Clinical Performance and Considerations in Coronary CTA
Images
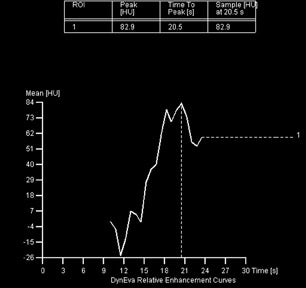
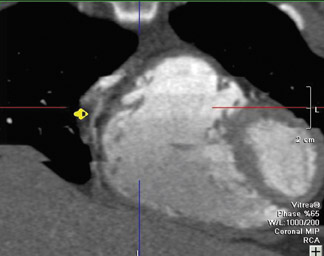
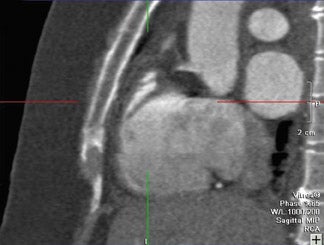
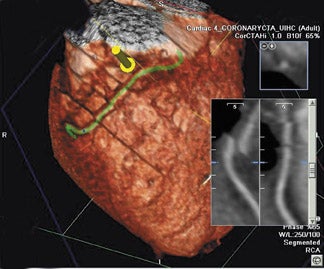
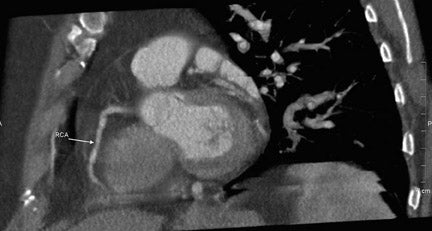
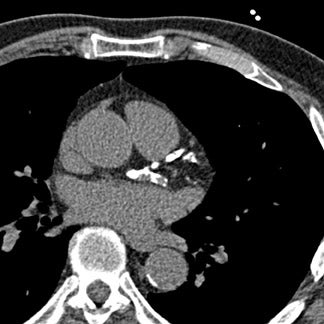
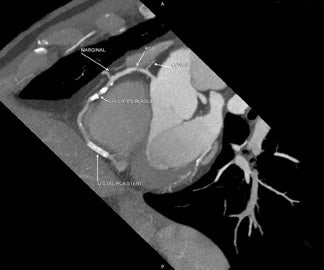
Dr. Staffey is currently a Third-Year Cardiology Fellow at the University of Iowa Hospitals and Clinics in Iowa City, Iowa. She plans to pursue an advanced cardiac imaging fellowship in coronary CT angiography (CTA), echocardiography, cardiac MRI, and cardiac nuclear medicine at the University of Iowa. Dr. Staffey graduated from the University of Illinois College of Medicine, Rockford, IL in 2001. She completed her internship and residency training at the Mayo Clinic in Rochester, MN in 2004. Dr. van Beek is a Professor in the Division of Diagnostic Radiology, Department of Radiology, University of Iowa, Iowa City, IA. Dr. Jagaaia is an Associate Clinical Professor of Cardiology, Department of Internal Medicine, University of Iowa, Iowa City, IA.
Multidetector computed tomography (MDCT) allows for evaluation of native coronary arteries, coronary artery anomalies, bypass graft patency and quantification of atherosclerosis. Multidetector CT provides a safe, accurate, noninvasive alternative to invasive coronary angiograms in a select patient population. Successful CTA acquisition involves various components that this article will focus on, including adequate patient preparation, utilization of beta-blockers for heart rate control, understanding the principles of scan acquisition, and optimization of radiation dose. The current limitations of CTA and the potential role of dual-source CT (DSCT) to resolve many of the current limitations are also discussed.
Noninvasive echocardiographic-gated multidetector computed tomography (MDCT) allows for visualization and characterization of native coronary arteries, coronary artery anomalies, quantification of atherosclerosis and luminal vessel diameter as well as the evaluation of bypass graft patency. The growing acceptance of MDCT as an alternative or adjunct to conventional cardiac evaluations has fostered the rapidly emerging technical advances in the field.
Proper patient selection and adequate patient preparation are vital to the success of the procedure. Radiation exposure is a significant concern with CT angiography (CTA), and new scanning protocols are being evaluated for radiation reduction while maintaining diagnostic image quality. Conventional invasive coronary angiography remains the gold standard for the detection of coronary artery stenosis, in-stent restenosis, and the evaluation of bypass graft patency. The risks of adverse events, cost, and potential complications of the procedure have led to an intensive search for a noninvasive alternative. The growing acceptance of MDCT as an alternative or adjunct to conventional cardiac evaluation has fostered the rapidly emerging technical advances in this field.
The objectives of this article are to provide current information from the literature on some of the fundamental aspects of CTA, including the following: adequate patient preparation, utilization of beta-blockers for heart rate control, principles of scan acquisition, optimization of radiation dose, current limitations of CTA, and potential of dual-source CT (DSCT) to resolve many of the current limitations of CTA.
The technology is rapidly advancing, and with increasing experience the clinical acceptance of MDCT is growing. Multidetector CT will provide a safe, accurate, noninvasive imaging alternative to invasive coronary angiograms.
Patient selection and preparation
Proper patient selection and adequate patient preparation are vital to the successful performance of coronary MDCT. First, patients must be screened for potential risk of iodinated contrast, including allergic reactions and renal insufficiency. Subsequently, upon arrival in the CT suite, patients require heart rate and blood pressure monitoring and need to receive a detailed explanation of the procedure. It is important that patients receive clear instructions on breath-holding and are made aware of the reasons for lying absolutely still during the scanning procedure. Relative contraindications for CTA include patients who are unable to lie still, who are unable to hold their breath for up to 15 seconds, or who have arrhythmias, including atrial fibrillation or frequent premature atrial or ventricular contractions.
Heart rate is a modifiable variable that has a major impact on the quality of the scan. It has been shown that lower heart rates (<65 bpm) produce better-quality scans with improved visualization of all artery segments with more accurate stenosis detection. 1 Lower heart rates provide a longer motion-free time during mid-diastole. 2 Mid-to-end diastole is the portion of the cardiac cycle when the heart has the least amount of motion. Lower heart rates optimize scan acquisition by creating a longer R-R interval-thus, a longer diastolic phase. 3 Optimal heart rate control can be achieved with oral and/or intravenous beta-blockers prior to the scan. One study reported that 20% of patients had heart rates that were above target level in spite of administration of beta-blockers. 4 Thus, most studies have routinely excluded patients with heart rates >65 bpm and patients with irregular heart rhythm. 5,6
Most centers administer 50 to 100 mg oral metoprolol to patients with heart rates >65 bpm approximately 1 hour prior to the scan, followed by additional intravenous metoprolol if required. Knowledge of relative and absolute contraindications for beta-blocker use must be understood and practiced to allow for safe administration.
Image acquisition
The basic principles and sequence of scan acquisitions are quite similar among various scanners. The sequential steps in performing CTA include obtaining planar scout images, followed by a noncontrast electrocardiographic (ECG)-gated CT for calcium scoring. Most centers then obtain a contrast scan with a test bolus of contrast to assess the circulation time of contrast (Figure 1). After a detailed explanation of the study, including breath-hold instructions, the contrast CTA is performed. It must be emphasized that optimal heart rate control (<65 bpm) and a proper breath-hold are integral to the success of the scan.
Spatial resolution is defined as the smallest possible resolution of 2 line pairs that allows sharp and clear delineation of the coronary arteries. Temporal resolution is defined as the speed with which images can be obtained to allow for fast coverage of the cardiac structures within the least number of cardiac cycles. Temporal resolution is determined by the pitch (table advancement speed during a single 360˚ X-ray tube rotation), gantry rotation time, and the patient's heart rate. Multidetector CT is highly dependent on increased temporal resolution to minimize scan time and to reduce coronary motion artifacts. There are current technologic limitations on the pitch and gantry rotation time. Hence, optimal heart rate control plays a critical role in obtaining a good-quality scan. The recent advancements in MSCT technology-including reduced gantry rotation time, improved temporal resolution, and an increment in detector arrays to the current 64channel systems- have improved spatial resolution and, thus, have dramatically improved image quality. 7
Improved image quality is best explained by the increased sensitivity and specificity, and high negative predictive value (NPV) and positive predictive value (PPV) in regard to stenosis detection. Studies indicate that the 16-slice scanners detect >50% stenosis in segments of vessels with sensitivity 92% to 95%, specificity 86% to 97%, NPV 92% to 97%, and PPV 80% to 87%. However, 20% of coronary artery segments, predominantly in small vessels and side branch vessels, are nonevaluable. The modern 64-slice scanners also detect significant stenosis in small coronary artery segments and side branches with higher sensitivity (81% to 94%) and specificity (93% to 97%), and improved NPV (95% to 97%) and PPV (78% to 90%). The significant decrease in the number of nonevaluable segments (7%) contributes to the increased diagnostic accuracy of the 64-slice MDCT. 8
Protocol
Planar scout films are unenhanced images used by the technologist to determine scan volumes. During acquisition of the scout films, the patient performs a breath-hold, and the resulting anteroposterior image is used to ensure that all pertinent vasculature of interest is included in the scanning field. The patient must be consistent with the depth of breath-hold during the scout image acquisition, the non-contrast CT, and the contrast CT examination. A shallow breath-hold will move the heart into a higher position, and a deeper breath-hold will move the heart into a lower position in the chest. 7
A noncontrast CT for calcium scoring is obtained to determine the total calcium burden. 9 Besides calcium scoring, the noncontrast scan can be utilized for the identification of prior stent placement, vascular clips after bypass grafting, foreign bodies, and other objects that may obscure the contrast agent. 7 Coronary calcium is a reliable marker of atherosclerotic plaque burden and angiographic disease and is an independent predictor of cardiovascular events. 10-12 High calcium scores are useful in predicting coronary artery disease (CAD) and helpful in determining the role of MDCT in patients with possible CAD. Calcium scores >1000 or heavily calcified coronary artery seg ments can make accurate interpretation of plaques difficult, leading to overestimation of luminal narrowing. Hoffmann et al 8 determined that calcification was the major underlying cause of overestimation of coronary lumen stenosis resulting in the majority of false-positive findings (94%). Several researchers suggest that CTA should not be performed to diagnose CAD if the patient's calcium score is >1000. 7,9 On the opposite end of the spectrum, Haberl et al 12 reported a very high NPV of coronary calcium scanning. A coronary artery calcium score of 0 effectively excludes hemodynamically significant CAD in 99% of cases. This information is useful in adjusting the patients' pretest probability for CAD and assists in interpretation of the CTA. Of note, radiation exposure from calcium scoring is minimal secondary to prospective gating techniques and utilization of only a single predefined cardiac phase for image reconstruction (1.3 to 1.7 mSv for a 16-slice scanner). 13
Contrast administration for CTA should, ideally, be performed via an antecubital vein, although other injection sites in the upper extremities except for veins in the hands can be used. The use of venous access in the hands is avoided because of the high flow rates of contrast injection. 14 The lower extremities and the hands also cannot be used because of longer delay times. The calculation of transit time from the time of contrast injection must be accurate to ensure adequate contrast opacification of the left ventricle and coronary arteries. Some centers obtain timing scans with a small contrast test bolus (10 to 20 mL) to determine transit time from the injection site to the ascending aorta. This test bolus method to detect accurate transit time can be performed by imaging the ascending aorta every 2 seconds after an initial 10-second delay after contrast injection. The transit time varies with each patient but is typically 15 to 18 seconds. Several factors, such as low ejection fraction, decreased cardiac output, and pulmonary disease, can influence the transit time. 7 Bolus tracking software eliminates the need for a timing test bolus, since the software determines when the contrast has arrived in the coronary arteries and initiates the scan after a preset limit (eg, 100 HU) is reached in the ascending aorta within a predefined region of interest. 13
Most centers use contrast injection rates of 4.5 to 5.0 mL/sec. 7,13-15 Allergic reactions and contrast extravasation from the vein are rare but highly concerning procedural complications. Dual contrast injectors, with one injector filled with contrast and the other with saline, are commonly used to reduce the contrast requirements and improve opacification of the coronary arteries. The total contrast volume is based on the required scan time. 6 Typically, 60 to 80 mL of iodinated contrast with high iodine concentration (300 to 400 mgI/mL) is injected at a flow rate of 4.5 to 5.0 mL/sec, followed by a saline chase bolus of 40 to 70 mL at the same flow rate. 7,13-15
Multiple variables affect image quality and the elimination of respiratory-phase cardiac motion with patient com pliance with breath-holding technique is critical for a high-quality scan. The breath-hold time continues to decrease with the increase in the number of slices that can be obtained in one rotation of the gantry and with increased speed of image acquisition. Although 4-slice scanners required breath-holds of 20 to 40 seconds, modern-era scanners require breath-holds of 15 to 30 seconds for 16-slice and 8 to 20 seconds for 64-slice. 7,16 Breathing artifacts are problematic and create blurring of the images from cardiac motion. Patients need clear and repetitive instructions on appropriate breath-holding prior to scanning.
Retrospective ECG-gated MDCT utilizes simultaneous acquisition of imaging and ECG data during scanning. This allows for flexibility in the reconstruction of the data sets during systolic or diastolic phases of the cardiac cycle. The R-R intervals must be regular and at a heart rate of approximately 60 to 65 bpm for optimal application of the retrospective reconstruction technique. Hence, it is critical that patients referred for CTA are in sinus rhythm. Atrial arrhythmias (flutter, fibrillation), frequent premature atrial contractions, and frequent premature ventricular contrasts are contraindications to MDCT. 7
Image reconstruction
Multidetector CT scanning is performed in the spiral mode, and images are acquired continuously throughout the cardiac cycle. A slice thickness of ≤0.75 mm with 50% overlap is usually used for image reconstruction with a 512 × 512 pixel matrix. In obese patients, images can be reconstructed at 1.0 mm thickness to reduce noise in the image. A small field of view (18 to 20 cm) is used for a dedicated CTA. A medium-smooth reconstruction filter (kernel) is usually used for standard CTA. Reconstruction with a sharper kernel may be used for evaluation of calcified plaques and intracoronary stents. As described previously, a retrospective ECG-gated image reconstruction technique is utilized in CTA to minimize radiation exposure. Although half-scan reconstruction algorithms are used in most patients, multisector reconstruction from several consecutive heartbeats may be used to optimize image quality at faster heart rates (>65 bpm). The half-scan single reconstruction can be done in any phase of the cardiac cycle. 1,3,16-18 It is recognized that each coronary artery has a different pattern of motion during a cardiac cycle. The visualization of the right coronary artery (RCA) is highly sensitive to motion on MDCT secondary to its extensive motion radius and short motion-free period. Motion artifacts account for a portion of noninterpretable coronary artery segments. 1 Reconstructed images earlier in the cardiac cycle (30% to 40% phase, end-systolic) provide diagnostic-quality images for RCA evaluation and later in the cardiac cycle (60% to 80% phase, mid-to-end diastolic) provide quality images of the left coronary system. 1,3,16-18
CT angiographic literature indicates that the optimal reconstruction phase is based on the patients' heart rate. Herzog et al 18 recently published a prospective study in 70 patients that indicated that the R-R interval phase choice should be based on the heart rate at the time of the MDCT. In patients with higher heart rates (65 to 75 bpm) the best image quality was obtained during end-systole and early diastole (27% to 39% of R-R interval), and for patients with low heart rates (<65 bpm) the best image quality was obtained during mid-diastole (60% to 65% of R-R interval). 17
Optimization of radiation dose
Three primary factors determine radiation dosimetry: X-ray energy or tube voltage, tube current, and exposure time. 7 Effective dose refers to the amount of radiation absorbed in different organs and tissues and is a standard means of comparing various imaging modalities. Radiation exposure is a significant concern for MDCT; a current estimated range of radiation exposure is 8 to 22 mSv. Radiation exposure with other cardiac imaging modalities such as cardiac catheterization is 2.5 to 5 mSv, and nuclear testing with single-photon emission CT is 15 to 20 mSv. The current radiation exposure of MDCT is equivalent to 100 to 160 anteroposterior and lateral chest X-rays. 8 Coles et al 19 recently published a 91-patient comparison of effective radiation doses with 16-slice MDCT and diagnostic coronary angiography. The study results revealed a significantly higher mean effective radiation dose associated with MDCT: 14.7 mSv for MDCT versus 5.6 mSv for a diagnostic invasive angiogram.
The technical factors utilized to enhance image quality, particularly increased spatial resolution using a high- voltage current X-ray tube and an increased number of slices per rotation, have resulted in increased radiation exposure. Compared with 16-slice scanners, 64-slice scanners result in a signi-ficantly greater amount of radiation exposure, 11.0 ± 4.1 mSv for 64-slice CT versus 6.4 ± 1.9 mSv for 16-slice CT. 20
The U.S. Food and Drug Administration (FDA) has issued a statement regarding the radiation risks associated with CT screening describing the potential risk of cancer from radiation exposure. 7 The reported cancer risk associated with 14.7 mSv radiation exposure is 1 in 1400 versus 1 in 3600 with diagnostic angiogram (5.6 mSv). 19 Due to the concern and risk associated with medical radiation exposure, new scanning protocols are being evaluated for radiation exposure reduction and image quality.
A recent retrospective study conducted by Hausleiter et al 20 that compared 16-slice to 64-slice scanners in 1035 patients evaluated 2 different radiation dose reduction strategies and the outcomes on image quality. The increased radiation dose with the 64-slice MDCT is due to increased spatial and temporal resolution. High spatial resolution is necessary for optimal evaluation of artery segments. Temporal resolution is improved due to increase gantry rotation speed. To maintain image quality with the reduced slice thickness and faster gantry rotation, the tube current is increased which results in more radiation exposure. 20 The 2 radiation dose reduction algorithms evaluated were ECG-dependent modulation of tube current and reduction in tube voltage.
ECG-dependent modulation of tube current (dose modulation) reduces radiation by decreasing the tube current 80% during ventricular systole. This algorithm allows for high tube current with optimal image quality during mid-diastole, the portion of the cardiac cycle with the least amount of motion and the phases typically utilized for coronary interpretation. Hausleiter et al 20 utilized this algorithm in 82.2% of the patients with a radiation dose reduction of 40% with 16-slice and 32% with 64-slice scanners. The dose reduction did not result in a reduction in image quality. ECG-dependent dose modulation is limited to patients with low heart rates and regular sinus rhythms without premature ventricular contractions.
The second radiation reduction method involved a reduction of tube voltage (X-ray energy). Tube voltage reduction results in greater attenuation by contrast media, creating a higher contrast between arteries and the surrounding tissue. This method provides improved signal and decreased radiation exposure but creates increased image noise. A combination of the 2 methods of radiation exposure reduction reduced exposure 53% with the 16-slice scanner and 64% with the 64-slice scanner. 20
Current limitations of CTA
Multidetector CT has limitations that must be recognized and well understood by clinicians and technologists. Not all patients are candidates for MDCT, and proper patient selection is critical. Artifacts during MDCT acquisition create significant limitations in imaging quality and diagnosis.
Cardiac motion artifacts occur when heart rates exceed approximately 70 to 75 bpm or with increased heart rate variability. This is particularly relevant in the evaluation of the mid RCA. 8 Cardiac motion typically creates blurring or stepladder artifacts. Blurring occurs because the cardiac movement has exceeded the temporal resolution of the scanner. Stepladder artifacts are seen at the end of the breath-hold secondary to increased heart rate and increased heart rate variability (Figure 2). 16
Pulmonary/respiratory artifacts produce stair-step artifacts through the entire dataset and cannot by corrected with image data reconstruction. These artifacts involve the heart border and the anterior chest wall, creating blurring, image gaps, and overlapping of image sections. 16 Pulmonary artifacts can be avoided with careful patient instruction and education on breath-hold technique (Figure 3). 8
Blooming artifacts result from high-attenuation structures, such as calcified plaques or stents. They create an enlarged "bloomed" appearance secondary to partial volume averaging effects that obscure the surrounding coronary lumen. Coronary calcium is very problematic in image interpretation and may result in overestimation of the degree of stenosis, creating false-positive results. Secondary to the significant interpretation limitations of heavily calcified coronary arteries, most centers will not proceed with contrast MDCT if the Agatston score is >1000. 8 Hoffman et al 21 reported that 94% of the false-positive findings in their prospective, blinded assessment of 33 patients were secondary to overestimation of coronary lumen obstruction from calcification. Despite the increased temporal and spatial resolution of 64-slice scanners, which allow for more optimal assessment of calcified segments of the coronary arteries, severely calcified coronary plaques remain a significant limitation to interpretation (Figure 4). 22
Beam-hardening artifacts result when the X-ray beams cross a high-density structure (calcified plaque, stent, surgical clips), creating artifacts that resemble noncalcified plaques in adjacent coronary lumen (Figure 5). 8.
Structure-related artifacts are the result of overlapping contrast-filled normal anatomic structures, such as left atrial appendage and cardiac veins. Cardiac veins may obscure evaluation of the coronary arteries, leading to pseudostenosis. 16 Obesity creates image noise, and utilization of soft kernels may improve image quality. 14
A current significant limitation of MDCT is the assessment of stent patency and/or in-stent restenosis. Currently, stent diameters >3 mm can accurately be evaluated with MDCT. 8 The assessment of in-stent restenosis in smaller diameter stents (<3 mm) is limited secondary to the high-density of the stent obscuring the visualization of the lumen or beam-hardening artifacts from the stent struts. 8 Sharper filters or hard kernels and thinner slices improve in-stent visualization. 23
Multidetector CT cannot detect sluggish, retrograde, or antegrade flow in the coronary arteries, thus the filling patterns of the arteries can be difficult or impossible to determine. A final important limitation of MDCT is its inability to provide functional information about the physiologic significance of an intermediate-grade coronary stenosis.
Trends and developments in cardiac CT
Dual-source CT is a new imaging technique that improves temporal resolution of cardiac scans and eliminates the need for heart rate control. Temporal resolution of <100 msec is required to enable adequate cardiac imaging without heart rate control. Current scanners cannot mechanically achieve this rotation speed. The DSCT utilizes 2 arrays of X-ray tubes and 2 corresponding detectors that are mounted on the rotating gantry and angled 90º apart. 24,25 One detector covers the entire scan field of view, while the other detector is limited to the central field of view. The DSCT provides improved temporal resolution at one-fourth the gantry rotation time; thus, ECG-gated single-segment (one cardiac cycle only) reconstruction with temporal resolution of 83 msec can be obtained. The patient's heart rate is no longer an obstacle to scan acquisition because only 1 cardiac cycle is needed for image reconstruction. Initial experiences in the literature on DSCT from Achenbach et al 24 and Flohr et al 25 indicate that DSCT provides improved image quality in patients with high heart rates.
Another technological advancement in CT is the 256-slice MDCT. The 256-slice scanners will allow for wide anatomic volume coverage (128 mm of anatomy with 0.5 mm slices) with a single gantry rotation. The primary benefit of this wide volume coverage is the ability to perform dynamic examinations of large organs, such as the heart, including the whole organ and perfusion in the single gantry rotation. As described before, conventional MDCT systems acquire phasic information of the entire volume by performing multiple helical runs or limited dynamic information for a limited anatomic volume. The 256-slice MDCT will eliminate this current imaging limitation and allow for better imaging quality in a more diverse patient population. The major current concerns about 256-slice scanners are increased radiation exposure and increased cone angle, which could create cone-beam artifacts that limit diagnostic capability. 26,27
Mori et al 26 recently compared the radiation dose in 256-slice and 16-slice scanners. Interestingly, the radiation dose for the 256-slice scanners was smaller than the dose with 16-slice scanners for all examinations performed (head, chest, abdomen, and pelvis). The decreased dose with the 256-slice scanners is the result of the large beam width resulting in greater anatomic coverage without the need for overlap margin coverage. The 256-slice scanners provide sufficient image quality for diagnosis with 1 rotation of the gantry and, thus, less radiation exposure. The 256-slice scanners are in evolution and the utility of the application to coronary artery evaluation remains to be determined. However, the current literature supports the future application of the 256-slice scanners to dynamic whole- organ evaluation.
Conclusion
This article reviewed the current literature and addressed 5 key principles of coronary CTA: appropriate patient selection and preparation, utilization of beta-blockers for heart rate control, the principles of scan acquisition and optimization of radiation dose, recognition of the current limitations of CTA, and potentials of DSCT to address the current limitations of CTA. Multidector CT is rapidly gaining acceptance as an imaging modality for coronary artery disease detection and quantification, stent assessment, and bypass graft evaluation. However, clinicians must understand the limitations of MDCT. Severe coronary calcification is an important limitation that may preclude visualization of coronary artery lumen and may be a contraindication to CTA acquisition. The current amount of radiation exposure from MDCT is of concern and must be addressed. Novel radiation dose reduction algorithms are currently being evaluated. MDCT technology has made major strides in the last 5 years. MDCT provides a safe noninvasive imaging alternative to diagnostic invasive coronary angiography. With the progressive advances in MDCT technology, it is expected to continue playing a major role in the assessment of the cardiovascular system.