Health effects of ionizing radiation from diagnostic CT imaging: Consideration of alternative imaging strategies
Images
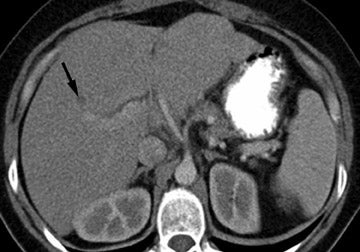
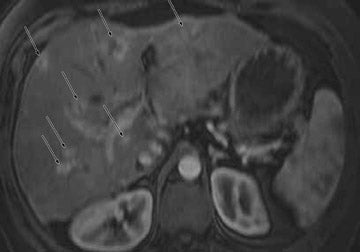
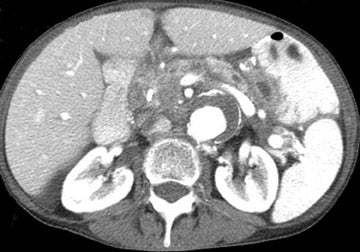
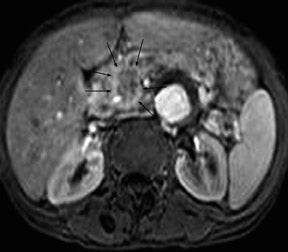
Dr. Martin is a Professor and the Director of MRI, Department of Radiology, Emory University School of Medicine, Atlanta, GA. Dr. Semelka is a Professor of Radiology, the Director of MRI, and the Vice Chair of Clinical Research, Department of Radiology, University of North Carolina at Chapel Hill, Chapel Hill, NC.
It is scientifically accepted that the ionizing radiation generated by medical diagnostic X-ray machines induces neoplasms through damage and alterations to cellular DNA, and that this risk is related to the dose administered. Computed tomography (CT) examinations account for the largest population radiation dose from medical diagnostic studies, with more than 60 million CT examinations performed per year in the United States that represent an estimated 70% of all medical X-ray exposure. 1 Consensus on risk estimates are more challenging because of the low doses administered, the stochastic nature of radiation effects, and the time course between cause and effect. 2
Only a small fraction of X-ray photons will lead to DNA changes, and only a fraction of those changes will lead to cancer formation. The time between a DNA-altering event and progression to cancer formation may require periods as long as 10 to 20 years in most cases. Statistical analysis requires large numbers of people to be studied to yield useful data. The atomic bomb survivors represent the largest and most studied population. Although atomic bomb radiation produces gamma rays, and medical exposure produces X-rays, the carcinogenic potential is considered to be equivalent for an equivalent dose. 2 Even so, the fact that approximately 42% of the U.S. population die from cancer-related causes makes statistical analysis difficult. 2
Several national and international organizations have been given the task of producing periodic reviews of the cumulative data on human low-dose population exposure to determine health risks. The seventh National Academy of Science report on Biological Effects of Ionizing Radiation (BEIR VII) 2 is the most recent update from a highly respected organization. The findings from BEIR VII are summarized here and reviewed in the context of other studies, with a view to discuss how radiologists may consider approaches to imaging that may moderate radiation exposure to our patients. Given that the typical CT studies resulting in the highest doses involve studies of the torso (chest, abdomen, and pelvis), the potential for supplementing or replacing CT examinations using magnetic resonance imaging (MRI) will be discussed regarding the strengths and limitations of this strategy.
Health risks from exposure to low levels of ionizing radiation
The National Academies' National Research Council has produced a series of reports on BEIR, and the seventh report of this series has been released. 2 This report represents an exhaustive evaluation of the literature and updates prior health-risk estimates using the latest epidemiological data. The report specifically examines low-dose, low linear energy transfer (LET) ionizing radiation, as is produced by CT or plain-film imaging systems. 3-6
In this study, low doses are defined as <100 mSv, while the typical CT examination of the abdomen and pelvis will result in an exposure of between 10 and 20 mSv. 2 In contrast, the exposure from a chest radiograph is approximately 0.1 mSv, 2 and the estimated dose for a body scan with a combined positron emission tomography (PET) and computed tomography (CT) scanner is 25 mSv. 7 Although in the past it has been argued that the biological damage and health risks from ionizing radiation may not have a linear relationship with dose, the BEIR committee concluded that the biological and biophysical data supports a linear no-threshold (LNT) risk estimate model that indicates that even the smallest dose of low-level ionizing radiation has the potential to cause an increase in health risks to humans. 2
Biological effects of ionizing radiation may be mediated by breaking biomolecular bonds of cellular DNA. In somatic cells (non-germ cells), DNA damage may lead to initiation or promotion of tumor formation by destroying important regulatory mechanisms encoded in the DNA that are used for control of cell division and differentiation. 8-10 Leukemias are the types of tumors that have been most studied epidemiologically in relation to ionizing radiation exposure in humans, and these studies have primarily concentrated on mortality risks. The new epidemiological information and approaches used in BEIR VII include estimation of risks for development of leukemias and of solid tumors. 2 In addition, risk estimates for both mortality and incidence have been determined. In germ cells, DNA damage could yield growth and developmental defects passed on to offspring. The BEIR VII Report examines data to attempt assessment of such risk.
Risk estimates at low doses
Estimates have been based on models other than the LNT model, and some investigators believe that there is evidence to support the conclusion that low doses of ionizing radiation may have a cancer risk in excess of what is predicted by a linear model. 11-14 Similarly, others have argued that low doses of radiation may have no effect, or may even be beneficial by inducing the production of DNA repair mechanisms within cells exposed to a low radiation dose. 15-18 The BEIR VII Report has taken into account a comprehensive examination of the literature on this subject and has concluded that there exists a "preponderance of scientific" experimental and epidemiological data that predominantly supports the use of an LNT model to predict cancer risk. 2
The main source of epidemiological data has been the study of Japanese atomic bomb survivors from Hiroshima and Nagasaki. Other data was derived from studies of medically exposed individuals and nuclear workers. BEIR VII specifically examined the incidence and mortality related to leukemia and to 11 different solid tumors, including breast and thyroid carcinomas. Since the last BEIR Report issued in 1990, an additional 15 years of follow-up of exposed individuals has been evaluated and an updated dosimetry metric has been implemented. New data included the evaluation of individuals exposed to radiation for medical reasons. The individuals in the lowest-dose group of atomic bomb survivors who showed a significant rise in cancer incidence and mortality received total body exposure with organ equivalent doses in the range of 5 to 100 mSv (mean equivalent dose 29 mSv) and 5 to 125 mSv (mean equivalent dose 34 mSv), respectively. Typical equivalent doses in directly irradiated organs are in the range of up to 20 to 30 mSv for a single, routine, adult abdominal CT examination. 14,19 Therefore, risk estimates for radiation exposure from CT examinations can be directly related to the findings among atomic bomb survivors, without a need for extrapolation.
In summary, the BEIR VII Report indicates that in a population with the demographic distribution of the U.S. population, a single dose of 100 mSv is associated with an estimated lifetime attributable risk (LAR) for developing a solid cancer or leukemia of 1 in 100. The overall risk of developing a solid cancer or leukemia from causes other than ionizing radiation in this same population would be 42 in 100. 2 A single dose of 10 mSv is associated with a LAR of 1 in 1000 for developing a cancer. Given that an estimated 60 million CT examinations are performed each year, 20 using the assumptions of 1 whole-body 10 mSv dose per examination per patient, this would predict an estimated LAR of 60,000 solid cancers and leukemia, every year. In comparison, the estimated risk of serious complications and death from receiving iodinated intravenous CT contrast is approximately 1 in 400,000, 21 which is lower than the LAR from a single 10 mSv dose. We recognize that these estimates make simplistic assumptions regarding population exposure, but they are useful in order to appreciate a discussion of the magnitude of current population medical exposure levels associated with CT studies.
Non-neoplastic health risks
Ionizing radiation has been associated with the development of other, non-neoplastic disorders, including cardiovascular disease. 22-24 However, these disorders will develop with doses >100 mSV. The BEIR VII Report indicated that the data was insufficient to quantify the risk for nonneoplastic disorders that are associated with low-dose ionizing radiation <100 mSv. Similarly, the risk of low-dose radiation for genetically transferred defects could not be shown. However, it is further noted that the most likely outcome of a fetus suffering from a radiation-induced DNA defect would be a spontaneous abortion, but this has not been studied.
Other risk-estimate reports
Several other recent publications have attempted to estimate risks in specific risk groups. Of particular note, the 2005 report from the Department of Health and Human Services listed ionizing radiation as a known human carcinogen in the Eleventh Report on Carcinogens. 25 Major international scientific organizations that are responsible for evaluating health risks of ionizing radiation and for establishing policies include the International Commission on Radiological Protection (ICRP) 26 and the United Nations Scientific Committee on the Effects of Atomic Radiation (UNSCEAR). 27 These organizations have come to the same conclusions as the BEIR VII Report with regard to using an LNT model for dose-risk estimates, thus concluding that there is no entirely safe dose of ionizing radiation. The U.S. Food and Drug Administration estimates that a CT examination with an effective dose of 10 mSv (eg, 1 CT of the abdomen) may be associated with the increase in the possibility of fatal cancer of approximately 1 chance in 2000. 28,29 Furthermore, published frequency data masks the number of times a single patient receives multiple CT examinations 29 or receives multiphase CT scanning. Radiation risk may be related to the cumulative dose of medical radiation, and there is limited data to track the number of repeated CT scans or the number of multiphase scans that individuals are receiving, although studies have shown that up to half of the patients receiving a CT scan will have multiple scans, and that the average number of scans for a given indication is 2, yielding an average dose that may exceed 40 mSv. 20,30,31 Other estimates indicate that 30% of all individuals having a CT will have a total of at least 3 examinations. 29 The pediatric population is more vulnerable to the risks of CT radiation, and an estimate of the lifetime cancer mortality risk attributable to the radiation exposure from a single abdominal CT examination in a 1-year-old child is approximately 1 in 550. 19 Additional concern derives from the potential for use of suboptimal CT technique and excessive exposures in smaller pediatric patients: at the same technique factors, pediatric doses are substantially higher. 3,32 It has been estimated that 600,000 abdominal and head CT examinations were performed annually in children under the age of 15 years with an estimated LAR of 500 deaths from cancer, and that the number of CT studies is growing rapidly. 19
Implications for standards of patient care
Informed patient consent
Currently, most institutions require a signed consent form from patients undergoing a CT scan. Typically, the risks described to the patient are related to the intravenous iodinated contrast utilized for a contrast-enhanced CT examination. However, the need to discuss the radiation risk of CT examinations is not mandatory and is rarely a component of the standard consent form. In addition, informed consent requires that the radiologist, or a representative, describe any reasonable alternatives to having the CT examination; this is also not commonly done. A recent study in health policy and practice by Lee et al 33 considered adult patients with mild-to-moderate abdominal/pelvic or flank pain who underwent CT examination during a 2-week period in the emergency department of a U.S. academic medical center and who were subsequently surveyed after acquisition of the CT scan. Only 7% of patients stated that they were informed about the risks and benefits of a CT scan. More importantly, only 3% of patients reported that they were informed about the increased lifetime cancer risk associated with CT.
Informed practitioner
Evidence suggests that referring emergency department physicians are largely unaware that there are potentially harmful effects from CT radiation exposure, 33 with only 9% aware of increased cancer risk. In fact, data show that radiologists performing the CT examination consider the radiation exposure of limited concern, with only 47% of radiologists familiar with the increased risk of cancer associated with the CT examination. 33 Even many radiologists are unaware of the dose of radiation delivered to the patient during the CT examination. 33 Another recent study from a large hospital in the United Kingdom used a simple questionnaire to assess the knowledge of primary care and specialist physicians on radiation doses and risks. 34 Results of this study showed an urgent need to improve physicians' understanding of radiation exposure. Only 27% of physicians and only 57% of radiologists and radiology-related subspecialists achieved a test score >45%.
In addition, the CT imaging protocol used is entirely at the discretion of the radiologist or CT technologist. If meticulous care is not employed, it is technically feasible to scan a patient using multipass techniques for the purpose of achieving multiple phases of images before and after the administration of iodinated contrast and to use tube current settings that could radiate a patient with tube current levels that are set up to maximum levels- far in excess of the minimum required to achieve a diagnostic examination. Details of imaging strategies must also include the consideration of the use of slice profiles, since the settings of slice thickness and table pitch (the speed with which the patient passes through the scanner) also have direct effects on radiation exposure dose. For the purpose of dose minimization, these parameters-and the consideration of the pathology and organ systems being evaluated-must be considered. 3,4,32,35,36 In addition, imaging requirements and risks will differ according to the size and age of the patients. The use of faster CT scanners has promoted scanning beyond the indicated field of view. For example, CT technologists may regularly perform an abdominal examination to include most of the pelvis, even though the pelvic images were not requested and the additional pelvic slices will increase the total radiation dose. 37 Currently, there are no industry, federal governmental, or medical regulatory bodies that have control over these CT scan parameters or practice patterns, and a wide range of methodologies are applied at different centers.
Imaging alternatives
In most cases, ultrasound (US) and MRI represent safer alternatives to CT for abdominal and pelvic imaging. Additionally, MRI is useful and may be the preferred alternative for assessing certain diseases of the thorax and for brain and spine imaging. Limitations of MRI include its high cost, limited availability, contraindications, and the complexity associated with operating the MR machine.
MRI has been considered an expensive diagnostic test. However, the actual cost of MRI equipment, service, and site expenses has steadily diminished during the past 20 years by factors of approximately 65%, 55%, and 80%, respectively, while the fixed costs associated with a state-of-the-art CT have increased. The average MRI technical fee in 2004 fell by 70% compared with the average fee in 1985, and the trend in decreasing costs of MRI is expected to continue for the next 20 years. 38
Availability of MRI lags behind that of CT, thus limiting patient access to MRI. However, increased demand for MRI studies would place a burden on centers to increase the numbers of scanners. In addition, the technology continues to evolve, bringing forth developments that are designed to shorten the scan times, thus increasing throughput on a given MR system. Individual scans may not be approved because of reticent medical insurance providers. Documentation and continuing education of the insurance providers is required. State and federal governments have a vested interest in supporting actions that promote health maintenance, and they may have to establish insurance support guidelines.
The complexity of MR scanner operation may be largely resolved through engineering hardware and software solutions. For example, many steps performed by a technologist for an abdominal MRI study (which is considered one of the more complex techniques) are repetitive and lend themselves to automation. Solutions may result from automated patient and organ detection methods that assist the operater in patient positioning, field-of-view, surface coils, and optimized sequence parameter selections. This optimization procedure could be automatically individualized for each patient and propogated throughout the protocol to minimize the need for operator inputs. Combined with currently evolving coil hardware and software solutions, multiple-station examinations of multiple body parts, including whole-body imaging, has become feasible in relatively short examination times. Simplified user interfaces have lagged behind current software capabilities, but they represent an area of more dedicated development effort from vendors; increasingly simplified MR console software is beginning to appear on the market with user interfaces that borrow from more familiar personal computer operating systems.
Contraindications in MRI are mostly related to cardiac pacemaker devices, ferrous vascular aneurysm clips in the brain, and ferrous foreign bodies in the eye. The commonly used gadolinium chelates are a family of extremely safe contrast agents that can be used in patients who normally could not tolerate iodinated CT contrast because of allergy or renal insufficiency.
For most clinical problems for which CT is used to evaluate disease in the body, MRI would provide an acceptable or favorable alternative. In many cases, MRI would offer equal or superior imaging results with regard to diagnostic sensitivity and specificity. This has been shown most clearly in most disorders of intra-abdominal and pelvic origin. Evidence to demonstrate the potential for evaluation of intra-thoracic disorders, including pulmonary disease, is developing. The soft tissues of the chest, abdomen, and the pelvis represent the most radiosensitive tissues and are the focus of the following review of systems.
Chest
Although CT imaging remains the standard imaging test for evaluation of interstitial lung diseases, the ability to evaluate pulmonary nodules on contrast-enhanced 3-dimensional gradient-echo MRI has been shown to have sensitivity equal to that of CT on nodules as small as 6 mm. 39 In addition, MRI can provide similar or better imaging of the chest wall and mediastinal soft tissues with regard to the vasculature, lymph nodes, and masses. Cardiac MRI can also provide dynamic information about cardiac morphology and function and for the evaluation of myocardial scar tissue that results from myocardial infarction. 40,41 Breast MRI has been shown to be more sensitive than standard mammography, although its specificity is relatively lower. 42-46
With regard to routine chest imaging, current MR technology can be used in cancer work-up, including the staging or restaging of metastatic disease, and can be used routinely in patients with an intolerance to iodinated contrast. The authors frequently combine the chest examination with an abdominal study when assessing metastatic disease.
Evaluation of pulmonary emboli has become a common request for CT imaging of the chest, particularly in acute or emergency care settings. These patients are frequently young adults and are predictably more sensitive to radiation effects. The use of MR angiography for the evaluation of pulmonary emboli is a developing area, and its feasibility has been reported. 47-49 It is expected that MRI will become at least an alternative modality for selected patients, such as young patients or those who have renal insufficiency and are therefore at a relatively higher risk for iodinated contrast-induced renal failure.
Abdomen
The examination of the liver and pancreas using MRI has the support of a considerable body of literature to argue in favor of the use of MRI as a first-line imaging study for the evaluation of tumors and for the evaluation of diffuse diseases, such as liver fatty accumulation, hepatitis, cirrhosis, hemochromatosis, 50-55 pancreatitis, and pancreatic or bile duct disorders. 56 CT imaging of the liver may show good sensitivity, but MRI provides superior sensitivity and specificity. 50,52,53,57,58 The strength in MRI is the ability to provide more information regarding the exact pathology within the liver to differentiate between benign and malignant lesions. In addition, compared with CT, MRI is better able to evaluate inflammatory changes that are associated with hepatitis and cholangiohepatitis with greater sensitivity. MRI can combine tissue imaging sequences with MR cholangiopancreatography techniques to more comprehensively evaluate disorders related to the intra- and extrahepatic bile ducts and gallbladder. 57,59 Imaging of the pancreas is another relative strength of MRI, 56,60-67 with its greater sensitivity and specificity for the evaluation of mild or severe changes of pancreatitis 67 and its demonstrated capacity for the detection of small, and potentially more treatable, pancreatic cancers. 66 In addition, it has been argued that sensitivity and specificity for small tumors is superior on MRI. Figure 1 shows characteristic examples of tumors of the liver or pancreas that are shown with greater conspicuity and specificity on MRI than on contrast-enhanced CT. The adrenal glands 68-70 and kidneys may be similarly evaluated for tumors on CT and MRI, although CT can be used to detect even 1 mm of renal calculi, and MRI is generally insensitive to the presence of nonobstructing calculi. However, MRI has been shown to have the potential to replace CT and intravenous pyelography (IVP) for the evaluation of renal uptake and excretion, renal function, and the collecting system. 71 Given the potential for inducing acute tubular necrosis with the use of iodinated contrast in patients with known or suspected renal dysfunction, this is an additional attractive attribute of MRI. Adrenal glands and retroperitoneal structures, including lymph nodes and the major vascular structures, may be similarly analyzed by CT or MRI. It should also be noted that a routine MRI examination should include dynamically acquired contrast-enhanced T1-weighted gradient-echo technique, with images acquired during the arterial, venous, and delayed phases of contrast distribution. The additional information acquired by this approach may be critical in many cases of tumor or in cases of inflammatory or fibrotic processes of the liver and pancreas. Routinely using multiphase contrast-enhanced imaging on CT studies would represent a significant increase in radiation dose. As MRI offers greater specificity for most cases in which multiphase imaging is indicated, it would be reasonable to replace the majority of multiphase CT examinations with MRI and reserve CT for only those patients in whom MRI fails or is contraindicated.
Pelvis
Soft tissues of the female pelvis generally produce only a small range of image densities on CT, leading to low contrast between structures. Generally, US and MRI provide significantly superior visualization of the soft tissues, including the uterus, with clear distinction of the endometrium and myometrium, cervix, and vagina, as well as parametrial and adnexal structures including the ovaries. Compared with US, MRI can generate superior contrast between the different soft tissue structures and may offer additional benefits by providing a full field of view to include the entire pelvis, which can be more challenging on sonographic examinations. 72-74 Examination of the prostate on MRI provides excellent soft tissue detail for the demonstration of the zonal anatomy and for the evaluation of the prostatic capsule and provides important structural delineation for prostatic carcinoma local staging. 75,76 The results of CT and MRI may be similar in the detection of pelvic lymph nodes and vessels.
Bowel
Experience using MRI for the evaluation of the bowel has been increasing, and results show that MRI may be an excellent imaging study for the evaluation of inflammatory bowel disease, 77 including Crohn's disease. It has been shown that CT findings of Crohn's disease do not correlate well with disease activity. Feasibility for using MRI for the evaluation of Crohn's disease and for the determination of disease activity has been reported. 77-83 In particular, the use of dynamically gadolinium-enhanced gradient-echo imaging, in combination with fat-suppressed single-shot echo-train T2- weighted imaging, may yield information that correlates with the degree of active inflammation in and around the involved bowel segments. Feasibility for the use of MRI for the investigation of other acute gastrointestinal disease also has been shown, such as for acute appendicitis. 72 It may be that MRI could be used as the primary imaging modality for the evaluation of acute appendicitis in young adults and in pediatric age patients.
Pregnant patients
Excellent depiction of a full range of disease processes and safety (due to lack of radiation) make MRI ideal for evaluating pregnant women. 84 Ultrasound is a similarly safe imaging modality but has lesser reliability to visualize disease processes and cannot provide the overall topographical display.
Whole-body scanning
Until recently, MRI systems have been used to evaluate disease processes that are limited to a single or a few body regions or organ systems, while CT systems are capable of scanning the entire chest, abdomen, and pelvis during a single breath-hold. Newer engineering developments are being introduced to facilitate more rapid imaging of larger body regions and to efficiently combine the imaging of multiple stations, such as the chest, abdomen, and the pelvis, or to include the entire head, neck, and body. For example, recent studies have shown that imaging the entire body as a method for tumor evaluation and staging, 85-87 or for whole-body MR angiography 88 is feasible.
Recommendations for the practicing radiologist: When and how to use CT
Clearly, CT is an excellent modality for investigating many disease processes. Indications for which CT should be considered a primary diagnostic method include the evaluation of pa-tients with severe trauma, the evaluation of the placement of tubes and catheters in extremely ill patients, the detection of renal and ureteric calculi, and the detection of interstitial lung disease. Radiologists who are responsible for adjusting CT protocols should be aware of the methods by which radiation dose may be minimized with regard to using the lowest possible dose to achieve a diagnosis. 4,31,32 This includes using the minimum kilovoltage and tube current according to the age or size of the patient and ensuring that the thickest slices, least number of phases, and minimal coverage of body parts is performed as is necessary to evaluate the clinical areas of concern. 37
Based on our current understanding of the radiation risks associated with certain CT studies, it is incumbent on the radiology community to discuss with patients the concern in the scientific community that X-rays may result in an increased risk of cancer development. Patients should be given an explanation of the expected benefit in relation to the risk for radiation-induced cancer. It would be reasonable to provide the patient with the LAR estimates for subsequent cancer quoted from the BEIR VII Report: 1 in 1000 for a single CT and cumulatively higher for a multipass CT or for repeated examinations. The risks are lower for older patients and may become <1:10,000 if the patient is older than 50 years of age, but are greater in younger patients and can be described as approaching twice the risk for adult patients, which is estimated to be 1 in 550 for a 1-year-old patient. 19
The current practice for imaging patients with known or suspected intracranial or spinal neurological disorders has been increasingly oriented toward using MRI as a primary imaging technique. A large potential impact on practice patterns would be expected from evaluating body imaging indications. Examples of common indications for which alternatives to body CT should be routinely recommended include patients with known or suspected hepatobiliary, pancreatic, adrenal, and renal disease with the exception of renal calculi. For example, in patients who have had an unenhanced or single-phase enhanced CT scan for an initial assessment of disease and who were found to have a nonspecific hepatic or pancreatic lesion, a multiphase gadolinium-enhanced MRI should be preferred in place of a multiphase CT study. Patients with right upper quadrant pain may initially be screened by abdominal US, and if nondiagnostic, a gadolinium-enhanced abdominal MRI should be recommended. Similarly, female patients with gynecologic or obstetric concerns should first be imaged by US, followed by MRI if the sonographic examination is non-diagnostic. 84
Conclusion
It is essential that the radiology community be committed to dose reduction. The community is responsibile for educating patients about the radiation risks and the use of alternative, safe imaging modalities--in particular, with emphasis on US and MRI--for the foreseeable future. Educational efforts should be directed to radiologists, referring physicians, regulators, public health officials, and patients. A key component for optimizing patient safety should be an aggressive assessment process to limit the improper use of CT.
The BEIR VII Report represents an updated consensus evaluation on low-dose ionizing radiation risks, in the range of exposures associated with routine clinical body CT examinations. These estimates show that the estimated risk is sufficiently significant that clinicians, as patient advocates, should include radiation risk as a component of obtaining informed consent from the patient prior to a CT study. As a routine component to the process of informed consent, clinicians should also discuss and be able to offer an alternative, such as an MRI study, when it can be argued that the MRI examination may provide similar or superior diagnostic results with lower short- and long-term health risks.
When CT examinations are performed, the current responsibility falls on the radiologist who is interpreting the study to ensure that the optimal CT protocol is implemented and that this includes utilizing a technique that will minimize radiation exposure while maintaining the diagnostic sensitivity and specificity of the examination. This includes restricting the dose rate and the field of view imaged. Specific strategies could be considered for particular indications. For example, in many cases, liver examinations using multiple-pass contrast-enhanced studies may be restricted to a single pass, and MRI can be used if the CT study is nondiagnostic. If a patient and clinician choose an MR examination, challenges in our current system include having adequate availability, having adequate expertise in the performance and interpretation of these studies, and obtaining approval for coverage of the MRI costs from the medical insurer. These are surmountable problems, however, and the solution will largely depend upon a greater concerted effort of clinicians to act as patient advocates.