Intracranial vascular imaging: Pearls and pitfalls
Images
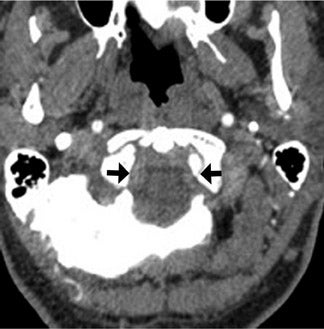
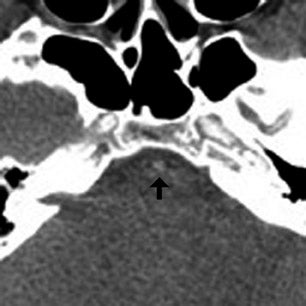
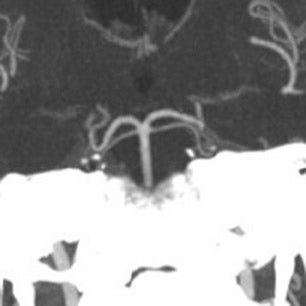
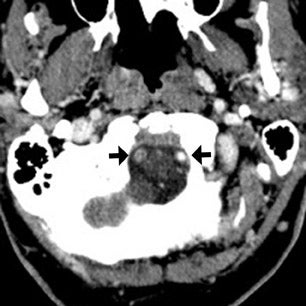
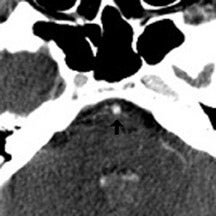
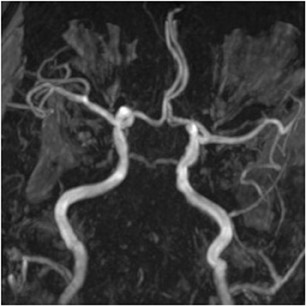
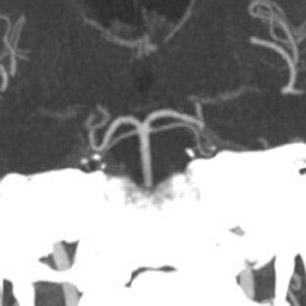
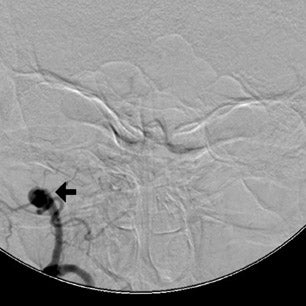
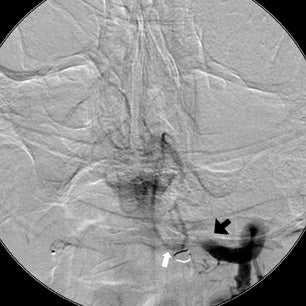
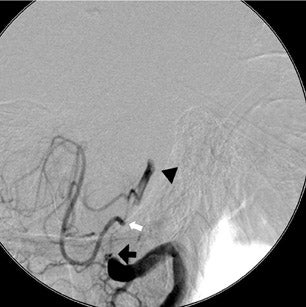
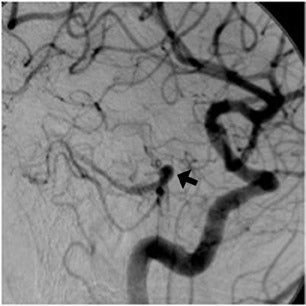
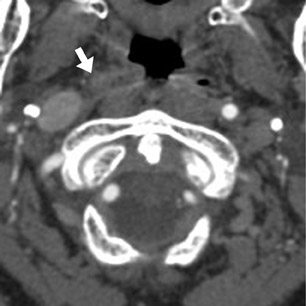
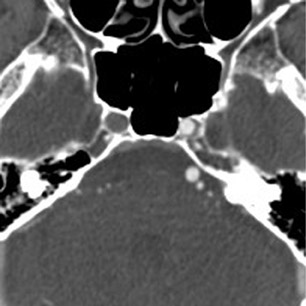
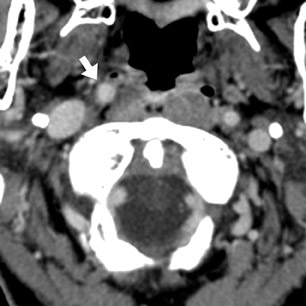
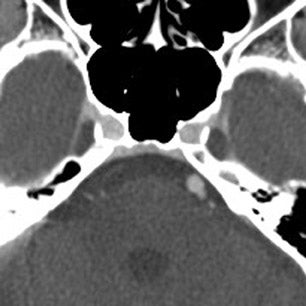
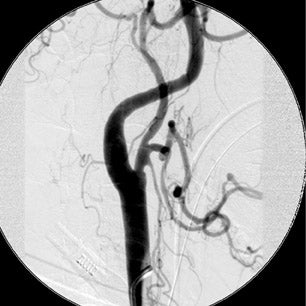
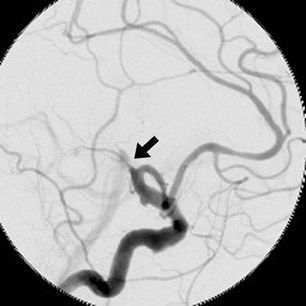
Dr. Kim is Assistant Professor of Clinical Radiology, Neuroradiology Section, San Francisco General Hospital, University of California, San Francisco, CA; and Dr. Wintermark is Chief of the Division of Neuroradiology, University of Virginia Health System, Charlottesville, VA.
There has been increasing reliance on computed tomography angiography (CTA) and magnetic resonance angiography (MRA) for evaluation of the intracranial vasculature in patients suspected of acute stroke. Digital subtraction angiography (DSA), while traditionally the gold standard, is invasive and associated with potential complications. Further, DSA is not readily accessible any time day or night. In the acute stroke setting, DSA is typically reserved for patients undergoing therapeutic intervention with intra-arterial thrombolysis or embolectomy.
The purpose of this article is to compare the diagnostic accuracy of CTA, MRA and DSA for evaluation of the intracranial circulation in patients with stroke. We will discuss the technical parameters and potential artifacts that must be understood so that an accurate interpretation of each modality can be made. We will structure our discussion around an illustrative clinical case of a patient who underwent CTA, MRA and DSA within a 24 hour time frame.
Clinical case
A 62-year-old man presented to the emergency room with acute onset diplopia, diffuse weakness and vomiting. Given the concern for posterior-circulation ischemia, a CT/CTA was requested and performed within the first hour of symptom onset (Figure 1). Our standard CT stroke protocol included 64-slice CTA from the base of the heart through the cerebral vertex, as well as delayed contrast-enhanced head CT obtained approximately 1 min after CTA (without the need for additional contrast material because contrast has already been injected for CTA). In this patient, the initial CTA demonstrated lack of opacification of the bilateral distal vertebral arteries and proximal basilar artery, while the delayed contrast-enhanced images demonstrated normal opacification of the left distal vertebral artery and proximal basilar artery.
Based on the clinical and imaging findings, the patient received IV tissue plasminogen activator (tPA) and underwent 3-dimensional time of flight (TOF) intracranial MRA approximately 4 hours following CTA to reassess his vasculature after thrombolysis (Figure 2). The MRA demonstrated absence of flow-related enhancement in the bilateral distal vertebral and entire basilar artery, which wasdiscrepant with the initial CTA, especially considering that there was no change in the patient’s clinical examination and no evidence of neurologic deterioration between initial CTA and follow-up MRA.
The patient then underwent DSA the following day, approximately 24 hours after the initial CTA, to reevaluate his vasculature (Figure 3). Again, there was no neurologic deterioration at the time of the study. DSA demonstrated occlusion of the right vertebral artery, occlusion of the left vertebral artery proximal to the posterior inferior cerebellar artery (PICA), reconstitution of the vertebrobasilar confluence distal to the PICA, and occlusion of the remainder of the basilar artery with filling of the basilar tip via the posterior communicating artery.
The important point about this case is that CTA, contrast-enhanced CT, MRA and DSA obtained within 24 hours of each other, and without any change in the patient’s clinical status, demonstrated very different findings. Both MRA and DSA appeared to demonstrate occlusion of the basilar artery, as well as occlusion of the bilateral distal vertebral arteries. However, most of the basilar artery appeared patenton CTA, which showed occlusion limited to the bilateral distal vertebral arteries and only the most proximal aspect of the basilar artery. Delayed contrast-enhanced CT, in turn, appeared to demonstrate patency of the distal left vertebral and entire basilar artery.
One explanation for the discrepancy is that the patient’s basilar artery thrombosed between the time of CTA and MRA/DSA. However, the absence of any neurological changes between examinations, as would be expected with a typically devastating basilar artery occlusion, weighs against this possibility. A more likely explanation for the radiologic discrepancies lies in each imaging modality’s way of capturing and assessing vascular flow.
Accurately reading intracranial CTA, MRA and DSA to avoid pitfalls of interpretation requires an understanding of the technical parameters and potential artifacts underlying each modality.
CT angiography
The steady introduction of CT scanners with ever-increasing numbers of detector rows has enabled greater craniocaudal coverage at increasingly faster scan times and better resolution. Current state-of-the-art clinical stroke CTA imaging relies on 64-slice CT,which can scan from the aortic arch through the intracranial vessels in a matter of 3 to 4 sec at submillimeter isotropic resolution. Acquisition time is significantly shorter than single-slice CTA and 4-slice CTA. This also means that less contrast material is used and that contrast material has less time to circulate within the vessels on 64-slice CTA than on single- or 4-slice CTA.
The entire duration of 64-slice CTA, from start of contrast injection to conclusion of scanning, is approximately 20 to 25 sec. Because image acquisition only requires 3 to 4 sec, most of this time is due to the delay between when contrast is injected and when scanning is begun. This 15 to 20 sec delay is necessary to ensure optimal opacification of the cervical and intracranial vessels, and the precise amount of delay can be determined by either timing bolus or by a bolus-tracking technique.1–3We use the former in our stroke CTA protocol.
The contrast-enhanced head CT that is obtained after 64-slice CTA in our stroke protocol utilizes contrast material that was previously injected for the CTA, and does not require that any additional contrast be used. Contrast-enhanced CT is obtained approximately 60 sec after CTA, which allows additional time for contrast material to circulate within the body as compared with 64-slice CTA. In our clinical example, CTA showed lack of opacification of the distal left vertebral and proximal basilar artery, both of which appeared opacified on delayed contrast-enhanced CT; only the distal right vertebral artery appeared occluded on both CTA and delayed CT. It is likely that slow or low flow through the distal left and proximal basilar artery caused them to appear occluded on fast 64-slice CTA, while delayed contrast-enhanced CT allowed for greater contrast circulation to establish patency. Figure 4 illustrates a similar case in a different patient.
These cases show that areas of significantly delayed blood flow, secondary to proximal vessel stenosis or distal thrombosis, may be imaged by rapid 64-slice CTA before adequate contrast opacification has occurred. The scan can “outrun” the contrast material circulation in areas of slow flow, which are able to opacify by the time delayed contrast-enhanced CT is obtained. Arterial pseudo-occlusion is a type of flow artifact incurred by fast CT scanning.
CTA is reported to have high sensitivity and specificity for detection of intracranial stenoses and occlusions. Two recent studies indicated high sensitivity and specificity of CTA for detecting moderate/severe stenosis (>97%); for occlusion, the sensitivity and specificity were even higher (approaching 100%).4,5 The positive predictive value of CTA for diagnosing intracranial occlusion was 100% in these studies. Both of these studies evaluated images obtained on earlier-generation CT scanners (single- and 4-slice in one study, 8- and 16 slice in the other). The longer image acquisition time in these studies may help to explain the lack of any false-positive findings of occlusion. However, with increased availability and adoption of very fast CT scanning with 64-slice scanners, the possibility of image acquisition outrunning the contrast bolus in cases of slow or altered flow should be considered to prevent overestimating and misdiagnosing occlusion.
MR angiography
Published literature on 3-dimensional intracranial TOF MRA shows equivalent, to slightly lower, sensitivity of MRA as compared with CTA for diagnosis of steno-occlusive disease. Sensitivity ranges between 70% to 100% for detecting moderate/severe stenosis, and between 87% to 100% for occlusion; specificity is close to 100% for both.4,6,7
However, MRA has several important limitations. Unlike CTA and DSA, which employ a contrast agent to directly image the blood within a vessel, TOF MRA relies on the magnetization of spins flowing into an imaging slice and is prone to certain flow artifacts.
TOF MRA uses a gradient echo sequence and a rapid succession of radio frequency (RF) pulses to saturate and suppress signal from stationary background tissue. Blood situated outside the imaging plane remains relatively unaffected by the pulses and flows into the imaging plane with magnetization intact, generating bright MR signal (flow-related enhancement). As such, ideal TOF imaging requires that background tissue be completely suppressed, and that inflowing blood be completely unsaturated to generate maximal signal. This is not always achievable in practice.
Background may not be entirely suppressed with tissues that have short T1 relaxation times, such as fat, hematoma or thrombus. These tissues quickly recover their longitudinal magnetization despite the rapid succession of RF pulses, and are able to generate bright MR signal that may interfere with image interpretation and obscure arteries.
Flowing blood may also experience saturation effects by RF pulses. Slow flowing blood spends more time within a given imaging volume, making it susceptible to saturation by RF pulses. Areas of slow flow due to proximal or distal stenosis/thrombosis may have signal loss and appear occluded.
Areas of turbulent blood flow near a stenosis can also appear occluded due to signal loss. The observation of signal loss due to spin dephasing at stenoses with turbulent flow has been described in both phantom models and clinical studies.6,8,9
Finally, retrograde flow through collateral vessels (in cases of vessel occlusion) may not be depicted. TOF imaging frequently uses a saturation band to suppress all flow-related enhancement opposite in direction to arterial flow, which typically eliminates unwanted venous signal but may also have the unintended consequence of suppressing retrograde collateral flow. Knowledge of these potential pitfalls is critical for accurate interpretation of MRA. This is especially relevant to acute stroke imaging, as patients with cerebral thromboembolic disease are more likely to have alterations in flow dynamics with slow flow, turbulent flow and retrograde flow.
Given the potential for artifactual signal loss with TOF MRA in cases of altered flow, it is not surprising that MRA has a high false-positive rate for detection of intracranial stenosis and occlusion. Bash et al. compared CTA, MRA and DSA findings in patients suspected of acute cerebrovascular events, and found that MRA had positive predictive values of 65% for stenosis and 59% for occlusion, compared with 93% and 100% for CTA.4
In our clinical example, the basilar artery appeared patent on CTA but occluded on MRA obtained 4 hours later, following thrombolysis. It is likely that the distal vertebral artery occlusion resulted in slow flow through the basilar artery and/or retrograde flow through posterior communicating arteries, causing artifactual signal loss on MRA. The complete absence of any clinical deterioration between CTAand MRA makes basilar artery occlusion unlikely. MRA in this case was falsely positive for occlusion, a conclusion that can be reached given an understanding of flow artifacts common to TOF MRA.
Digital subtraction angiography
DSA is traditionally considered the gold standard for assessment of the intracranial vasculature, and is the modality with which CTA and MRA are compared. In our clinical case, DSA showed occlusion of most of the basilar artery, with only minimal opacification of the vertebrobasilar confluence and basilar tip (the latter via posterior communicating arteries on carotid injection). However, CTA and delayed contrast-enhanced CT had previously shown good opacification of the basilar artery and there had been no interval change in the patient’s clinical status between CT and DSA.
Given the CT findings and stable clinical examination, it is likely that DSA reflected a false-positive finding of basilar artery occlusion. Bash et al. reported cases of false-positive DSA findings of occlusion in 6 of 28 patients (21%) who presented with symptoms of acute stroke and underwent CTA, MRA and DSA.4 All 6 cases involved the posterior circulation and occurred in very slow- or low-flow states distal to a significant stenosis, causing the vessel to appear occluded on DSA but stenotic (and otherwise opacified) on CTA. MRA was also falsely positive for occlusion in 4 of these 6 cases. The conclusion of a false-positive DSA finding was reached by consensus re-evaluation of the data in all 6 cases.
The likely explanation for the discrepancy between CTA and DSA findings in our case, as well as the reported cases, lies in differences in image acquisition time. Angiographic runs are typically obtained over 5 sec (at a 4 frames-per-second film rate) and capture a single intracranial circulation cycle. A single intracranial circulation cycle, from opacification of the carotid siphon to maximal opacification of the cortical veins, lasts approximately 4 to 6 sec.10,11 While 64-slice CTA is extremely fast, time from contrast injection to scan completion is 20 to 25 sec and still leaves more time for contrast material to circulate through areas of severe stenosis than on DSA. Delayed contrast-enhanced CT obtained 60 sec after CTA allows even more time for contrast material circulation to opacify patent segments of the vessel lumen oneither side of stenotic or occluded areas.
Conclusion
Our clinical case and literature review highlights several important points about intracranial vascular imaging with CTA, MRA and DSA. CTA and MRA have fairly high sensitivity/specificity for detection of intracranial stenoses and occlusions, when using DSA as the gold standard. However, flow-related artifacts can create the appearance of vasculature occlusion in each modality. The mechanism by which this occurs is different.
In TOF MRA, signal loss can occur due to slow, turbulent or retrograde flow; this is well known and described extensively in the literature. In contrast, flow-related artifacts are not typically associated with CTA or DSA. As acquisition times become increasingly shorter with greater–detector-row CT scanners, the possibility of image acquisition outrunning the contrast bolus and producing pseudo-occlusion must be considered. Knowledge of these potential pitfalls is useful and may prevent inaccurate interpretation of imaging findings caused by dynamic alterations to blood flow in patients with cerebrovascular disease.
REFERENCES
- Bae KT. Test-bolus versus bolus-tracking techniques for CT angiographic timing. Radiology. 2005;236:369-370; author reply 370.
- Cademartiri F, Nieman K, van der Lugt A, et al. Intravenous contrast material administration at 16-detector row helical CT coronary angiography: Test bolus versus bolus-tracking technique. Radiology. 2004;233:817-823.
- Hallett RL, Fleischmann D. Tools of the trade for CTA: MDCT scanners and contrast medium injection protocols. Tech Vasc Interv Radiol. 2006; 9:134-142.
- Bash S, Villablanca JP, Jahan R, et al. Intracranial vascular stenosis and occlusive disease: Evaluation with CT angiography, MR angiography, and digital subtraction angiography. AJNR Am J Neuroradiol. 2005;26:1012-1021.
- Nguyen-Huynh MN, Wintermark M, English J, et al. How accurate is CT angiography in evaluating intracranial atherosclerotic disease? Stroke. 2008; 39:1184-1188.
- Furst G, Hofer M, Steinmetz H, et al. Intracranial stenoocclusive disease: MR angiography with magnetization transfer and variable flip angle. AJNR Am J Neuroradiol. 1996;17:1749-1757.
- Korogi Y,Takahashi M, Nakagawa T, et al. Intracranial vascular stenosis and occlusion: MR angiographic findings. AJNR Am J Neuroradiol. 1997;18:135-143.
- Bradley WG, Jr., Waluch V, Lai KS, et al. The appearance of rapidly flowing blood on magnetic resonance images. AJR Am J Roentgenol. 1984; 143:1167-1174.
- Evans AJ, Blinder RA, Herfkens RJ, et al. Effects of turbulence on signal intensity in gradient echo images. Invest Radiol. 1988;23:512-518.
- Greitz T.A radiologic study of the brain circulation by rapid serial angiography of the carotid artery. Acta Radiol Suppl. 1956:1-123.
- Milburn JM, Moran CJ, Cross DT, 3rd, et al. Effect of intraarterial papaverine on cerebral circulation time. AJNR Am J Neuroradiol. 1997;18: 1081-1085.