Knee Pain: MRI of Sports Injuries
Images
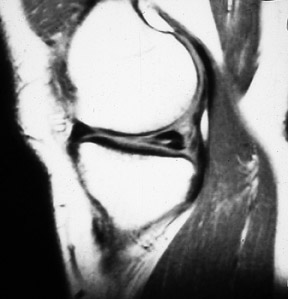
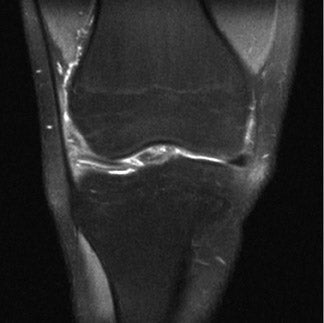
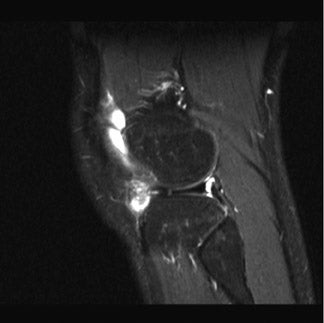
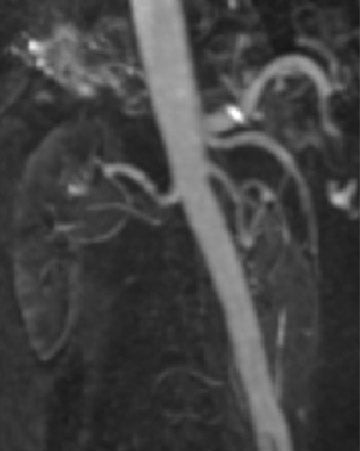
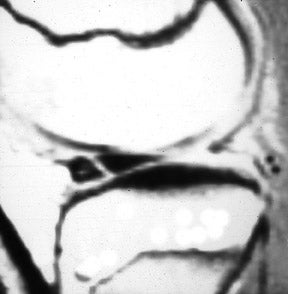
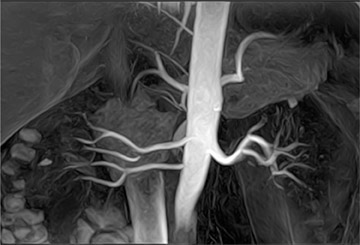
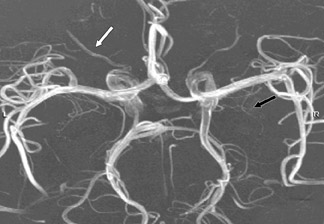
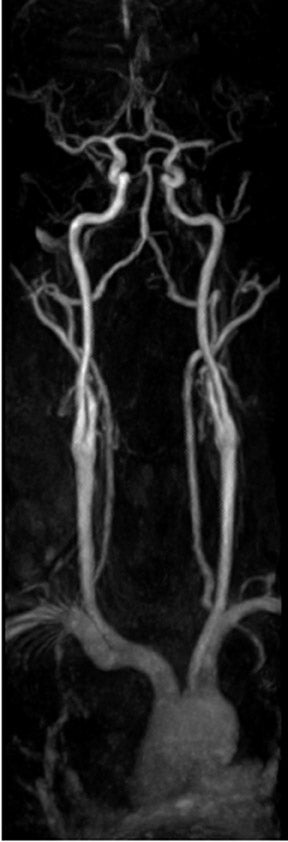
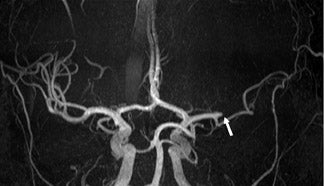
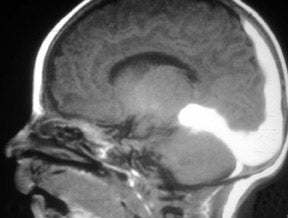
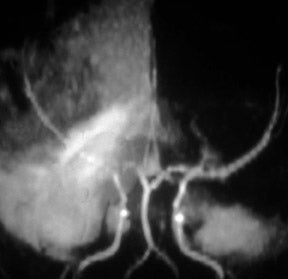
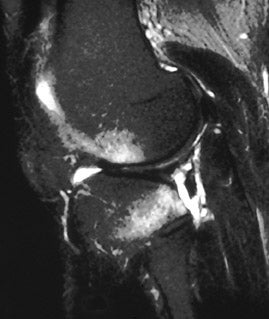
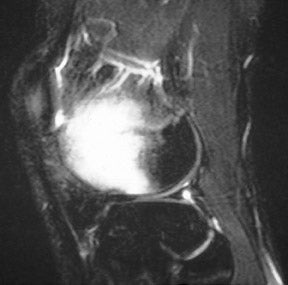
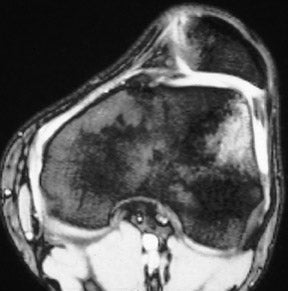
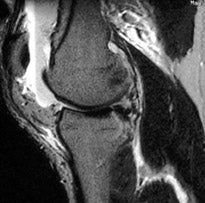
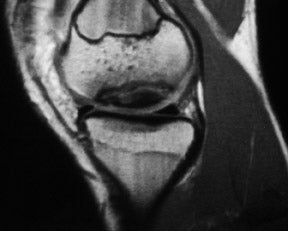
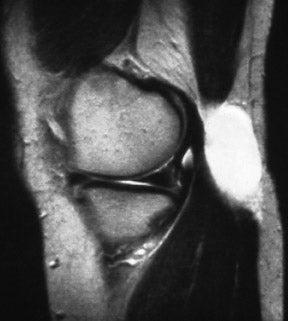
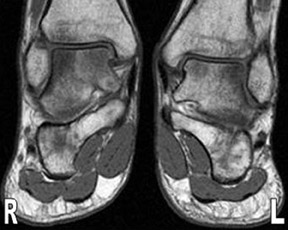
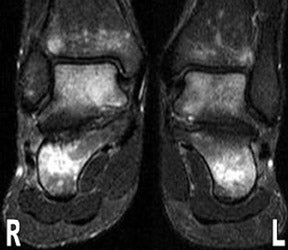
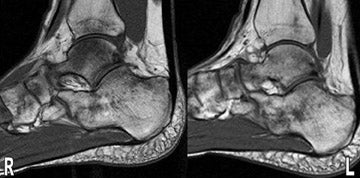
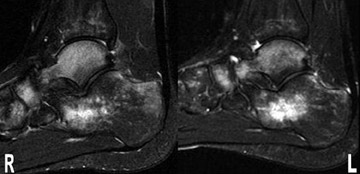
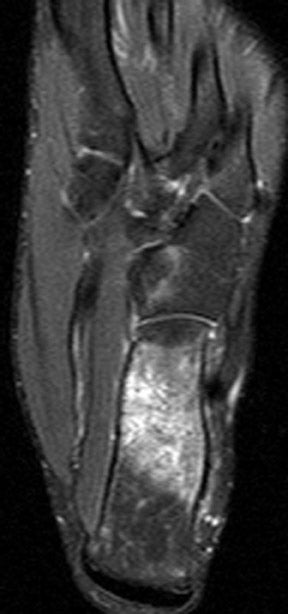
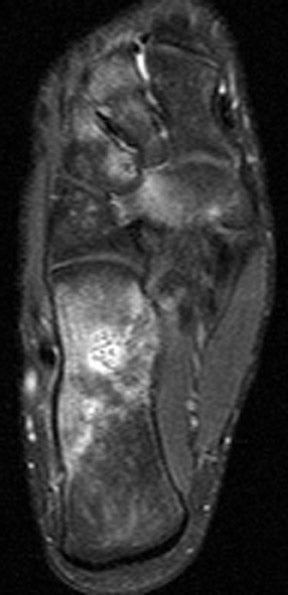
Dr. Bradley is a Professor of Radiology and the Chairman of the Department of Radiology, University of California San Diego Medical Center, San Diego, CA.
This article has been adapted from a newsletter publication: Bradley WG. Knee pain. Applied Imaging: Applications in MRI. 2001;2(1):1-4. Material reprinted with permission of Anderson Publishing, Ltd.
Although plain radiographic films have traditionally been the first diagnostic imaging study performed in the evaluation of the painful knee, today they are useful only for evaluating joint space narrowing, alignment, and major trauma. Over the past 20 years, magnetic resonance imaging (MRI) has become the premier, first-line imaging study that should be performed in the evaluation of the painful knee. This article in this supplement to Applied Radiology describes the MR evaluation of internal derangement of the knee (tears of the menisci and the cruciate and collateral ligaments), osteochondral abnormalities (chondromalacia, osteoarthritis, and osteochondral defects), synovial cysts, and bone bruises.
Internal derangement
In order to evaluate internal derangement adequately, the knee must be scanned in the axial, coronal, and sagittal planes using thin sections (3-mm thick) with a combination of T1- and T2-weighted techniques as well as at least one 5-mm thick short tau inversion recovery (STIR) or T2-weighted image with fat suppression. 1
The anterior and posterior horns of the medial and lateral menisci appear as wedge-shaped, low-intensity structures pointing toward each other on sagittal and coronal views. 2 High signal within the meniscus, extending to the superior or inferior articular surface (Figures 1 and 2), constitutes a meniscal tear. This is best seen on images with narrowed "meniscal" windows (Figure 1). A caveat: In patients who have had prior knee surgery, it can be difficult to distinguish an old scar from an acute tear without injecting gadolinium into the joint space. Internal meniscal signal that does not extend to the articular surfaces or that extends only to the base of the "wedge" does not constitute a tear. In children, this represents the normal vascular pedicle of the meniscus; in adults, it represents myxoid degeneration, ie, normal aging.
Horizontal-oblique tears are considered the results of myxoid degeneration and are called "degenerative" tears. Those oriented in the vertical direction, which involve both the superior and inferior articular surfaces, are considered "traumatic" tears. 2 Tears do not need to be seen in both the sagittal and coronal planes, as they are only visualized when they are perpendicular to the plane of the section. In fact, tears running oblique to both the coronal and sagittal planes may well be visualized only by a notch on the articular surface. This "notch sign" 3 has served us very well over the last decade in diagnosing subtle meniscal tears (Figure 3). Truncation of the tip of the meniscus represents a "parrot-beak" tear (ie, fraying of the free margin of the meniscus).
Occasionally, a large piece of meniscal fibrocartilage will become detached from its capsular attachment and flip back-to-front or centrally into the intercondylar notch. Clinically, these patients present with a locked knee. These "bucket-handle" or "flap" tears 4 are recognized on the basis of two findings: An absence of the normal meniscus in its expected position and abnormal meniscal-intensity material in an unexpected location (Figure 4). Bucket-handle tears flipping from posterior to anterior appear as 2 arrowheads pointing posteriorly in the position of the anterior horn of the meniscus, with nothing at all in the expected position of the posterior horn. This pattern is more common for bucket-handle tears of the lateral meniscus. Tears flipping from the periphery into the intracondylar region of the knee appear as an extra structure adjacent to the cruciate ligaments, with an absence of meniscal signal in the expected position of the posterior horn. Typically, this pattern is seen with bucket-handle tears of the medial meniscus. When the displaced meniscal fragment lies parallel to the posterior cruciate ligament (PCL), it may produce the "double PCL sign." 4
The anterior cruciate ligament (ACL) may be seen on sagittal images (Figure 5); however, it is always seen as an upside-down "V" on coronal images (Figure 6). The ACL is lateral to the rounded dark PCL. (If the coronal plane does not contain the fibula to indicate the lateral aspect, the adductor tubercle on the medial aspect of the femur [Figure 6] can usually be seen.) The ACL is most commonly injured by valgus stress to the knee while the leg is in external rotation, eg, a "clipping" injury in football or getting hit laterally coming down from a layup in basketball. 5-7 MR studies performed shortly after the injury will fail to reveal the separate fascicles of the ACL, which are replaced by an ill-defined mass of tissue that is bright on T2-weighted images (reflecting edema). MR imaging performed much later in a chronically "ACL-defi-cient" knee also fails to show the well-defined fibers of the ACL (Figure 7), but lacks the T2 hyperintensity of an acute tear.
With the medial knee joint distraction resulting from valgus stress, the medial collateral ligament (MCL) as well as the medial meniscus may also be torn (this is known as O'Donoghue's triad). Acute injuries of the MCL are best evaluated in the coronal plane, revealing discontinuity of the normally black line (Figure 6) that runs along the medial aspect of the knee. With acute injuries, there is also a linear fluid collection (which is bright on a T2-weighted image) superficial to the torn MCL (Figure 8). This is usually associated with reticulation of the overlying subcutaneous tissues, indicating soft tissue swelling. Chronic MCL tears result in thickening without associated fluid.
In addition to the medial distraction resulting from valgus stress during an ACL injury, there is lateral impaction. This may lead to bone marrow contusions of both the femoral and tibial surfaces of the lateral compartment (Figures 8 and 9). At the time of the MR examination, the leg is in a neutral position (rather than being externally rotated as it was at the time of ACL injury); therefore, the "kissing contusions" are not directly opposite each other. 8
Bone marrow contusions (also called "bone bruises" and "trabecular fractures") cannot be seen on plain films and can be a major source of pain. 8 They should be suspected in the patient with an acutely injured knee without a meniscal or ligamentous injury in the absence of plain-film findings of fracture. Because their water content is higher than that of adjacent fatty bone marrow, these contusions are best detected on fat-suppressed T2-weighted images. 9 At high field, we use fat-saturated T2-weighted fast spin-echo images and, at any field strength, STIR images.
In a study of 73 consecutive patients with suspected sports-related internal derangement of the knee, fat-saturated T2-weighted fast spin-echo imaging detected ≥1 bone bruises in 30% of patients in whom lesions were not seen on conventional MRI. 9
In evaluating bone marrow contusions, it is important to determine if they extend to the articular surface, as this is usually an indication that the athlete must rest for 30 days. Continued activity in this setting could convert a bone bruise with intact cartilage (type I) (Figure 9) to one in which the cartilage becomes disrupted (type II) (Figure 10A), leading to early osteoarthritis. 8
Bone bruises can be seen whenever abnormal forces are applied to normal tissue or essentially normal forces are applied to weakened tissue, eg, osteoporotic bones in middle-aged "weekend warriors." Thus, milder versions of the same forces that lead to tibial plateau fractures will lead to bone marrow contusions, resulting in normal plain films and high signal on STIR or fat-saturated T2-weighted fast spin-echo images.
An interesting pattern of bone marrow contusion can be seen with traumatic dislocation of the patella. After the patella dislocates laterally when the knee is in hyperextension, the quadriceps contracts, jamming the medial patellar facet against the lateral femoral condyle, resulting in bone marrow edema on both surfaces (Figure 10B). Usually, this is also associated with disruption of the black line of the medial retinaculum, which, acutely, may have associated bleeding or edema. 10 Such injuries are seen in football and basketball players who come down on a hyperextended knee.
Bone marrow contusions or fractures associated with cortical disruption can leak marrow into the joint space, leading to a lipohemarthrosis. Since sagittal images are acquired with the patient supine, fat rises to the top, joint fluid is in the middle, and intact red cells are at the bottom (Figure 11).
Lesions of the PCL and the lateral collateral ligament are much less common than their anterior counterparts. The PCL is recognized as a dark C-shaped structure on sagittal images and is visualized as a dark, rounded structure seen en face on coronal images (Figure 6). 1 The coronal images are best for visualizing partial PCL tears (which show increased signal in a normally black structure), while the sagittal view may better illustrate complete disruption (Figure 8).
Osteochondral abnormalities
The earliest of the osteochondral abnormalities is simple cartilaginous thinning, as seen in chondromalacia patellae 11 and osteoarthritis. Although cartilage can be evaluated on routine MR sequences, we tend to use a higher resolution (512 × 512 matrix) thin-slice (1.5-mm sections) gradient-echo technique when subtle abnormalities of cartilage are being investigated.
With advancing osteoarthritis, the articular cartilage is completely denuded and subchondral sclerosis can be seen as low intensity on all sequences. With continued bone-on-bone irritation, fibrovascular reaction can lead to bone marrow edema, which appears bright on STIR or fat-saturated T2-weighted fast spin-echo images.
Osteochondral defects arise from varying degrees of traumatic and ischemic insults to the articular surface of the knee (Figure 12). Early osteochondral defects appear as localized areas of rounded bone marrow edema without frank fluid collection. 12 With advancing disease, a ring of frank fluid can be seen surrounding the osteochondral defect, although the overlying articular cartilage remains intact. Subsequently, the articular cartilage also becomes disrupted and, eventually, the fragment separates, becoming "free" within the joint space, leaving a "divot" behind.
Cystic disease of the knee
Synovial cysts are very common in the knee given the large number of synovium-lined surfaces. The most common is a Baker's cyst (Figure 13), which generally results from a knee effusion that forces synovium through the space between the tendons of the medial head of the gastrocnemius and the semimembranosus. 13 Baker's cysts may persist following resolution of the knee effusion and are a cause of medial joint line tenderness.
Another, less common cause of medial joint line tenderness is anserine bursitis, 14 which is inflammation of the synovium of the pes anserinus (the confluence of tendons from the sartorius, gracilis, and semitendinous muscles).
Synovial cysts can be seen in conjunction with torn menisci, more commonly involving the lateral than the medial meniscus. These cysts appear to result from a ball valve mechanism that allows fluid to flow from the torn meniscus into the cyst but not in the opposite direction. 15
Intramedullary abnormalities of the knee
Excluding bone bruises, most intra-medullary abnormalities of the knee can be seen on plain films. Tumors (eg, osteosarcomas and metastases) are rare and result in focal destruction of bone with an associated mass. Bone infarcts appear as metaphyseal rings of hyperintensity on T2-weighted images, corresponding to the peripheral ring of calcification noted on plain films. Enchondromas (which are usually asymptomatic) have a spiculated appearance on T2-weighted images, corresponding to the "popcorn" calcification noted on plain film. Frank fractures appear as linear areas of hyperintense fluid on T2-weighted images, with surrounding less intense areas of bone marrow edema. Frequently, cortical disruption can be seen on the tomographic, thin MR slices, but cannot be appreciated on projectional plain films.
Conclusion
Over the past 2 decades, MRI has become the preferred imaging technique for the evaluation of the painful knee following a sports injury. It can detect soft tissue abnormalities (meniscal and cruciate/collateral ligament tears) and fractures that cannot be detected by plain film. These findings are critical for the therapeutic decisions to be made by the orthopedic surgeon.
REFERENCES
- Crues JV III, Manji SA, Shellock FG: Knee. In: Stark DD, Bradley WG, eds. Magnetic Resonance Imaging. 3rd ed. St. Louis, MO: Mosby; 1999:811-871.
- Crues JV III, Mink J, Levy TL, et al. Meniscal tears of of the knee: Accuracy of MR imaging. Radiology. 1987;164:445-448.
- Davis SJ, Teresi LM, Bradley WG, Burke JW. The "notch" sign: Meniscal contour deformities as indicators of tear in MR imaging of the knee. J Comput Assist Tomogr. 1990;14:975-980.
- Wright DH, De Smet AA, Norris M. Bucket-handle tears of the medial and lateral menisci of the knee: Value of MR imaging in detecting displaced fragments. AJRAm J Roentgenol. 1995;165:621-625.
- Gentili A, Seeger LL, Yao L, Do HM. Anterior cruciate ligament tear: Indirect signs at MR imaging. Radiology. 1994;193:835-840.
- Kaplan PA, Walker CW, Kilcoyne RF, et al. Occult fracture patterns of the knee associated with anterior cruciate ligament tears: Assessment with MR imaging. Radiology. 1992;183:835-838.
- Robertson PL, Schweitzer ME, Bartolozzi AR, Ugoni A. Anterior cruciate ligament tears: Evaluation of multiple signs with MR imaging. Radiology.1994; 193:829-834.
- Lynch TC, Crues JV III, Morgan FW, et al. Bone abnormalities of the knee: Prevalence and significance at MR imaging. Radiology.1989;171:761-766.
- Kapelov SR, Teresi LM, Bradley WG, et al. Bone contusions of the knee: Increased lesion detection with fast spin-echo MR imaging with spectroscopic fat saturation. Radiology. 1993;189:901-904.
- Spritzer CE, Courneya DL, Burk DLJr, et al. Medial retinacular complex injury in acute patellar dislocation: MR findings and surgical implications. Am J Roentgenol. 1997;168:117-122.
- Noyes FR, Stabler CL. A system for grading articular cartilage lesions at arthroscopy. Am J Sports Med.1989;17:505-513.
- Deutsch AL, Mink JH, Shellock FG. Magnetic resonance imaging of injuries to bone and articular cartilage: Emphasis on radiographically occult abnormalities. Orthop Rev. 1990;19:66-75.
- Lee KR, Cox GG, Neff JR, et al. Cystic masses of the knee: Arthrographic and CT evaluation. AJR Am J Roentgenol. 1987;148:329-334.
- Hall FM, Joffe N. CT imaging of the anserine bursa. AJR Am J Roentgenol. 1988;150:1107-1108.
- Ferrer-Roca O, Vilalta C. Lesions of the meniscus. Part I: Macroscopic and histologic findings. Clin Orthop Relat Res.1980;146:289-300.
Prepared by Peyman Borghei, MD, Research Fellow of Musculoskeletal Radiology; Maryam Golshan Momeni, MD, Research Fellow of Musculoskeletal Radiology; and Jamshid Tehranzadeh, MD, Professor of Radiology and Orthopaedics, the Departments of Radiological Sciences and Pathology, University of California (Irvine), Irvine, CA.
CASE SUMMARY
A 19-year-old male kickboxer presented with a 3-week history of bilateral foot pain. Physical examination revealed general tenderness of both feet. His pain worsened while walking and was aggravated by kicking. He had taken nonprescription analgesics to alleviate his pain. Magnetic resonance imaging (MRI) of the foot was performed for further evaluation (Figures 1 through 6) and established the definite diagnosis.
IMAGING FINDINGS
MR images show diffuse bone marrow edema of multiple tarsal bones, of both distal tibias, and of the right fibula. There are lines of low signal intensity on the T1-weighted images (T1WI) and surrounding diffuse marrow edema on the selective partial inversion recovery (SPIR) images in both calcanei, tali, and navicular bones. These are compatible with fatigue fractures (Figures 1 through 4). In addition, fatigue fractures of the left cuboid (Figure 5) and bone contusions of the right lateral and middle cuneiforms are seen on the axial MR image (Figure 6).
DIAGNOSIS
Multiple tarsal bone fatigue fractures and bone contusions (Kickboxer's feet)
DISCUSSION
Stress-related injury to bone is common in athletes. These injuries comprise a wide spectrum ranging from bone edema to fatigue fracture and are caused by intense and repetitive activity without adequate periods of rest. 1 Some fatigue fractures have sports specificity, such as those in the coracoid process in trap shooters, 2 those in the isthmus of the lumbar spine in divers, 3 the tibial stress injury in ballet dancers, and the metatarsal stress in marching soldiers. 4
In kickboxing, throwing punches with both the upper and lower extremities is permitted, and trauma is not confined to a specific limb. Therefore, injury to any or every organ can be anticipated. Gartland et al 5 reported that lower limb injuries were second only to head and neck injuries in a group of Thai kickboxers. This case is a kickboxer who complained of 3 weeks of pain in both feet and who was diagnosed with multiple tarsal bone fatigue fractures and contusions by MRI. A review of the literature revealed no reports of multiple tarsal bone contusions or fatigue fractures due to a specific sports injury. However, there are reports of bone contusion or fracture in the talus or cuneiform of runners and gym-nasts. 3,6
In our case, the radiographs of the feet were normal. The conventional radiograph should always be the initial study in this clinical setting, although demonstrable findings may be minimal or absent, as in this case. Radiography of fatigue fractures shows a linear area of sclerosis within the cancellous marrow cavity, which is usually perpendicular to the long axis of the bone and is often seen in the chronic phase of the process. MRI findings of fatigue fractures are highly sensitive and are usually evident several weeks before any radiographic manifestations. The sensitivity and specificity of MRI for stress-related injuries has been reported to be 60% to 100%. 7 Acute bone contusions show reticular hypointense areas on T1WI that are hyperintense on T2WI and on fat-suppressed T2WI and are due to microfractures of the trabecular bone and edema or hemorrhage of the bone marrow. These bone contusions usually resolve within 8 to 12 weeks if the causal activity is stopped. Continuous stress, however, may lead to a complete fracture and can be significant in areas such as the femoral neck.
A condition known as "stress response" precedes the fatigue fracture and shows edema, hyperemia, and osteoclastic activity. In this situation, MRI shows abnormal signal intensity similar to a bone contusion. If the stress persists and a fracture develops, MRI will show an irregular, hypointense line within the area of edema and hyperemia. Bone marrow abnormalities are best evaluated with fat-suppression techniques such as short tau inversion recovery (STIR) or selective partial inversion recovery (SPIR), 8 and these imaging protocols should be used in all cases of suspected stress-related injury.
CONCLUSION
A 19-year-old kickboxer presented with a 3-week history of bilateral feet pain. MRI showed multiple tarsal bone fatigue fractures and diffuse bone marrow contusion. We have termed these findings Kickboxer's feet , since the individual developed these injuries from this activity.
In all cases of suspected stress injury, a high index of clinical suspicion is necessary, especially in athletes who are involved in martial arts. In these cases, MRI should be performed expeditiously, as radiography is often not very revealing. Persistent foot pain related to loading activity should alert the clinician to the possibility of stress injury or bone contusion.
REFERENCES
- Iwamoto J, Takeda T. Stress fractures in athletes: Review of 196 cases. J Orthop Sci.2003;8:273-278.
- Boyer DW Jr. Trap shooter's shoulder: Stress fracture of the coracoid process. Case report. J Bone Joint Surg Am.1975; 57: 862-864.
- Rossi F, Dragoni S. The prevalence of spondylolysis and spondylolisthesis in symptomatic elite athletes: Radiographic findings. Radiography.2001;7:37-42.
- Tehranzadeh J, Serafini A, Pais MJ. Avulsion and Stress Injuries of the Musculoskeletal System. Basel, Switzerland: Karger Publishing Co.; 1988.
- Gartland S, Malik MH, Lovell ME. Injury and injury rates in Muay Thai kick boxing. Br J Sports Med.2001;35;308-313.
- Pitsis GC, Best JP, Sullivan MR. Unusual stress fractures of the proximal phalanx of the great toe: A report of two cases. Br J Sports Med.2004; 38:e31-e33.
- Hodler J, Steinert H, Zanetti M, et al. Radiographically negative stress related bone injury. MR imaging versus two-phase scintigraphy. Acta Radiol.1998;39:416-420.
- Masala S, Fiori R, Marinetti A, et al. Imaging the ankle and foot and using magnetic resonance imaging. Int J Low Extrem Wounds. 2003; 2:217-232.