Pearls and pitfalls of pediatric head trauma imaging
Images
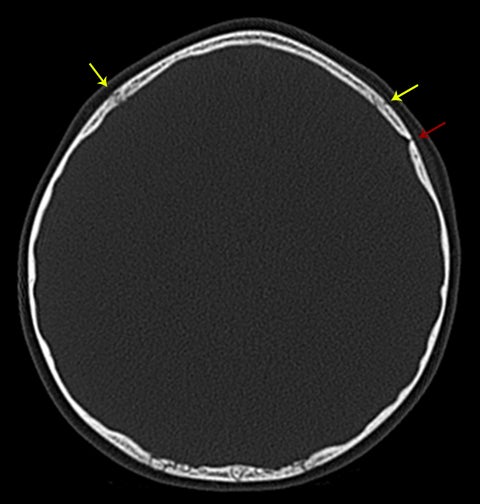
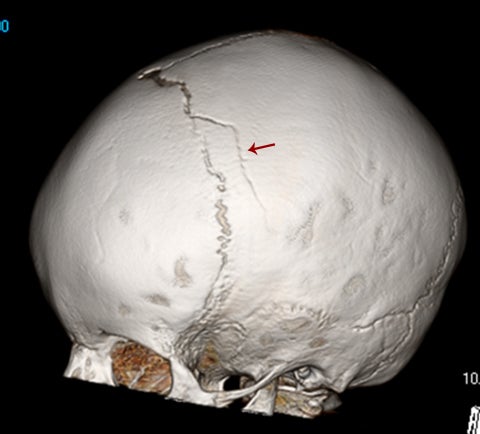
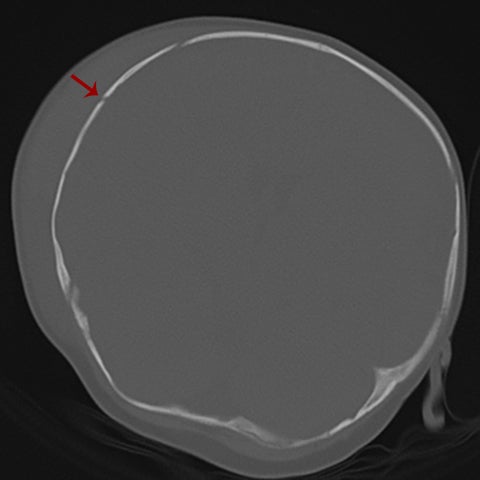
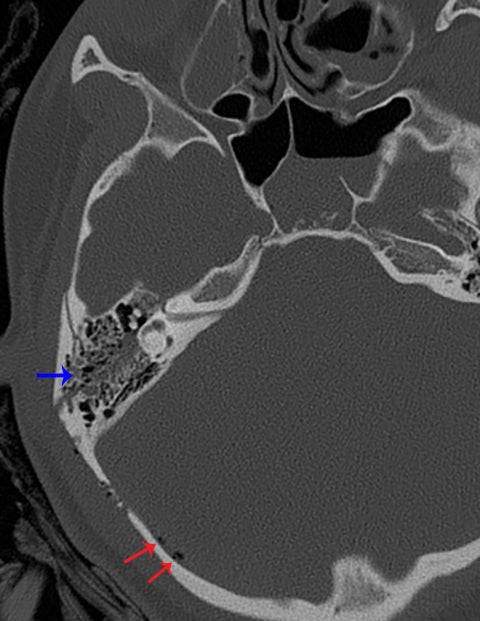
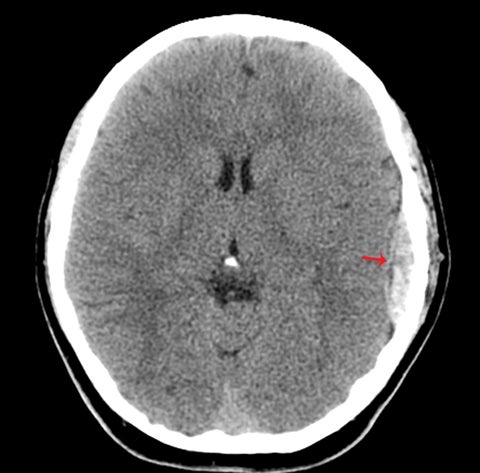
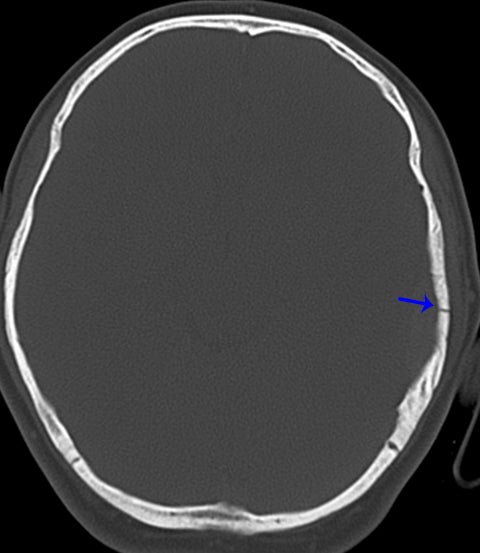
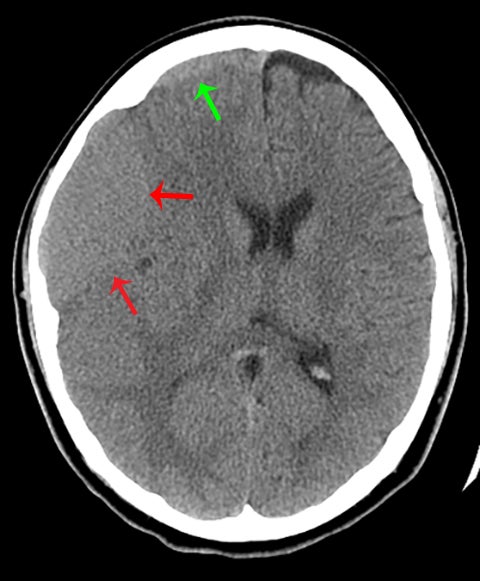
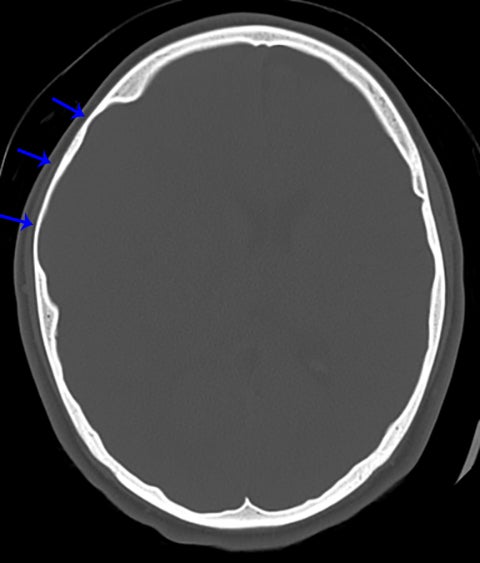
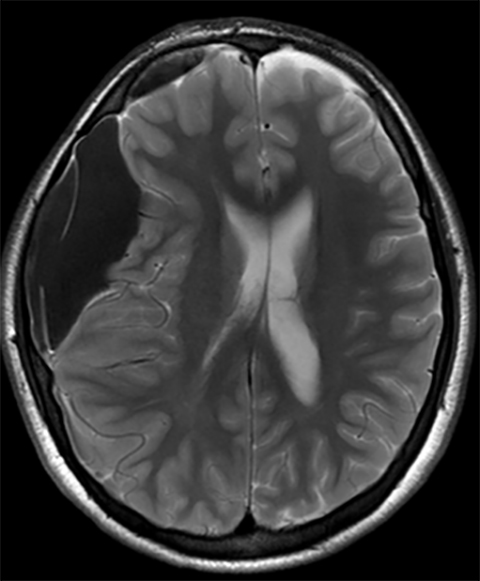
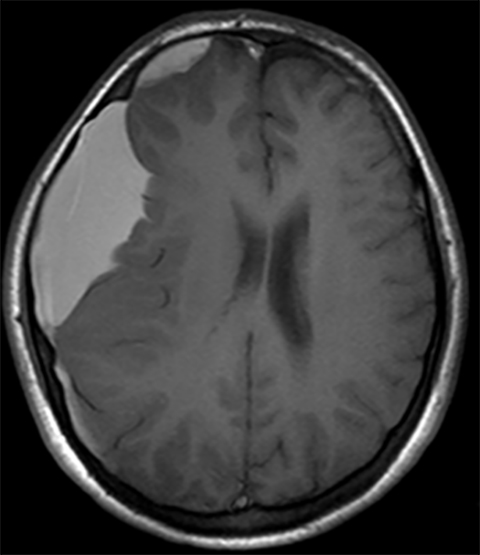
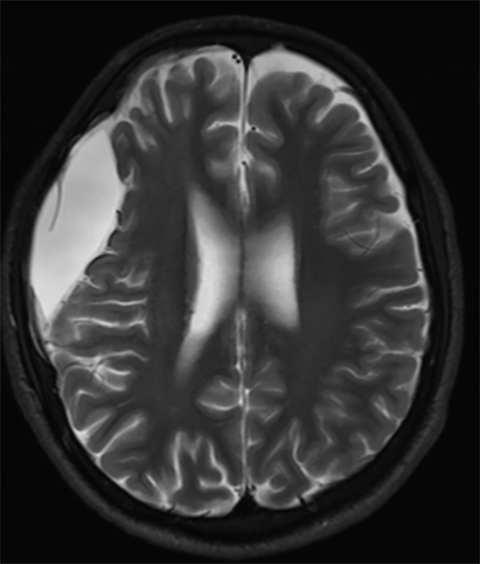
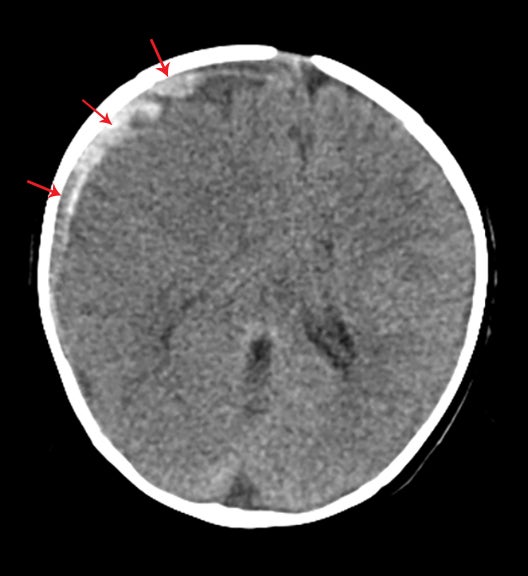
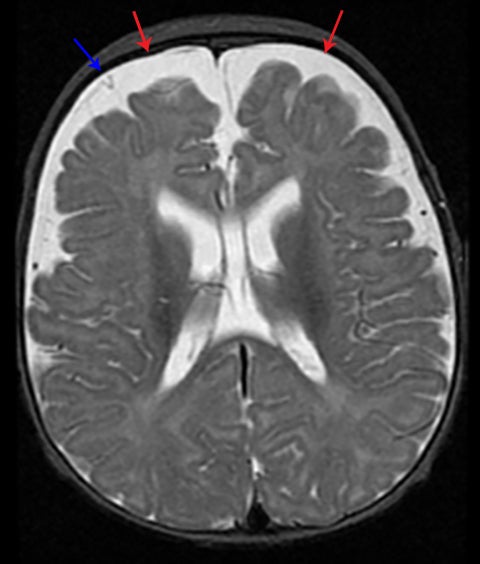
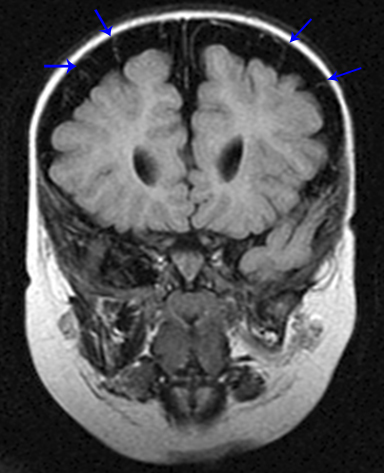
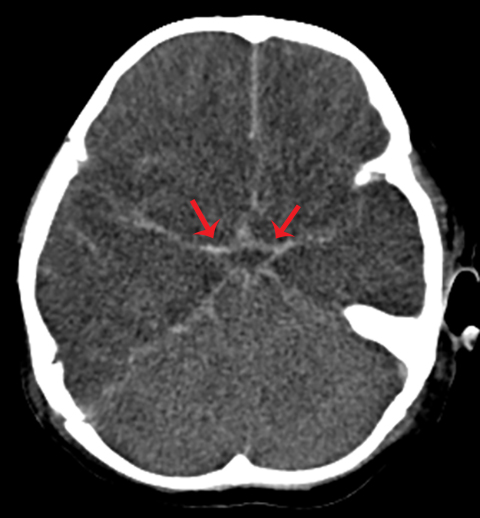
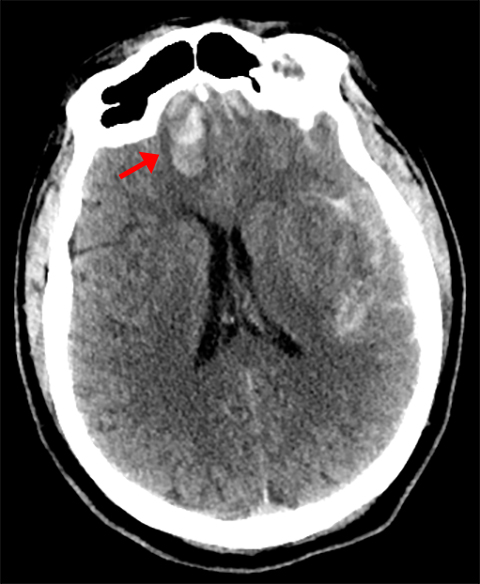
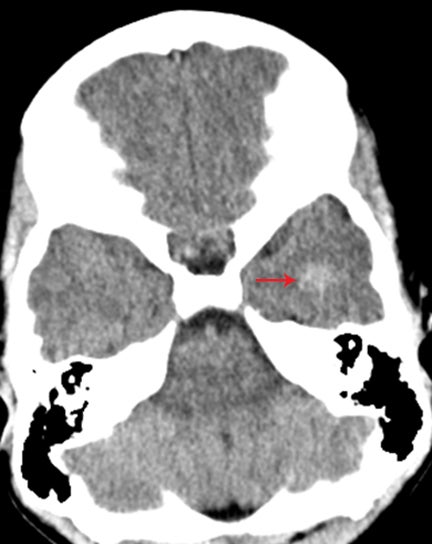
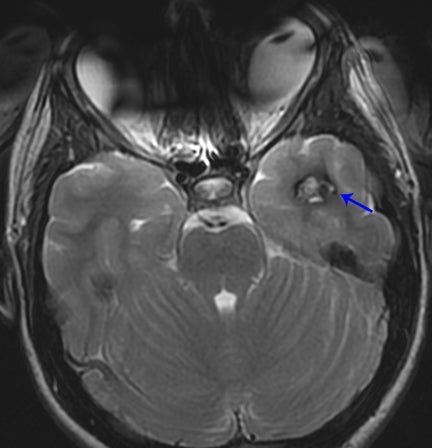
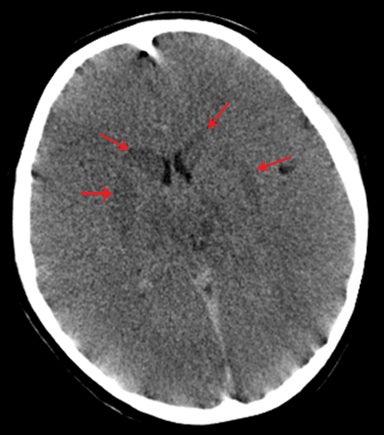
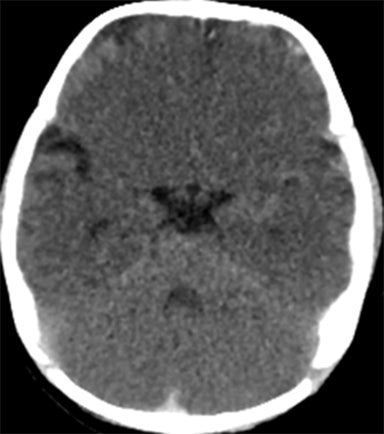
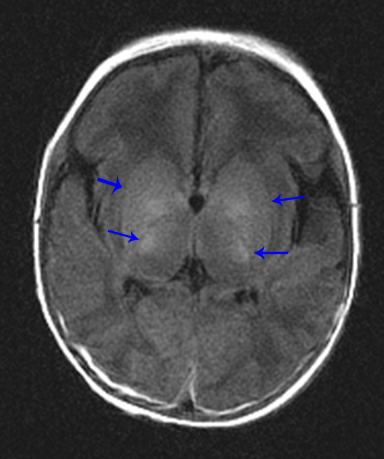
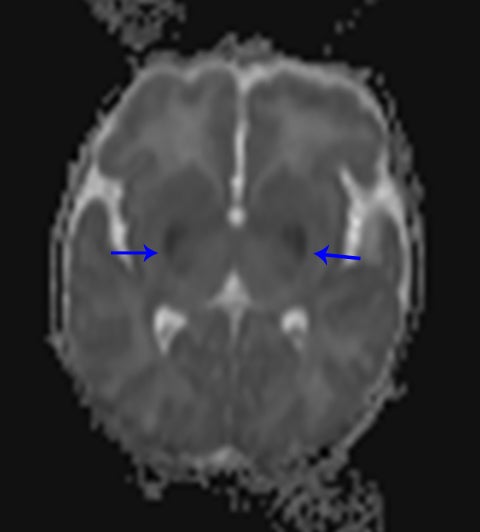
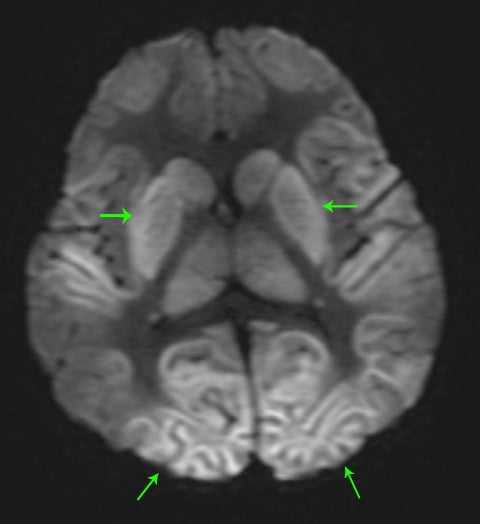
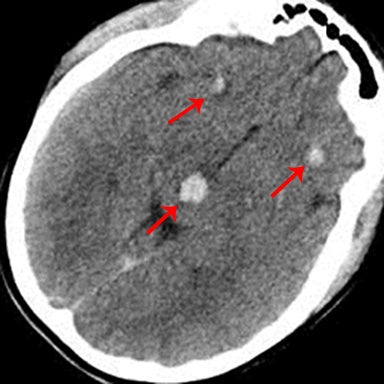
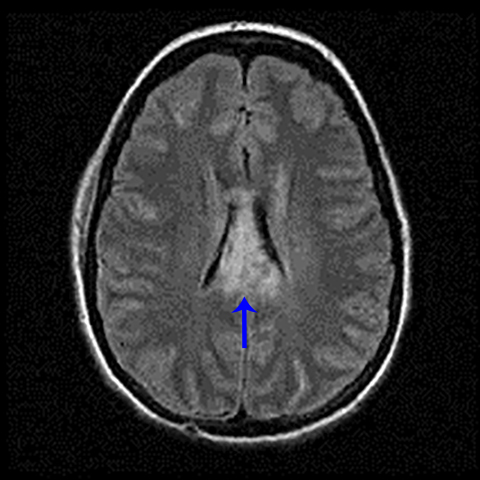
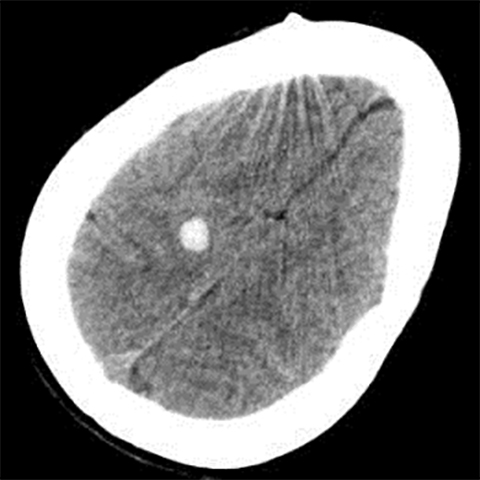
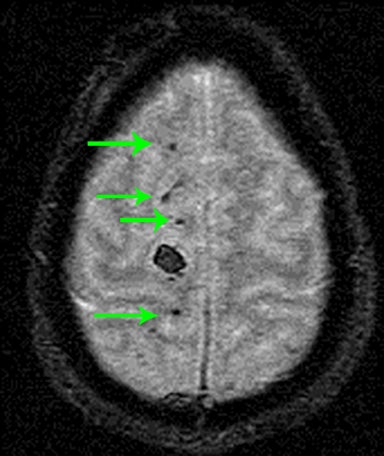
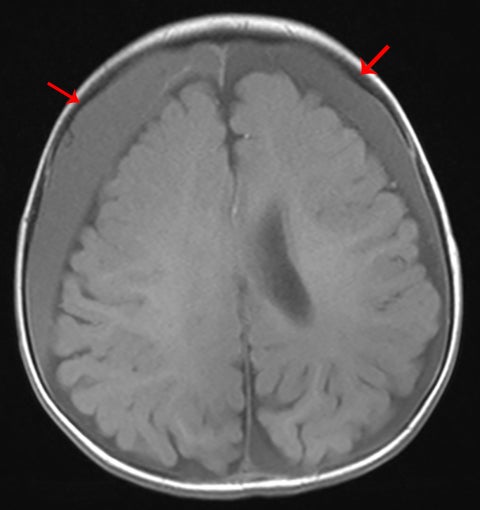
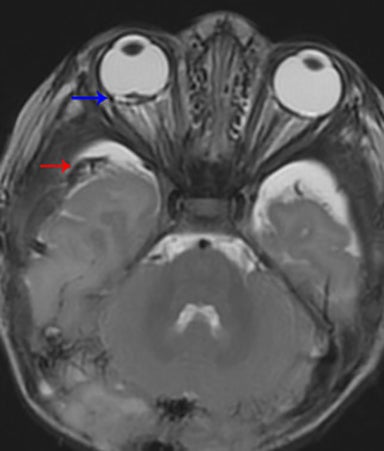
Pediatric head trauma, whether it involves high-velocity impact, self-inflicted causes, or nonaccidental trauma, is a major cause of morbidity and mortality. It is estimated that the annual incidence of pediatric head trauma is on the order of 0.2% to 0.3% in the United States.1,2 The radiologist’s role on the trauma team is to promptly and accurately recognize and report the degree of injury. This not only allows clinicians to determine an appropriate strategy for treatment, but avoids delay in treatment that can result in significant morbidity and mortality.
Head trauma can be separated into two categories: primary brain injury, which occurs at the time of trauma, and secondary brain injury, occurring after the initial insult. Primary brain injury may be due to direct impact or indirect forces.2,3 Examples of primary brain injury include skull fractures, subdural and epidural hematomas, hemorrhagic contusion, and diffuse axonal injury. Secondary brain injury is due to anoxia, as may be seen in the setting of birth trauma or drowning.3 Nonaccidental trauma may take the form of primary and/or secondary brain injury. Obvious intracranial abnormalities are often picked up easily on imaging; however, it is important to be able to distinguish between true pediatric trauma and its mimics.
This article approaches pediatric head trauma in two parts; the first will compare and contrast the differences between true lesions and mimics, which may often represent normal variations in the pediatric age group and/or are considered “don’t-touch” lesions.Finally, the authors will discuss lesions which should not be missed by any radiologist.
Calvarial fractures and associated mimics
Calvarial fractures are a common finding in both accidental and nonaccidental trauma and can have far-reaching effects in both clinical and social management.4 However, the presence of sutures, accessory sutures, and wormian bones can confound their timely diagnosis. Fractures in the imaging plane can be extremely difficult to identify on conventional axial computed tomography (CT) slices, making a good case for always evaluating the CT scout images. Modern development of 3-dimensional reconstructions of CT data, which require minimal post-processing time and no additional radiation, have enhanced the ability of the radiologist to confidently diagnose and describe calvarial fractures, and accurately identify their mimics.4
Normal sutures are symmetric and found in expected locations. Thus, the radiologist reading pediatric trauma studies should be familiar with normal sutural anatomy, typical times of sutural closure, and common accessory sutures to avoid misdiagnosis. Normal sutures are usually identified on both axial CT images and 3-dimensional reconstructions by their typical zigzag course and sclerotic borders (Figure1). One must also carefully evaluate normal-appearing sutures for the presence of diastasis, as these are the most common of pediatric skull fractures and also tend to be more subtle than their linear, comminuted, or depressed counterparts.5 The use of symmetry is a helpful tool for identifying sutural diastasis.
Accessory sutures most commonly occur in the parietal and occipital bones, most likely as a consequence of their multiple ossification centers. Like normal sutures, they demonstrate a similar zigzag course without diastasis and merge with adjacent sutures. They are often bilateral, in which case they are relatively symmetric.6
True fractures generally show a linear course with lack of well-defined sclerotic borders. In contrast to normal or accessory sutures, they may cross, rather than merge with adjacent sutures. Fractures are more often unilateral and asymmetric. When bilateral, as sometimes seen in high-impact trauma, there is often associated displacement and/or comminution, with asymmetry as the rule.6 The presence or lack of local soft-tissue swelling is an extremely valuable finding, as calvarial fractures rarely occur in the absence of an overlying soft-tissue abnormality (Figure 2).7 However, one must be careful of dismissing a suspicious finding based on lack of soft tissue abnormality alone, especially in the setting of nonaccidental trauma, where late presentation is not uncommon.
Other helpful clues to subtle calvarial fractures include pneumocephalus, which sometimes may only be a few foci of gas, or fluid within the aerated spaces such as the mastoids (Figure 3). In rare difficult cases where a definitive diagnosis cannot be reached, close-interval follow up can be a helpful problem-solving tool, which can confirm presence of fracture healing at 2 to 3 months.6
Extra-axial hemorrhage
Extra-axial hemorrhage is a common primary manifestation of trauma in children. Although its appearance is classic and generally well known, several pitfalls can lead to misdiagnosis and subsequently to suboptimal clinical management. Extra-axial hemorrhage is classified by the space in which it occurs—epidural, subdural, and subarachnoid.
Epidural hemorrhage is characterized by its lentiform shape and respect of sutural boundaries; it may cross midline. Epidural hemorrhage is due to injury of either epidural arteries or veins and often associated with an overlying calvarial fracture and soft-tissue swelling (Figure4).8 Depending on its configuration, intracystic hemorrhage into an arachnoid cyst may mimic either epidural or subdural hemorrhage with associated mass effect. Although generally rare, a higher risk of this complication occurs in patients with larger cysts and those who have experienced trauma.9 Treatment for this entity is unique to each patient, with some cases adequately managed conservatively, in which case a premature diagnosis of epidural hematoma could lead to unnecessary craniotomy.10 This pitfall can be avoided by close attention to the overlying calvarium.
Arachnoid cysts are developmental lesions that are most often incidentally discovered. The chronic presence of an arachnoid cyst thins and remodels the inner table of the calvarium, which is suggestive of the diagnosis and is seen best on CT with bone windows (Figure 5). However, even when the diagnosis appears clear, care should be taken to exclude superimposed subdural hematomas that can b epresent in these patients.11
Subdural hematoma is characterized by its crescentic shape and ability to cross sutures.8 Subdural hemorrhage is bounded by the midline falx and is due to tearing of bridging cortical veins (Figure 6). Benign enlarged subarachnoid spaces of infancy (BESSI) can look similar to chronic bifrontal subdural hematomas, but is a normal variant that should be considered in the appropriate clinical setting. Differentiating between these entities can be difficult and may require further evaluation with magnetic resonance imaging (MRI),where the unique features of each entity are better distinguished. BESSI is typically located along the frontal lobes and between the anterior interhemispheric fissure. Vessels are typically seen traversing the enlarged subarachnoid space, with lack of mass effect, normal to slightly enlarged ventricles, and no evidence of hemorrhagic products (Figure 7). In contrast, subdural hematomas may be asymmetric,demonstrate mass effect, have non-cerebrospinal fluid (CSF) intensity signal within or along the collections, and displace vessels along the inner table of the calvarium.12
Subarachnoid hemorrhage appears as increased attenuation in the cerebral sulci and CSF cisterns on CT.8 The presence of subarachnoid hemorrhage in the setting of trauma is not an unexpected finding. However, high attenuation in the basal cisterns often associated with subarachnoid hemorrhage is not pathognomonic, especially in the presence of other important imaging findings. Diffuse cerebral edema either primarily or secondarily related to trauma is one of several causes of pseudo-subarachnoid hemorrhage, which can confound the diagnosis of subarachnoid hemorrhage. This phenomenon is thought to be related to a combination of displaced CSF, distention of superficial vasculature, and low attenuation edema within the adjacent cortex. Although its appearance is that of subarachnoid hemorrhage, closer evaluation of density yields significantly lower Hounsfield units than true subarachnoid hemorrhage (Figure 8).13
Intraparenchymal contusions versus cavernoma or other underlying lesions
Cortical contusions account for approximately 50% of all intra-axial trauma in the pediatric population. Typical contusions tend to be multiple, bilateral, and occur along the inferior frontal lobes and anterior temporal lobes, where the brain has a greater chance of direct impact against rigid bone. Within the frontal lobe, injury is usually just above the cribriform plate and lesser sphenoid wing. In the temporal lobe, injury occurs adjacent to the petrous bone and greater sphenoid wing (Figure 9). In younger children, cortical contusions are less common because the inner table of the skull is smoother, and therefore, the above locations may not be typical.1,2,14 It is important to look at the soft tissues of the scalp, as intraparenchymal contusions are classically coup (just below the point of direct impact) or contracoup(opposite to the site of direct impact) in location.
By definition, cortical contusions occur in the gray matter and tend to spare the adjacent subcortical white matter, unless the hemorrhage is large.2 These may be difficult to identify on CT in the early stage, as one may only see faint areas of hypoattenuation reflecting early edema, with patchy punctate areas of hyperattenuation representing hemorrhage. Close follow-up imaging will unmask these subtle hemorrhages.14 MRI of the brain is extremely useful, as even more subtle lesions can be identified; one must look for foci of high T2 signal edema and for punctate areas of low signal on gradient echo or susceptibility-weighted images reflecting hemorrhage.
Occasionally, a head CT may have a single focus of hyperattenuation, and the possibility of an incidental cavernous malformation must be considered. In patients with multiple hereditary cavernous malformations, multiple foci of hyperattenuation can be seen, although this is rare. A cavernous malformation, also known as a cavernoma, cavernous angioma, or cavernous hemangioma, consists of a compact mass of sinusoidal-type vessels with no intervening brain parenchyma.15,16 In those lesions that are not complicated by hemorrhage, areas of hyperattenuation on CT may represent foci of calcification. They tend to have no surrounding mass effect or vasogenic edema, unless complicated by superimposed hemorrhage. Cavernomas are typically centered in the subcortical regions and extend outwards toward the cortex, which is another key factor in distinguishing this incidental lesion from acute cortical contusion.17
MRI proves useful in questionable cases. Hemosiderin deposits within the lesion cause a rim of hypointense signal on all sequences. Post-contrast images will demonstrate variable enhancement.17 T2-weighted images and susceptibility-weighted images demonstrate a centralarea of reticulation and a peripheral halo of hypointense signal, giving the characteristic “popcorn” appearance (Figure 10).18,19 Cavernomas are often seen together with developmental venous anomalies, and identification of these enlarged medullary veins can help confirm the diagnosis.
Other mimics of intraparenchymal contusions include hemorrhage secondary to an underlying lesion, such as an arteriovenous malformation or tumor. In such cases, location, morphology, and degree of surrounding edema can be helpful clues to an accurate diagnosis.
Symmetric-appearing brain versus anoxic brain injury
One of the unique aspects of reading a CT or MRI exam of the head is that there is an internal standard of symmetric brain structures. At first glance, it is easy to pick up small findings just by comparing the left and right hemispheres; however, this technique can also be a major pitfall in pediatric imaging.
A normal pediatric head CT should have normal gray-white matter differentiation, with a distinct appearance of the basal ganglia. Any loss of blood flow or profound hypoxia can cause diffuse cerebral swelling, which will appear symmetric and can potentially be called normal by the untrained eye. CT will demonstrate loss of the gray-white matter differentiation, sulcal and ventricular effacement, as well as effacement of the cisterns.2 One should look for low attenuation of the deep gray nuclei because these structures are extremely vulnerable to hypoxic ischemic insults, even if the event is short in duration, due to their inherent high metabolic activity and oxygen requirement (Figure11). It is also important to remember that the midbrain and cerebellum are the least vulnerable to hypoxic injury, and therefore, diffuse cerebral injury may result in the appearance of a relatively lower attenuation cerebrum, when compared to the cerebellum, causing the “white cerebellum sign” (Figure 11).20 In cases of trauma with anoxia, CT is usually the first imaging modality employed. Therefore, correctly identifying the diagnosis on CT should appropriately prompt further evaluation with MRI to characterize the extent of injury.
In general, with neonates, mild cases of hypoxic-ischemic injury tend to involve only parts of the deep gray nuclei, with progressively severe forms involving a majority of the deep gray nuclei with peripheral extension to involve the cortex. With mild injury, T1-weighted images will demonstrate high signal within the ventrolateral thalami and posterolateral putamina. The T1 hyperintensity of the posterior limb of the internal capsule seen in full-term infants may or may not be preserved (Figure 11). Moderate injury tends to cause T1 hyperintensityof the anterior putamen, and the posteromedial thalamus, with usual loss of the normal T1 hyperintense signal in the posterior limb of the internal capsule. Severe injury causes T1 hyperintensity in the perirolandic cortex, insula, or possibly even involving all the cortices (Figure11).21.22 In neonates, there may be no appreciable T2 signal change except for subtle indistinctness of gray-white matter junctions; later in life, one will see areas of T2 hyperintensity. Diffusion-weighted images will demonstrate corresponding areas of restricted diffusion and may be the only images that reveal subtle injury.1
“Don’t-miss” lesions
Diffuse axonal injury
When assessing trauma, it is important to look at the history and mechanism of injury. Depending on the location of edema and/or hemorrhage, one should be able to make the diagnosis of diffuse axonal injury — a diagnosis which has critical prognostic implications due to its poor clinical outcome and nonsurgical management. These patients tend to present with loss of consciousness directly after head injury.14,23 The mechanism of injury is a shear-strain deformation of the brain due to rotational acceleration and deceleration, and therefore, this entity is also known as “shearing injury”.23,24
As the name implies, these lesions occur within the axonal white matter. The most common location is the lobar white matter, followed by the corpus callosum (a majority of which are in the posterior body and splenium), and finally the brainstem (Figure 12). Less common locations include the cerebellar peduncles and basal ganglia.14,23,24 CT may not have any appreciable findings, or only possible faint foci of hemorrhage or edema. For this reason, or in the setting of high clinical suspicion, it is important to recommend MRI for further evaluation.On MRI, it is critical to look for foci of low signal on susceptibility-weighted images representing hemorrhage (Figure 12).25 Diffusion-weighted images may also show areas of restricted diffusion.26
Nonaccidental trauma
Due to the medical, legal, and social implications, another “don’t-miss” lesion is nonaccidental head injury. When looking at a pediatric head scan, it is important to look at both the pattern and various stages (acute versus chronic) of injury. Homogeneously hyperattenuating subdural hematoma on CT is more commonly seen in children with accidental head trauma than those with nonaccidental head trauma.Mixed attenuation within a subdural hematoma or two (or more) subdural hematomas of varying ages should raise the suspicion for nonaccidental head injury (Figure 13).27
One term frequently used in literature is “shaken-baby syndrome;” it implies a rotational acceleration type of injury. The bridging veins that connect the dural sinuses to the brain are stretched, which causes hemorrhage into the subdural and subarachnoid space. The same stretching mechanism occurs along the axons in the white matter and in the retina. Therefore, the constellation of subdural hematoma,diffuse axonal injury, and retinal hemorrhage should alert the radiologist to suspect child abuse (Figure 14).28.29 This suspicion must be promptly relayed to the referring physician.
It is also critical to look at both the history of the trauma, as well as other organ systems to get a global sense of injury. Look for fractures in various stages of healing, posterior rib fractures, vertebral body-compression fractures, duodenal hematomas, and pancreatic injury.29 These patients may present with apnea, irritability, and decreased consciousness.1,2
Atlanto-occipital dissociation/dislocation
Radiologists in training are often reminded to look at the edges of all imaging studies, a pearl that proves crucial in pediatric head trauma imaging. Due to concern about radiation exposure in the pediatric population, some clinicians may only perform a CT scan of the head without the cervical spine. It is important in these situations that the radiologist takes time to evaluate the craniocervical junction.
Osteoligamentous injuries at the craniocervical junction are of critical importance and can have devastating clinical consequences that are avoidable in some cases with early diagnosis and treatment. Ligamentous laxity, large size of the head relative to the body, relative weakness of the cervical musculature, and hypoplastic occipital condyles predispose children less than 10 years of age to atlanto-occipital injury.30,31 Reportedly, 20% of patients with atlanto-occipital injuries initially present neurologically intact.32 In some cases, dissociation of the atlanto-occipital joint is clear. In more subtle cases, perhaps in the setting of secondary signs of injury such as prevertebral soft-tissue swelling, measurements and ratios are often used to help make the diagnosis. Recent studies have demonstrated the usefulness of the atlanto-occipital joint interval (condyle-C1 interval, or CCI) above other commonly used ratios/measurements in identifying subtle craniocervical dissociation, with measurements above 2.5 mm in the coronal or sagittal planes considered abnormal.33-35
In cases where cervical spine injury is suspected in the absence of CT findings, MRI can be an excellent problem-solving tool that may help to identify ligamentous injury that is occult on other imaging modalities.36
Conclusion
The radiologist clearly plays an integral role in the setting of pediatric trauma. Prompt identification of the site and severity of injury can prove to be lifesaving for the pediatric patient. It is important to be aware of not only the patient’s mechanism of injury, but also to the location and pattern of injury, paying special attention to the peripheral aspects of the imaging study. In instances of a mimic, proper recognition can prevent unnecessary treatment or surgery.
REFERENCES
- Woodcock RJ, Davis PC, Hopkins KL. Imaging of head trauma in infancy and childhood. Semin Ultrasound CT MR. 2001;22:162-182.
- Poussaint TY, Moeller KK. Imaging of pediatric head trauma. Neuroimaging Clin N Am. 2002;12:271-294.
- Holbourn AHS. Mechanics of head injuries. Lancet. 1943;242:438-441.
- Prabhu SP, Newton AW, Perez-Rossello JM, Kleinman PK. Three-dimensional skull models as a problem-solving tool in suspected child abuse. Pediatr Radiol. 2013;43:575-581.
- Mulroy MH, Loyd AM, Frush DP, et al. Evaluation of pediatric skull fracture imaging techniques. Forensic Sci Int. 2012;214:167-172.
- Sanchez T, Stewart D, Walvick M, Swischuk L. Skull fracture vs. accessory sutures: How can we tell the difference? Emerg Radiol. 2010;17: 413-418.
- Osborn A. Trauma. In: Osborn’s Brain: Imaging, Pathology, and Anatomy. 1st ed. Salt Lake City, UT: Amirsys 2012:11.
- Barkovich AJ, Schwartz ES. Brain and Spine Injuries in Infancy and Childhood. In: Barkovich AJ, Raybaud C, eds. Pediatric Neuroimaging. 5th ed. Philidelphia, PA: Lippincott Williams & Wilkins, 2012:335-338.
- Cress M, Kestle JRW, Holubkov R, Riva-Cambrin J. Risk factors for pediatric arachnoid cyst rupture/hemorrhage: A case-control study. Neurosurgery. 2013;72:716-722.
- Patel AP, Oliverio PJ, Kurtom KH, Roberti F. Spontaneous subdural hematoma and intracystic hemorrhage in an arachnoid cyst. Radiology Case Reports. 2009;4:298.
- Iaconetta G, Esposito M, Maiuri F, Cappabianca P. Arachnoid cyst with intracystic haemorrhage and subdural haematoma: Case report and literature review. Neurol Sci. 2006;26:451–455.
- Barkovich AJ, Schwartz ES. Hydrocephalus. In: Barkovich AJ, Raybaud C, eds. Pediatric Neuroimaging. 5th ed. Philidelphia, PA: Lippincott Williams & Wilkins, 2012:839-840.
- Given CA II, Burdette JH, Elster AD, Williams DW III. Pseudo-subarachnoid hemorrhage: A potential imaging pitfall associated with diffuse cerebral edema. AJNR. 2003;24:254–256.
- Gentry LR. Imaging of closed head injury. Radiology. 1994;191:1-17.
- Smith ER, Scott RM. Cavernous malformations. Neurosurg Clin N Am. 2010;21:483-490.
- Vilanova, JC, Barceló J, Smirniotopoulos JG, et al. Hemangioma from head to toe: MR imaging with pathologic correlation. Radiographics. 2004;24:367-385.
- Vogler R, Castillo M. Dural cavernous angioma: MR features. AJNR Am J Neuroradiol. 1995;16:773-775.
- Rigamonti D, Drayer BP, Johnson PC, et al. The MRI appearance of cavernous malformations (angiomas). J Neurosurg. 1987;67:518-524.
- Rocco CD, Iannelli A, Tamburrini G. Cavernomas of the central nervous system in children. Acta Neurochir. 1996;138:1267-1274.
- Bathla G, Hegde AN. MRI and CT appearances in metabolic encephalopathies due to system diseases in adults. Clin Radiol. 2013;68:545-554.
- Heinz RE, Provenzale JM. Imaging findings in neonatal hypoxia: A practical review. AJR Am J Roentgenol. 2009;192:41-47.
- Izbudak I, Grant PE. MR imaging of the term and preterm neonate with diffuse brain injury. Magn Reson Imaging Clin N Am. 2011;19: 709-731.
- Parizel PM, Özsarlak Ö, Van Goethem JW, et al. Imaging findings in diffuse axonal injury after closed head trauma. Eur Radiol. 1998;8:960-965.
- Arfanakis K, Haughton VM, Carew JD, et al. Diffusion tensor MR imaging in diffuse axonal injury. AJNR Am J Neuroradiol. 2002;23:794-802.
- Tong KA, Ashwal S, Holshouser BA, et al. Hemorrhagic shearing lesions in children and adolescents with posttraumatic diffuse axonal injury: Improved detection and initial results. Radiology. 2003;227:332-339.
- Hergan K, Schaefer PW, Sorensen AG, et al. Diffusion-weighted MRI in diffuse axonal injury of the brain. Eur Radiol. 2002;12:2536-2541.
- Tung GA, Kumar M, Richardson RC, et al. Comparison of accidental and nonaccidental traumatic head injury in children on noncontrast computed tomography. Pediatrics. 2006;118:626-633.
- David TJ. Shaken baby (shaken impact) syndrome: Non-accidental head injury in infancy. J R Soc Med. 1999;92:556-561.
- Lonergan GJ, Baker AM, Morey MK, Boos SC. From the archives of the AFIP. Child abuse: Radiologic-pathologic correlation. Radiographics. 2003;23:811-845.
- Deliganis AV, Baxter AB, Hanson JA, et al. Radiologic spectrum of craniocervical distraction injuries. Radiographics. 2000;20:S237–S250.
- Bertozzi JC, Rojas CA, Martinez CR. Evaluation of the pediatric craniocervical junction on MDCT. AJR Am J Roentgenol. 2009;192:26-31.
- Fisher CG, Sun JC, Dvorak M. Recognition and management of atlanto-occipital dislocation: Improving survival from an often fatal condition. Can J Surg. 2001;44:412-420.
- Pang D, Nemzek WR, Zovickian J. Atlanto-occipital dislocation: Part 1–normal occipital condyle-C1 interval in 89 children. Neurosurgery. 2007;61:514–521.
- Pang D, Nemzek WR, Zovickian J. Atlanto-occipital dislocation–part 2: The clinical use of (occipital) condyle-C1 interval, comparison with other diagnostic methods, and the manifestation, management, and outcome of atlanto-occipital dislocation in children. Neurosurgery. 2007;61: 995–1015.
- Gire JD, Roberto RF, Bobinski M, et al. The utility and accuracy of computed tomography in the diagnosis of occipitocervical dissociation. Spine J. 2013;13:510–519.
- Junewick JJ, Meesa IR, Luttenton CR, Hinman JM. Occult injury of the pediatric craniocervical junction. Emerg Radiol. 2009;16:483-488.