistribution protocols wherein radionuclide imaging was performed immediately after thallium-201 injection and again at a delayed interval. 1,6,26,29 The mean sensitivity in predicting functional recovery was 86%. However, specificity was only 59%, indicating that there was no evidence of regional functional recovery following revascularization in 41% of patients with delayed thallium-201 uptake. However, it is now known that functional recovery may not occur until as long as 6 months after revascularization in patients with hibernating myocardium (in contradistinction to those with only stunning). Many of these published studies performed follow-up for only ≤3 months, so actual specificity may have been underestimated. Moreover, it is known that the probability of functional recovery increases with increasing amounts of jeopardized myocardium. Some studies failed to establish a threshold for the degree of defect reversibility as a predictor of recoverable function. Finally, we must keep in mind that improved outcome and long-term survival following revascularization may be due not only to functional recovery but also to the prevention of remodeling and arrhythmogenesis afforded by revascularization. However, it has yet to be shown that these latter beneficial effects of revascularization can be predicted by thallium-201 viability imaging protocols. Although Tl-201 SPECT is a widely available option for assessment of myocardial viability, there are reports of significantly higher sensitivity of FDG-PET when compared with Tl-201 rest-redistribution imaging, as described below. 30,31
F-18 FDG PET and SPECT
Positron emission tomography is being increasingly recognized as a noninvasive imaging technique with clinical applications in cardiology, oncology and neurology. The principal radiopharmaceuticals used in cardiac applications include Rb-82 or N-13 ammonia for myocardial perfusion imaging, and F-18 FDG for myocardial viability.
Following intravenous injection of positron-emitting radiopharmaceuticals and a variable amount of injection-to-imaging delay (called "the uptake period"), tomographic images are acquired. The positrons, once emitted from the nucleus, interact with electrons within a few millimeters in the tissue, resulting in the emission of 2 simultaneous high-energy photons, 180° apart from each other. Imaging of these high-energy photons is best achieved with multicrystal complete or partial ring detector systems, called "dedicated PET systems." Crystal materials used in these systems, such as bismuth germanate (BGO), lutetium oxyorthosilicate (LSO), and gadolinium orthosilicate (GSO), possess variable degrees of efficiency in absorbing these high-energy photons, converting them to light, and providing high spatial and temporal resolution. In addition, gamma camera SPECT systems, routinely used in nuclear medicine departments, can be equipped with coincidence circuit or high-energy 511 KeV collimators, providing a cost-effective means of imaging positron-emitting radiopharmaceuticals. In general, higher image resolution is more critical for oncologic PET imaging than for cardiac PET imaging. Therefore, relatively poor image resolution of gamma camera SPECT systems is not a significant limitation in cardiac PET imaging.
Myocardial metabolism imaging with PET identifies preserved metabolic activity in dysfunctional and hypoperfused myocardial regions. As such, assessment of myocardial viability is accomplished by comparing FDG uptake with the regional distribution of myocardial perfusion, determined either by PET or SPECT. There are 3 possible combinations of blood flow and metabolism, as discussed below:
- Normal blood flow and glucose utilization.
- Regionally increased F-18 FDG uptake relative to regional myocardial blood flow (perfusion-metabolism mismatch) suggests myocardial viability and predicts reversibility of contractile dysfunction following revascularization (Figure 3). Consideration should be given to the fact that such a mismatch does not always predict complete recovering of contractile function because scar and viable myocardium may coexist in such regions.
- Regionally reduced F-18 deoxyglucose uptake in proportion to regionally reduced myocardial perfusion (perfusion-metabolism match) suggests irreversibility of contractile dysfunction (ie, absence of myocardial viability) (Figure 4).
Whereas diabetes is an important risk factor in the development of coronary artery disease, and for morbidity and mortality in patients with coronary artery disease, diabetic patients pose a significant challenge in obtaining good-quality FDG-PET images. However, appropriate control of plasma glucose levels and the use of intravenous insulin, preferably using a glucose-insulin infusion pump, provides good image quality in most diabetic patients.
F-18 FDG PET has been shown to be accurate in the identification of viable myocardium. Tillisch et al 18 evaluated 17 patients with 73 dysfunctional myocardial segments; 67 of these regions were revascularized adequately. At 3-month follow-up, there was improvement of wall motion in 85% of segments with normal flow and glucose metabolism or with flow/metabolism mismatches. In contrast, only 8% of segments with flow/metabolism matched defects showed functional improvement. The positive predictive value of preserved F-18 FDG uptake in predicting functional recovery was 85%, and the negative predictive value was 92%. The predictive value of F-18 FDG PET is related to the extent of mismatches and the severity of the left ventricular dysfunction prior to revascularization. 18,19,32 In patients with a perfusion/metabolism mismatch involving only one myocardial segment, the left ventricular ejection fraction remained unchanged. In patients with mismatches involving ≥2 myocardial segments, left ventricular ejection fraction increased significantly. 18,19 The predictive accuracy of F-18 FDG cardiac PET is also related to the severity of the left ventricular dysfunction prior to revascularization. 32 It was higher in patients with severe (as opposed to mild) hypokinesis. The predictive value was highest in segments with akinesis.
A number of studies have evaluated the prognostic value of F-18 FDG cardiac PET with regard to future cardiac events. 20-23,33 In patients with prior myocardial infarction but stable coronary artery disease, FDG uptake was shown to be the most important independent predictor of future cardiac events. 22 Other studies have shown flow-metabolism mismatch as an indicator of cardiac death. 20,33 Di Carli et al 23 have shown a correlation between the extent of mismatches and subsequent improvement in cardiac failure symptoms.
Several studies have established a strong relationship between blood-flow metabolism mismatches and subsequent development of myocardial infarction or cardiac death. In these long-term studies, the combined data reveals that when there was presence of blood flow/metabolism mismatch, there was an average of 24% incidence of cardiac death in 1 to 2 years. 34 When mismatch was absent, this rate dropped to 10% during the same period. These results make a strong argument for the value of blood flow/metabolism mismatch in prognostication as well as in the selection of patients likely to benefit from coronary artery bypass surgery. In the patients selected on the basis of blood flow/metabolism mismatch, 1-year and 5-year survival is reported to be as high as 70% to 80%. In contrast, when patients were managed conservatively despite the mismatch, the survival was only 40% to 50%. In addition, relief of ischemia, as documented by preoperative flow/metabolism mismatch, improves symptoms of congestive heart failure and improves quality of life. However, 1 investigation reported similar 5-year survival rates achieved in patients with ischemic cardiomyopathy, irrespective of presence or absence of flow/metabolism mismatch, 35 questioning the value of cardiac FDG-PET in this setting.
For the differentiation of scarred versus hibernating myocardium, the patient groups studied in most reported series include those with chronic coronary artery disease. It is not clear that the concept of flow/metabolism mismatch is as accurate in the setting of acute myocardial infarction. However, some publications emphasize the clinical use of F-18 FDG cardiac PET in early postinfarction patients. 36,37 With respect to the perioperative course of patients following coronary artery bypass surgery, 2 investigations reviewed outcomes based on conventional selection criteria with and without the blood flow/ metabolism mismatch information. 38,39 Among 317 patients with coronary ischemia and left ventricular dysfunction, conventional criteria (left ventricular ejection fraction >20%, suitable coronary anatomy for bypass grafting and lack of significant comorbidities) were applied in the subgroup of 122 patients undergoing coronary artery bypass surgery. In the second subgroup of 195 patients, the presence of a blood flow/metabolism mismatch was considered a prerequisite. In this subgroup, 145 patients showed a mismatch pattern and subsequently underwent bypass grafting. This subgroup showed fewer pre-operative complications, including the need for an intra-aortic balloon pump and inotropic support. The 30-day mortality rate in the latter subgroup was only 0.01%, in sharp contrast to the rate of 17.2% in the conventional criteria subgroup.
Dobutamine stress echocardiography
Dobutamine is a synthetic catecholamine, capable of augmenting myocardial contractility at low doses and causing increased heart rate and peripheral vasodilatation at higher doses. Dobutamine-induced increases in heart rate, left ventricular ejection fraction, and mean arterial pressure are accompanied by a decrease in pulmonary capillary wedge pressure and better contractility in the regions containing viable, jeopardized myocardium. In these regions, there is a biphasic response-low doses of dobutamine resulting in better contractility but higher doses resulting in impaired wall motion (Figure 5). In the evaluation of viable myocardium in patients undergoing intravenous thrombolysis following acute anterior wall myocardial infarction, Pierard et al 40 compared echocardiography before and during dobutamine infusion with PET. Echocardiography and PET were repeated at a mean interval of 9 months. In both initial and follow-up studies, concordant interpretation of the 2 techniques was found in 79% of the affected segments. They concluded that echocardiography during dobutamine infusion is a promising method to unmask viable myocardium in acute myocardial infarction. Early recovery of perfusion in the area at risk was associated with a good functional outcome, whereas a high glucose-to-perfusion ratio suggested jeopardized myocardium that frequently lost viability.
Contractile reserve can be assessed relatively easily using standard echocardiographic techniques. In 32 published studies correlating postrevascularization functional recovery with contractile reserve demonstrated following low-dose dobutamine infusion, the mean sensitivity and specificity were 82% and 79%, respectively. 41 Due to various factors, including variations in dobutamine protocols, differences in the patient selection criteria and the length of follow-up, there is a wide reported range of predictive accuracy for recovery of contractile dysfunction from 77% to 95%. 42 A comparison analysis showed higher specificity but a lower sensitivity of stress echocardiography for detection of reversible contractile dysfunction. 41 Of note, the reported "specificity" (no evidence of contractile reserve and no subsequent evidence of functional recovery after revascularization) was higher than that for either delayed thallium-201 uptake or F-18 FDG uptake. However, this is not surprising, since the end point of all of these studies was functional recovery, and low-dose dobutamine echocardiography specifically evaluates functional improvement. It is yet to be determined if other important parameters, such as the prevention of ventricular remodeling and the prevention of life-threatening arrhythmias, are better predicted by radionuclide or echocardiographic techniques. In addition, recent enhancements to echocardiography, such as echo contrast, harmonic imaging, and power Doppler imaging, all of which enhance endocardial border definition, may improve echocardiography's diagnostic accuracy in detecting contractile reserve.
Role of MRI in the assessment of myocardial viability
The spatial resolution of MRI, particularly with regard to differentiating subendocardial versus transmural myocardial abnormalities, is far superior to SPECT, PET, and echocardiography. MRI techniques have been developed to detect myocardial deformity or torque during ventricular contraction. Whereas regions of viable myocardium will demonstrate deformity of "tagged" magnetic lines during systole, myocardial scars will show no such deformity. A more recent and technically less demanding MRI method to assess myocardial viability is the phenomenon of "delayed hyperenhancement." Following MRI contrast injection, T1- and T2-weighted images will demonstrate decreased signal intensity both in regions of myocardial scar and also in areas of resting ischemia (ie, hibernating myocardium). However, in repeat, delayed images, myocardial scars will accumulate contrast (delayed hyperenhancement), whereas resting ischemia will not (Figure 6).
It has been shown that if <25% of the thickness of a myocardial wall shows delayed hyperenhancement, wall motion will improve following revascularization. In a study of 31 patients with ischemic heart failure, Klein et al 44 compared MRI and F-18 FDG PET. They concluded that MRI hyperenhancement as a marker of myocardial scar closely agreed with PET data. However, MRI seemed to identify scar tissue more frequently than did PET, reflecting the higher spatial resolution. In a study of 35 patients with chronic coronary artery disease, myocardial infarction, and regional akinesia or dyskinesia, Baer et al 45 compared rest/dobutamine MRI and FDG-PET and found that dobutamine-induced wall thickening was a better predictor of residual metabolic activity than was end-diastolic wall thickness at rest. However, when viability was defined as the presence of at least one MRI parameter, sensitivity improved without a major decrease of specificity or positive predictive accuracy. In a recent study, Kuhl et al 46 compared FDG-PET, Tc-99m tetrofosmin SPECT, and contrast-enhanced MRI for assessment of nonviable myocardium in 26 patients with chronic coronary artery disease and left ventricular dysfunction. Segmental glucose uptake on PET was inversely correlated (r = -0.86, P <0.001) to segmental extent of hyperenhancement (SEH) on MRI. Using SEH threshold of 37%, sensitivity and specificity of contrast-enhanced MRI to detect nonviable myocardium as defined by PET were 96% and 84%, respectively.
Conclusion
A variety of noninvasive imaging methods have emerged to assess myocardial viability and to help predict which patients will recover ventricular function following revascularization. However, in addition to recovery of function, we must also consider the potential for these methods to predict which patients may benefit from revascularization or other interventions to prevent ventricular remodeling and life-threatening arrhythmias. Each of the methods described in this review have particular technical and clinical advantages and disadvantages. Therefore, we await further reports of technical advancements and clinical validation to determine which one(s) of these will emerge as the diagnostic and prognostic frontrunner(s).