Digital X-Ray and PACS (2005)
Images
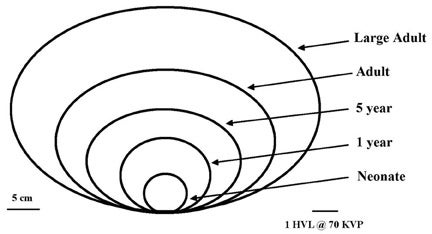
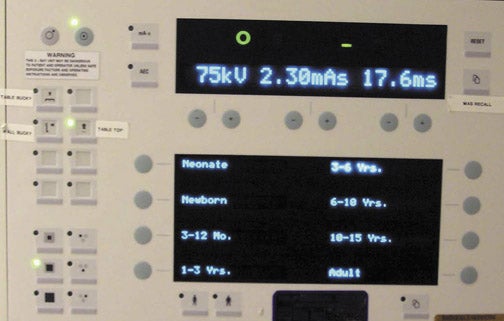
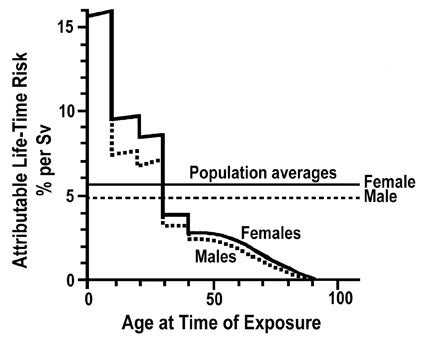
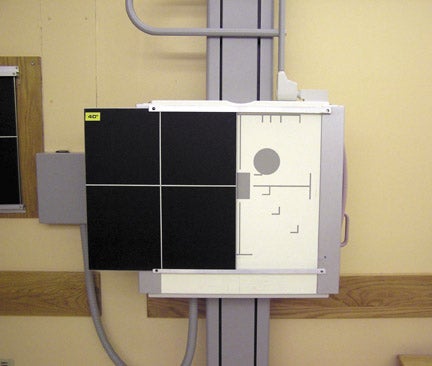
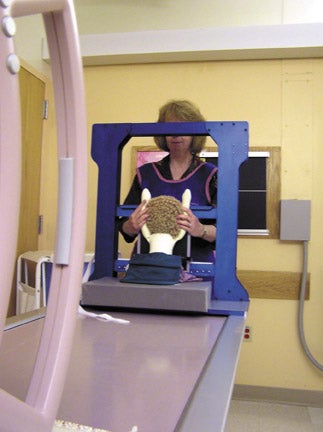
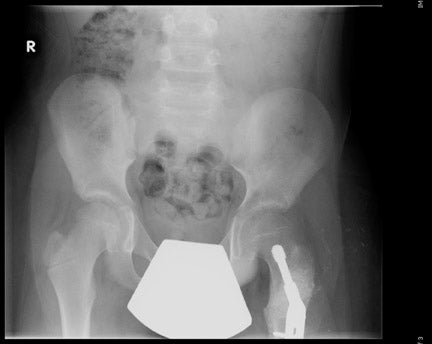
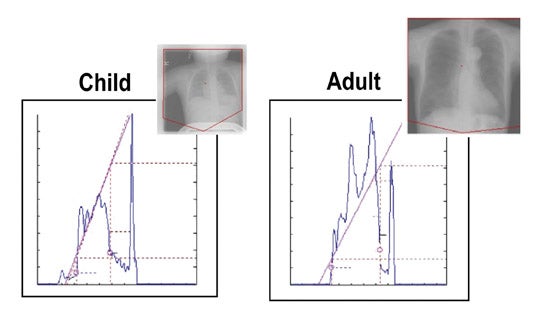
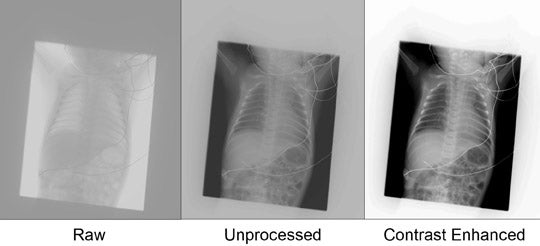
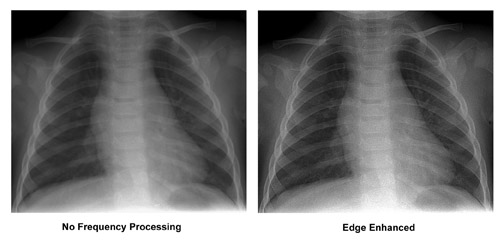
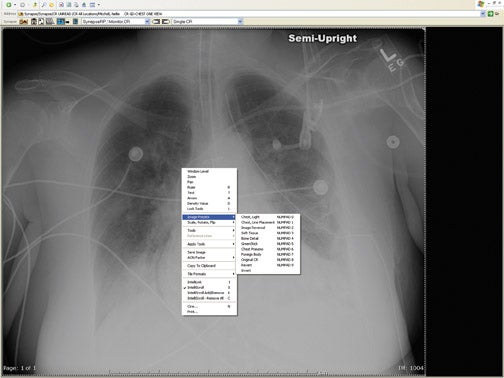
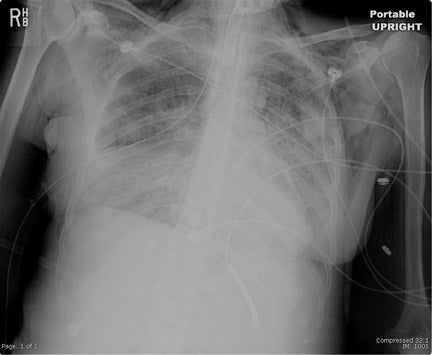
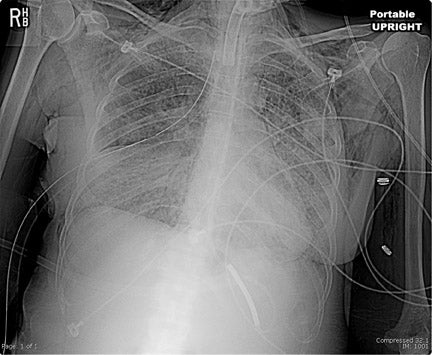
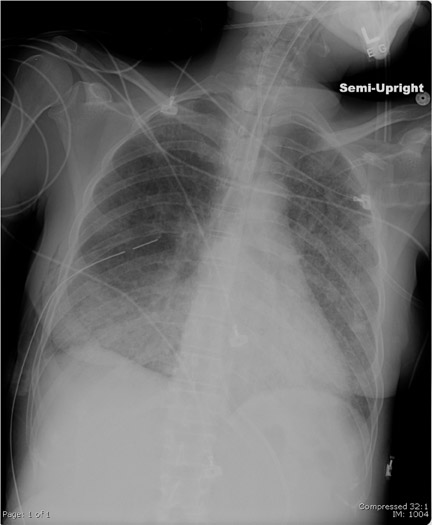
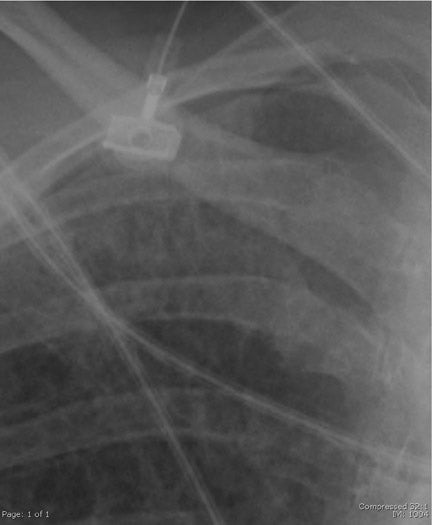
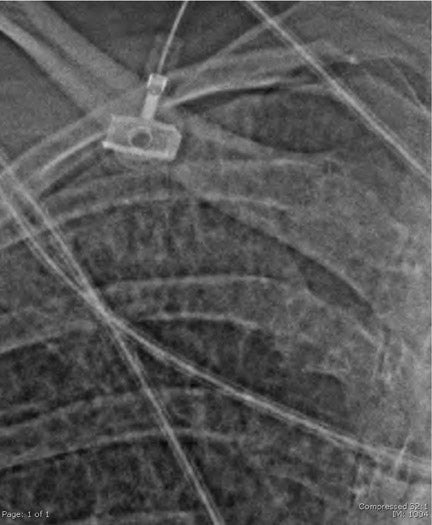
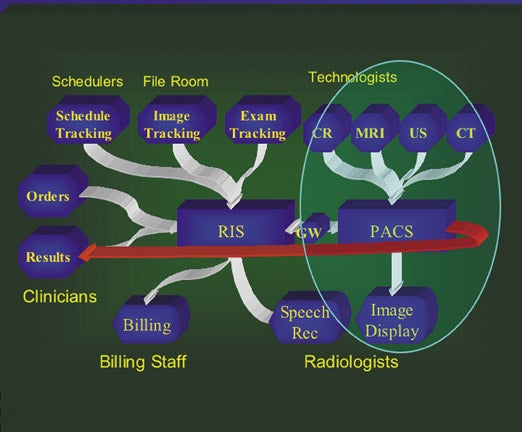
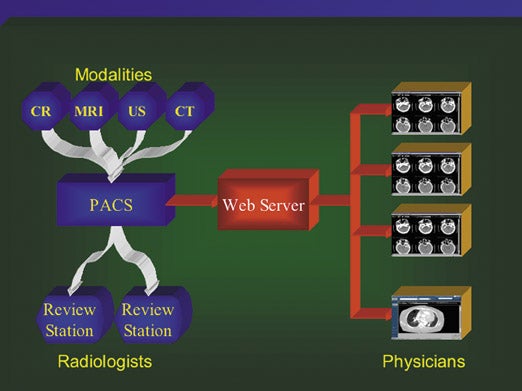
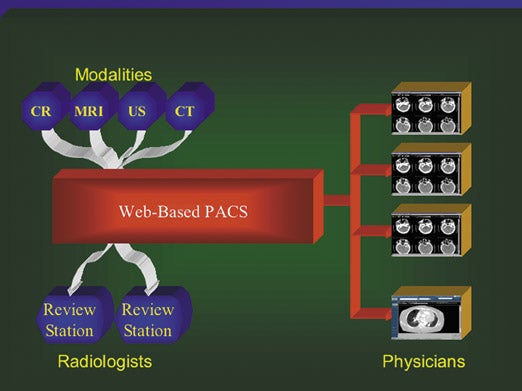
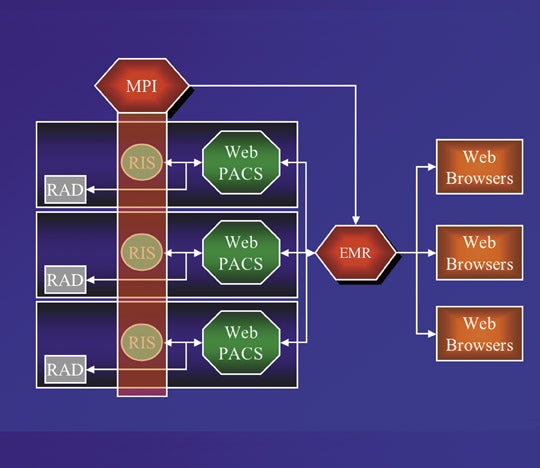
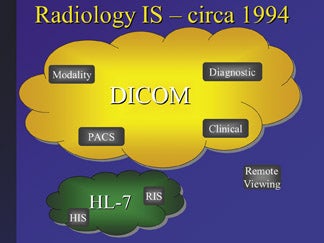
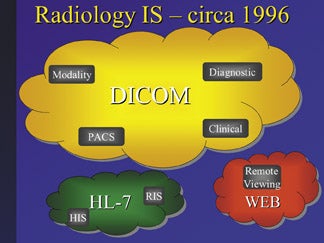
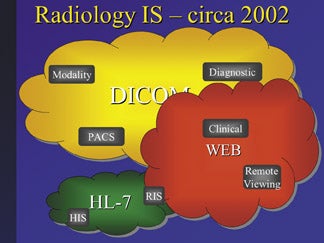
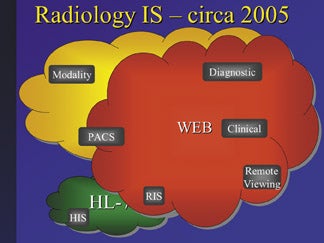
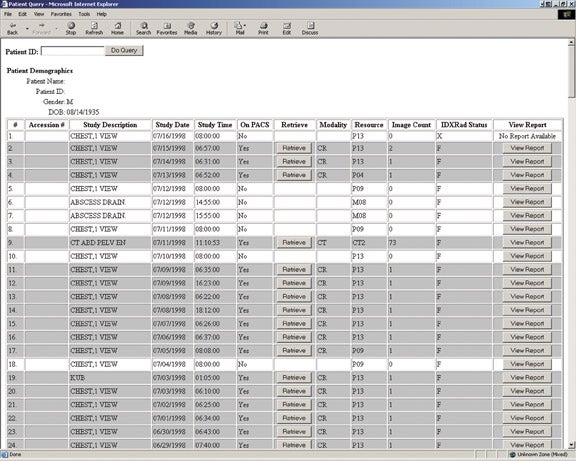
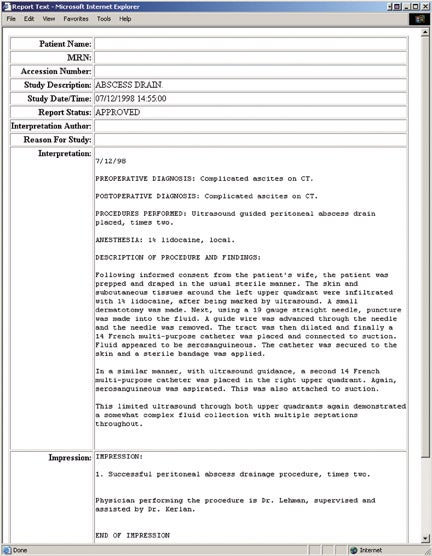
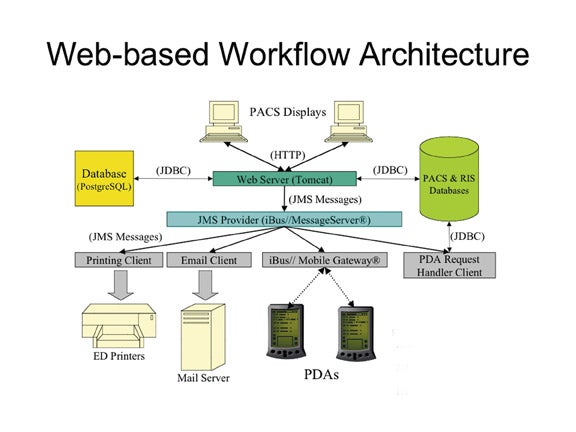
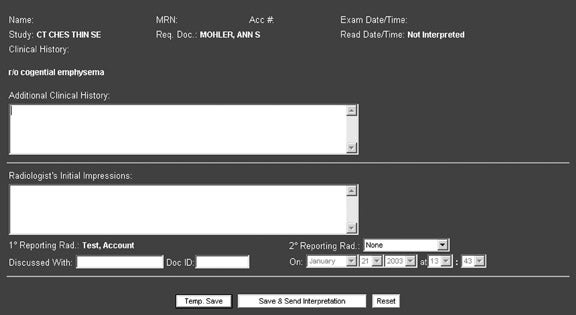
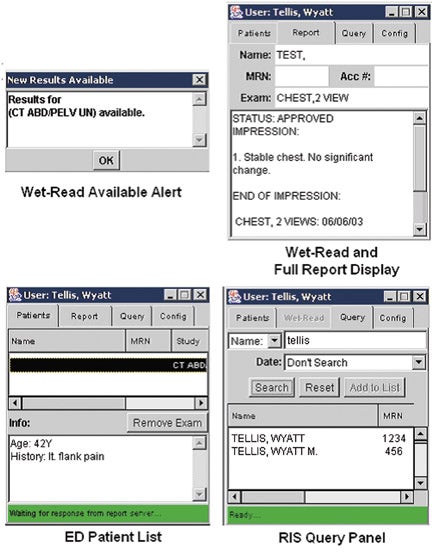
Dr. Staab is a Professor of Nuclear Medicine, Wake Forest University, Winston-Salem, NC. He is also a member of the editorial board of this journal and served as the Supplement Editor of this supplement.
The advent of digital imaging and the incorporation of related information technologies into the everyday practice of radiology have revolutionized our medical environment. Although many publications dealing with these rapidly changing informational systems are currently available in various journals and other media, I find it difficult to keep up with all the important advances that affect my specialty practice of radiology. This must be even more demanding for the general radiologist. For this reason, a timely review by experts geared to the practicing radiologist is offered in this supplement to Applied Radiology . Because of the brevity of this publication and the need to balance an in-depth look at the technology with the constraints of confining the remarks to a succinct presentation, only a few areas can be covered.
Drs. Berman and Dreyer call attention to the wide variation of information technologies currently available and offer their assessment of the current state of the art. These individuals have had a good deal of experience and have devoted considerable effort to advancing this field. Most will consider their state-of-the-art implementations to be future goals. It is exciting to know that prototypes of these advanced systems are actually in place and are already demonstrating productivity and patient care benefits.
Dr. Mezrich relives the changes in the practice of radiology brought about by advances in imaging technology and picture archiving and communication systems (PACS). He raises some serious issues that we are all facing and, most importantly, outlines what we must do to remain relevant. I encourage the interested reader to re-read this article. Each time I read it I came away with a better understanding of what Dr. Mezrich is trying to convey to us.
Radiation dose to children has been a topic for much discussion. Careful attention to details when using CR for image capture can assure quality imaging with reasonable exposure dosage. This is moderately challenging. Drs. Strauss and Poznauskis provide a blueprint of the steps needed to optimize the system. It is not necessary for the busy practicing radiologist to optimize the equipment; that is the task of a physicist working with the vendor. It is necessary for the radiologist to understand what is expected in a good quality study. After all, a well-trained radiologist is the final quality-control check. This is clearly one of the value-added aspects of having radiologists in charge of the imaging department.
Another aspect of computed tomography imaging is the establishment of reading protocols as discussed by Dr. Matalon. He points out that such protocols have largely been ignored. His discussion is provocative. Could we improve our productivity and accuracy with more attention to the details he lists?
Two additional papers address the use of Web-based technologies to link multiple disparate information systems and to globally communicate text and image information. The first by Dr. Dreyer is a short, concise and quite complete summary of the evolution of Web strategies to date. He speculates on how future development of these systems will affect the practice of radiology.
Finally, Drs. Arvin and Tellis describe the underpinnings of the Web-based customized workflow that most of the PACS vendors are using today to optimize our environments. The management of medical information and attention to improving the workflow by using these systems is an on-going pursuit.
Dr. Dreyer is Vice Chairman of Radiology and Corporate Director of Enterprise Imaging, Harvard Medical School, Massachusetts General Hospital, Boston, MA. Dr. Berman is a Director of iAtrogenix, Inc., Tucson, AZ.
With experience in the academic, private-practice, and vendor communities, we have the pleasure of visiting numerous hospitals and their radiology departments throughout the world. We have evaluated myriad radiology information systems (RIS) and picture archiving and communication systems (PACS) to explore their effectiveness and frustration points. It has become apparent to us that PACS and RIS implementations in hospitals today vary widely in their features and functionality.
This variability is due to a host of factors, many of which center around capital budgets and hospital finances, the ages of legacy RIS and PACS, the degree of PACS and healthcare information systems deployment, network infrastructure robustness, level of information technology (IT) integration and support, data-storage design and management, and the proclivity for technology adoption culture within a healthcare institution or enterprise.
In a highly competitive healthcare service providers market, the impact of PACS and RIS to improve the management and access of patient information and to increase productivity, efficiency, and job satisfaction levels among clinicians and support staff can place a radiology department and/or imaging center at a tremendous advantage or disadvantage to its competitors. This is being documented anecdotally in radiology and informatics publications.
Healthcare facilities are wise to adopt the attitude that change is good. Both RIS and PACS should be kept current with the purchase of software upgrades. With the maturation of Digital Imaging and Communications in Medicine (DICOM) and Health Level 7 (HL-7) standards, innovative products from third-party vendors can be integrated more easily and economically.
PACS technology is in a continuous stage of evolution. We consider the following features to be state-of-the-art. If your department has not yet implemented PACS or RIS, you might use this list to differentiate offerings by various vendors. If you are already a PACS or RIS user, we recommend that you use this list to evaluate your current vendor's offering and to see where you stand compared with the leading-edge technologies that are materializing in 2005.
Advanced visualization
Considered novel several years ago, 3-dimensional (3D) and volume visualization has become mainstream. Its adoption is out of necessity. Massive amounts of data are streaming from multidetector computed tomography (MDCT), cardiac CT, positron emission tomography (PET)/ CT, high-Tesla magnetic resonance imaging (MRI), and 4-dimensional (4D) ultrasound modalities. However, the use of independent 3D processing systems external to a PACS is fraught with problems. The workflow efficiency of radiologists is hindered by the necessity of moving between PACS and dedicated 3D workstations. Proprietary hardware and expensive software prevent its wide deployment, even within the radiology department itself. Even nearly state-of-the-art thin-client solutions allowing for generic PC access to a centralized advanced visualization system require the addition of yet another redundant array of inexpensive disks (RAID) system in the ever-expanding medical image storage explosion.
There was a widespread introduction of advanced visualization software integrated into PACS workstations in 2004. The capabilities vary greatly. With some, the interpretation process can be slow. In addition, many of the basic PACS features are not available during the use of 3D analysis. Try, for example, to use hanging protocols to compare a "with and without" contrast multiplanar reconstruction series to a similar prior examination.
State-of-the-art institutions have optimized their use of 3D to such an extent that it represents a significant part of their daily volume. Such advanced visualization exists today as an integral part of some PACS vendors' offerings. Users of these systems have seamless access to complex 3D visualization techniques from any workstation. But beware, there is a significant increase in radiologist productivity when using 3D functionality designed into a PACS versus a 3D system that has simply been integrated with a PACS. A good test for seamless integration is to confirm that PACS 3D functionality is as widely available, and as easy to use, as window/level or zoom.
Remote interpretation
Whether it occurs in the form of nighthawk services, home teleradiology, or covering a department while visiting another part of the world, remote reading has become a radiological necessity. In our experience, the ability of an institution to perform remote interpretation is becoming essential for recruitment and retention of radiologists.
Efficient remote interpretation is completely dependent upon the PACS architecture of an institution or an enterprise. Web-based technologies, computer hardware and storage advancements, and global proliferation of high-speed network accessibility have revolutionized diagnostic image distribution. State-of-the-art systems exist in which remote interpretation is identical to that of interpretation in the reading room. Prior images, reports, patient clinical information, and 3D are as easy to access from a hotel room in Hawaii as they are from a reading room in the hospital.
Such state-of-the-art systems can now be easily integrated with a PACS and a RIS. A user is no longer limited to deploying his/her PACS vendor's Web-viewing product. In today's world, a hospital with remote image distribution functionality that is limited to clinical review functionality is obsolescence-challenged.
Remote order entry and decision support
Today we can schedule trips and order plane tickets on the Web, finding the best prices and shortest times, all without talking to a single person. So why is it that we continue to burden our referring physicians with inefficient, manually intensive examination ordering processes? While most RIS require ordering physicians to call or fax schedulers to negotiate through the ordering process, alternative systems are available that make this process as easy as booking a flight online.
State-of-the-art radiology examinations ordering and scheduling is done over the Web. Smart RIS have the flexibility of offering the soonest available times in one or multiple locations, of scheduling multiple examinations for the same day, and of advising of the order in which multiple examinations should be scheduled. They activate prequalification processes within the RIS and provide electronic forms for completion. Just as speech recognition has optimized the reporting process, remote order entry (ROE) has streamlined another laborious and error-prone process.
With the advent of Web ordering comes the opportunity for additional onboard clinical knowledge delivery. New radiology decision support (RDS) is providing clinicians with up-to-date clinical knowledge regarding appropriate examination usage. Many clinicians today cannot keep abreast of state-of-the-art diagnostic procedures that may be more effective than a familiar alternative. Or conversely, they may be so enamored with or feel so protected by utilizing the newest solutions that these high-cost procedures are ordered inappropriately. Radiology decision support systems based on the American College of Radiology's (ACR) appropriateness criteria are rapidly guiding clinicians through the morass of indications and imaging alternatives, directing them toward the most appropriate examination given their patient's clinical state.
When a clinician orders a study, a selection of clinical indications is presented. As soon as indications are selected, an appropriateness criterion rating is made, as well as recommendations for substitute or supplemental procedures. Radiology decision support systems not only educate clinicians, but they can also reduce the number of inappropriate studies performed and may be used to justify recommended procedures to medical insurance companies for rapid prequalification and approval.
Advanced reporting and artificial intelligence
We all do it. We scour through hundreds of images looking for intricate anatomy and detailed pathology in a variety of planes and reconstructions to render a diagnosis. Then, due to the limitations of many systems, most of us transcribe our visual findings into a narrative report. Wouldn't it be great if we could record and share this effort by easily capturing concise visual cues to present our findings to our ordering physicians? Well, a limited few are actually using state-of-the-art systems to do exactly that.
Tight integration of PACS and reporting systems have allowed for the seamless integration of text and images. While most of us still use human transcription to convert spoken words into computer text, others have successfully mastered speech recognition (SR), drastically decreasing the production costs and delivery time of critical diagnostic results. Recent advances in speech recognition and natural language understanding (NLU) are bringing even greater accuracy to the reporting process.
State-of-the-art systems are providing instantaneous structure to SR-generated reports by employing on-the-fly NLU. The result is a reporting application that understands what the radiologist is saying while he or she is dictating. The power that this can bring to the interpretation process is incredible.
One such example is the ability to automatically reference medical information during the interpretation process and add it directly to the resulting report. Because state-of-the-art reporting systems can now recognize what a radiologist is saying, they can respond automatically with critical information that might otherwise be unavailable to the interpreting radiologist. Such information might include differential diagnosis, disease details, or recommended follow-up processes. Similarly, if the name of an anatomical part evades memory, a radiologist can click directly on a displayed reference image and be enlightened instantly. Differential diagnosis, detailed pictorial atlases, teaching file cases, or even patient-specific clinical data mined directly from prior reports all represent clinical knowledge that is becoming instantaneously available simply by asking for it.
The universal PACS workstation
With the rapid penetration of digital mammography, cardiac CT, 4D ultrasound, PET/CT, and the emerging molecular imaging, some radiology departments have found it nearly impossible for all of these examinations to be read on a PACS diagnostic workstation. The need to purchase modality-optimized workstations has led some PACS-enabled radiology departments into an image management and workflow nightmare.
Imagine following the images generated by any combination of these modalities through your hospital's digital delivery systems to workstations dedicated to specific specialty or modality types. It is chaotic for radiologists, PACS and radiology department administrative staff, and the IT team. As mentioned above, specialty workstations also restrict image access to all the clinicians that need this access. Much like the 3D integration challenge, success will be achieved only by those systems that offer seamless viewing with powerful software of any modality, from any workstation, anywhere in the world. That was the original objective of PACS. That is the future objective of PACS.
The good and bad of radiology is that it will never stand still. What radiologists considered state-of-the-art yesterday provides limited functionality today, only to be unacceptably obsolete tomorrow. To maintain state-of-the-art, the radiology community of clinical professionals and vendors must continuously learn from each other and embrace the certainty that we can never stand still.
Dr. Mezrich is a Professor of Radiology and the Chairman of the Department of Radiology, University of Maryland School of Medicine, Baltimore, MD.
The impact of technology in medicine has grown enormously, mostly due to the development of computers. Only 20 years ago, when computed tomography (CT) came onto the horizon, if 1 slice was produced every 5 minutes, that was considered high technology. Several years later, CTs produced a couple of slices every few minutes. Now it is not uncommon to get a data set of 2000 slices in just 10 to 20 seconds. And it is getting worse by the moment.
Technologic innovation has put a tremendous burden on radiologists. In the "olden days," I would sit at my viewbox down in the hospital's basement, where it was nice and dark. I would get a film every now and then. I would put it up on my viewbox, mumble an opinion, and life was nice. As the number of images kept increasing, I had more and more films to deal with. Someone developed an 8-film alternator, and we adopted our protocols to a kind of Procrustean scheme in which my acquired images always fit on 8 pieces of film. As the number of images increased further, I put more images on each page of film. I was able to handle this until the number of images per examination got to be several hundred. Then things went to hell.
At this point, I started using workstations, and these were able to make work okay again. Workstations enabled me to thumb through studies more rapidly. Workstation software made image interpretation manageable for image sets of 300, 400, and 500 slices, but when the image sets reached a couple thousand in number, the workstations could not manage the data-and neither could the radiologists.
Three-dimensional (3D) workstations emerged as a new way of looking at studies. All of a sudden, it became apparent that the way radiologists used to look at images-one slice at a time-was no longer relevant. No longer was there a relationship between the way an image was acquired and how it was viewed. In fact, I could look at it any way I wanted to, not just in the axial plane in which it was acquired. Liberation of image viewing arrived with 3D technology. As a radiologist, I could use all the new technologies to do segmentation, to do 3D display, and to do cutting and random planes. All of a sudden, with the advent of 3D workstations, life got better again for the radiologist.
Or did it? What happened, in fact, is that my clinicians said that they needed the same viewing tools too. If my friend, who is a neurosurgeon, is trying to understand where a tumor is, he can't plow through 2000 images any more than I can. Picture archiving and communication systems (PACS) and 3D enabled the neurosurgeons and other clinicians to have access to the new techniques for viewing images-and to the images as well. Today, the clinicians with whom I work can look at a study any time that they want to-maybe even at the same time that I am rendering a professional opinion. This capability did not exist before the evolution of digital technology. The desire of clinicians to review, one by one, sets of images numbering in the hundreds to thousands did not exist either.
Current PACS are revolutionizing the world of diagnosis. In 10 short years, PACS has revolutionized the ability to instantly access a patient's files. The amazing evolution of PACS is not the result of strides made in diagnostic medicine; digital imaging and PACS have ridden the back of computer technology. For the purposes of the business world and for computer games, faster and more powerful computers are being developed continuously. Perhaps more importantly, bigger and faster archives to store all the data that computers generate are also being built. This is good for radiology, because if I have a 2000-image data set, it involves a couple GB of data. Several years ago, ≥ 2 GB was a big number. Now it is possible to carry several GB around on your key chain. The price of memory is dropping to the point at which it is possible to go to a consumer electronics store and buy 1000 GB-a couple of months' worth of studies-for roughly $1000.
Technology has changed enormously. How I distribute diagnostic imaging information has changed enormously. For as computers have evolved, the Internet has become pervasive. It is not a big deal for me to be remote from the room in which an examination is being performed. I can be down the hall, down the block, or on the other side of the country. If I render a professional opinion in a matter of minutes, who is to know or care where I am?
The implications of all this are confusing. What does instant imaging access mean to radiology? What is our profession's value added? Let's look at this in perspective. Our value added used to be that we owned the film. If someone wanted to look at a picture or discuss the case, he had to come to me. The clinician had to descend to my cubby in the basement, where I was the emperor, and this was nice. I also rendered an opinion, which I would like to think was accurate. Well, those days of being the emperor are gone.
Today, if a clinician wants to look at a digital image, he goes to his desktop PC and pulls up the picture from a Web server. So what is my value added? Today, it is the information I add to the study, which is what it always was. But now I need to provide information in a timely manner. If my opinion is not rendered in a timely manner-when patient care is being delivered-then I wouldn't be involved in the care of the patient. The PACS both forces and enables me to do my business. I am supposed to be making diagnoses that will help my clinical colleagues take care of patients. I have imaging equipment that produces images beyond my wildest expectations of even 20 years ago. All of this is a good thing-for radiologists, for hospitals, and for patients.
Now comes the question: Since I have these wonderful imaging technologies that generate really big data sets and really beautiful pictures, does a patient really need a physical examination performed by a doctor? I can see so much more about a patient than my clinical colleague can feel or smell. What is the purpose of a clinical exam? With these amazing new technologies available now, how should we medical professionals work-up a patient?
Many years ago, the entrance to the Steeplechase amusement park in Coney Island was a rotating drum through which one had to pass. The hospital of the future might learn from Steeplechase. The rotating drum at the entrance of medical care might be a CT scanner at the front door. Before even getting a work-up, a patient will get a scan. In fact, in many of today's very busy hospital emergency departments (EDs), this is already the mode. A nurse will perform triage, and the patient goes into the CT. How many times will an ED doctor say to the attending radiologist reading STAT exams, "That's Mrs. Jones. What do you think?" And the attending radiologist will say, "Tell me about the patient." To which the ED doctor will reply, "I haven't seen the patient yet. What do you think?" To the patients of the future, we may say, "Enter the CT. Let the fun and games begin."
This may be the current model in some hospitals today, and the model of the future in others. But what should the procedure be for working-up a patient? Is a scan from top to bottom the right thing to do? The laying on of hands and applying a stethoscope may in fact be as obsolete as X-ray film. But it forces us to talk with the patient and ask him or her what hurts. There must be a blend of humanity and technology. A clinician has an obligation to talk with a patient, to ask what hurts or what pickle the patient has gotten into. With today's technology, a clinician can find something wrong that needs to be fixed, but it might not be the right thing to fix. Just as most people have pimples on the outside of their body, they also may have pimples on the inside. Most of these are benign. But if you go hunting for them to fix them, you may well cause harm to the patient. So that is one sequela of the new technology. How should we be delivering care? By identifying potential problems or by eliminating them?
In my opinion, Sherlock Holmes is the model of the perfect diagnostician. In The Sign of Four, Chapter 6, Arthur Conan Doyle (a physician himself) has Sherlock Holmes say, "When you eliminate all other possibilities, what remains, no matter how improbable, is the answer." CT should be used to eliminate diagnoses, not define them. Of these items on the list of possibilities, why are you here, Mrs. Jones? What are all the things that could cause this problem? My goal should be to order only those tests that eliminate potential diagnoses-tests that reduce the list of all possible things that might have brought Mrs. Jones to my door… and whatever is left, no matter how improbable, is the diagnosis . The new technology is so much better and the speed so much quicker. By eliminating diagnoses, I may be able to identify the one that fits.
The prevalence of the new digital modalities should impact the way that medicine is taught to our residents. We should insist that they read Sherlock Holmes. We should teach them the process of eliminating diagnoses.
Does PACS have an impact on the functionality and even the design of a radiology department? In the days of film, I needed to be reasonably close to where the patient was, because I needed to read the films that were just taken. I may have needed to interact with the patient- and actually perform the examination. Today's exams require my presence far less. Do I even need to be at the hospital, especially if I do my job better than I did it 20 years ago?
By harnessing digital technology, we hope that as radiologists we are delivering better care by working less often. The fear is that will we become irrelevant. Why have a radiologist look at the picture? Why not have the surgeon or the gastrointestinal doctor look at it?
We radiologists do know more than other physicians about the interpretation of anatomy and the effect of disease on anatomy. That is our value added-understanding disease and how it appears on an image. To apply that information, we have to do this in a timely fashion. If we don't, we have no relevance.
The bottom line in this brave new world of digital technology and medicine is that it is incumbent upon the radiologist to provide service. But it was always our job to provide service. Now we've got to do it in a more timely fashion and in a way that guarantees that this information gets into the head of our clinical colleague when he or she needs this information. If we don't do that, then we will become irrelevant. What PACS and new fast CT scans force us to do and enable us to do is our job.
Dr. Strauss is Director, Radiology Physics and Engineering, Children's Hospital Boston, Harvard Medical School; Ms. Poznauskis is the Technical Director, Department of Radiology, Children's Hospital Boston, Boston, MA.
It is well understood and accepted within the radiology community that a computed radiography (CR) image receptor must be used as a 200-speed-equivalent system. To achieve this, approximately 1 mR of exposure at the plate is needed to have enough photons to obtain reasonable image quality. Some hospitals that use CR to perform pediatric imaging find this acceptable. Several years ago, during a tour of a hospital with a fully implemented picture archiving communication system (PACS), a pediatric technologist enthusiastically commented, "We love CR because you can use one radiographic technique for all pediatric exams."
At Children's Hospital Boston (CHB), we disagree. When the hospital implemented PACS in 2003, our radiology department's intent for CR was to find a way to obtain good image quality at a reduced dose for our pediatric patients relative to the 200-speed standard; and we succeeded. Today, we use 250-speed for extremity imaging, 350-speed for general imaging, and roughly 1100-speed for scoliosis imaging. These applications produce the same radiation exposure levels that had been used with our retired film-screen image receptors.
The process required to achieve these levels was not easy. It required additional time to set up the CR to produce clinically acceptable pediatric images for our radiologists, additional funding, and lots of teamwork on the part of our technologists, clinical medical physicists, radiologists, and our CR vendor's support staff. This article addresses issues that we encountered to achieve our objective: Consistently good CR image quality at radiation doses no higher than those used in film-screen radiography. This article provides some guidelines for radiology departments planning on implementing CR for pediatric imaging.
Unique challenges of pediatric imaging
There are unique challenges to pediatric imaging. A successful CR implementation must address these challenges and resolve the problems associated with them.
First of all, pediatric patients themselves are a challenge. Many are uncooperative and most exhibit typical childlike behavior. Children may have medical complications that make them difficult to image. They are accompanied by anxious parents who exhibit different levels of stress. Technologists dealing with pediatric patients and their parents need a CR system that is simple to use.
Another challenge is the wide range in girth of the patients that we need to accommodate from birth through adulthood. A neonate may have a posterior-anterior (PA) girth of approximately 10 cm, while a large adult can have a PA girth >30 cm (Figure 1). The length of the bar in the lower right corner represents a half value layer (HVL) at 70 kV equal to approximately 3 cm. How can this fact be used to help set up techniques? The HVL is the thickness of tissue that reduces the intensity of the X-rays passing through the patient by a factor of 2. For example, compare the girths of a neonate (9 cm) and a child (18 cm). One might assume that the radiographic technique for the child should be doubled because the child is twice as thick as the neonate; but this is not the case. The difference in girth is 9 cm, which is equivalent to 3 additional HVLs for the child. The number of X-rays required doubles for each HVL, resulting in a total increase in milli-ampere-seconds (mAs) by a factor of 8, provided the kVp of the X-ray generator does not change. In this example, the entrance radiation dose of the child is 8 times greater, not double, the dose of the neonate.
This example illustrates the point that a very large dynamic range of techniques is required to properly image patients from neonates to young adults. It is necessary to create either a thickness or an age-based technique chart that prescribes the appropriate kVp and mAs for each size of patient. At CHB, we facilitate the selection of the appropriate technique chart by programming the techniques into the controls of our X-ray generators (Figure 2). The programmed information includes the correct focal spot size and exposure mode, and options for both automatic exposure control (AEC) of the generator or a manual technique. Automatic exposure control techniques that work well for adult patients may be problematic for children. The reason for this is that the 3 individual cells of the AEC detector are properly positioned and sized for adults. The small size of the child's anatomy may not adequately cover or "shadow" these cells.
Why are we so concerned about radiation doses delivered to children from diagnostic X-ray exams? The increased radiosensitivity on the part of children compared with adults is illustrated in Figure 3. This illustrates that the attributable lifetime risk of a radiation-induced cancer from 1 Sv of radiation dose during the first decade of life in a child is approximately 15%, while it is only 1% to 2% for middle-aged adults. 1 This comparison results in the common generalization that children are 10 times more radiosensitive than adults.
To summarize, it is imperative that standardized radiographic technique charts be developed and used carefully. Our radiology department takes a "no-prisoner" attitude, and we make sure that everyone uses standardized radiographic techniques. This avoids the problem of exposure creep. Exposure creep was verified approximately 10 years ago by survey results of exposures used by technologists conducting chest radiographic exams at the University of California at Davis. 2 The explanation is very logical. A direct correlation exists between an increase in radiation dose and both a decrease in noise in an image and complaints by radiologists about image quality. Technologists quickly recognize this correlation and act accordingly. It is the responsibility of the quality assurance manager to monitor for exposure creep on a consistent, ongoing basis, as it is a reccurring phenomenon.
Computed radiography image receptors are more sensitive to the scatter radiation that is partially eliminated by grids than are conventional film screen image receptors. This makes the use of appropriate grids for adult imaging very important, even during portable radiography. However, small children do not generate as much scatter as large adults do. Having a grid that is designed to remove lots of scatter that may not be present from a child also removes many information-carrying X-rays, thereby requiring an unnecessary increase in patient dose. For that reason, we reduce the standard grid ratio in our table buckies from 12:1 to 8:1. We also put a set of rails on our upright buckies (Figure 4) and remove the reciprocating grids. We use stationary grids that can be easily slid in and out by the technologist. This feature is important because upright chest examinations for any patient under the age of 12 years are done without a grid. A high line rate (≥150 lines/in) is used to prevent moiré pattern artifacts with the digital image receptor that could otherwise occur with more common lower grid line rates.
Positioning aids are important in pediatric imaging to place the patient's anatomy at the center of a CR plate. However, positioning aids that are imaged alongside a child's anatomy can alter the effectiveness of a CR reader in automatically determining the correct latitude and sensitivity as signals from the image plate are read and digitized. The baby chest stand developed at CHB (Figure 5) eliminates the need for restraining devices that place additional attenuators in the path of the X-ray beam and may unnecessarily frighten the child.
Figure 6 illustrates the important placement of a gonadal shield in an image of a child's pelvis to reduce radiation dose to the child's gonads. The presence of this shield in the image will probably require the development of unique display parameters by the CR vendor due to changes in the graphical analysis resulting from the presence of a lead attenuator in the middle of the patient's anatomy.
Perceived noise in CR images of pediatric chests must be limited, minimizing the amount of pediatric patient dose reduction that can be achieved. Perceived noise in the image has the same appearance as hyaline membrane disease, a common lung disease in newborns. High levels of perceived noise may also camoufiage subtle bone details and minute fractures of children who have been abused.
Pediatric institutions typically perform a large number of scoliosis examinations; CHB performs >150 weekly. It is very important that the CR system selected have an efficient stitching program that allows for the production of an approximately 36-inch long image of the anatomy. Pediatric departments will suffer an unacceptable workfiow hit if the stitching software is not easy to use and efficient.
Important preliminary steps for CR implementation
If a CR system is going to be pushed to its limit to achieve a lower dose, variable performance among the multiple X-ray machines, the multiple CR readers, and the multiple display workstations must be eliminated. First, the beam quality, the kVp, and the mA accuracy of each X-ray machine must be tested and adjusted to create the same exposure per mAs at a standard distance from the focal spot. In addition, all the attenuators that intercept the X-ray beam between the focal spot and the image receptor must be standardized on each machine. This includes the attenuation of the collimator mirror, the added filtration, the tabletop, the grid, the AEC pickup, and the image receptor cover. To get the same exposure to the plate from room to room, it is necessary to deal with all of these attenuating factors and also to verify that each machine is producing the same amount of radiation for a standard radiographic technique. Once this is achieved, a single technique chart can be developed carefully and programmed into each generator control in each X-ray room.
Second, uniformity among CR readers is just as critical. Do not assume that this will occur automatically if the CR readers are from one manufacturer. When the 15 CR readers purchased by CHB-all the same model and manufacturer-rolled out of the box, each one had a somewhat different response to image plates with the same level of exposure. It is necessary to perform acceptance testing and calibrate the readers so that they are indeed identical.
Third, monitors on diagnostic display workstations must also be tested and adjusted to ensure the same maximum brightness and the same look-up table (LUT). Both of these standardizations to monitor performance are necessary to ensure that the same digital image displayed on multiple workstations will have the same appearance to the interpreting radiologist.
Pediatric image processing challenges
These steps that are necessary to optimize the clinical information stored and captured on the CR plate are analogous to the careful steps required by a photographer with a 35-mm film camera when capturing light on the film in the camera. In the case of the photograph, the film must also be properly developed in the processing laboratory under carefully controlled conditions to produce the final analog image. In the case of the CR plate, its stored energy must be properly read, digitized, and processed to produce an electronic digital image that can either be displayed on the soft-copy monitors of a display workstation or printed on film for interpretation by the radiologist. Pediatric imaging places some unique demands on the CR reader-the processing lab in our analogy.
Most adult chests fill up the entire CR plate, but most pediatric chests do not. As a result, the CR reader must be capable of identifying the collimated border of the X-ray field hitting the plate. All current CR systems can do this quite well.
Once the collimated area is found, the CR reader performs an initial analysis of the data within the collimated border. This analysis tells the reader how much to amplify the digital signal that will represent each pixel in the final digitized image. Figure 7 presents the graphical analysis of both a child and an adult chest. The shapes and amplitudes of the two curves are different because the X-ray patterns in space that exit the child and the adult thoraxes are different. These differences are created as the X-rays pass through the unique anatomic structures in patients of different ages. The dashed straight line for each patient determines the amplification and latitude of the digitized image. Since the dashed straight lines for the child and adult chests are different, the final display parameters that determine the final appearance of the digitized clinical image must be different for adults and children. This means that age-specific display parameters within the CR reader must be developed for each anatomic region of the patient's body. While all manufacturers of CR readers have carefully defined and tested display parameters for all anatomic regions of the adult patient, typically the manufacturers' display parameters for children are not as complete as they should be and are not tested as well.
Contrast in the final image is controlled by these display parameters. Figure 8 shows 3 images of the same child. The unprocessed image results from the graphical analysis illustrated in Figure 7 that is applied to the raw image. The "contrast-enhanced" image is a digitized image with contrast levels similar to those expected with an analog film-screen image receptor. The CR reader calculates this contrast-enhanced image by applying curves from a LUT that are similar in shape to the H and D curve of the film-screen image receptor. Computed radiography manufacturers provide display parameters that allow the shape and slope of the curves in the LUT to be adjusted for each specific anatomic region of the body as a function of patient size.
Because anatomic structures in newborns and small children are much smaller than in adults, these smaller structures require smaller pixels in the final digital image (improved high contrast resolution) to properly image the anatomic detail. While most CR systems use 5 pixels/mm to produce the final clinical image, we doubled the pixel density to 10 pixels/mm in the final image to adequately image these small pediatric structures.
The majority of final digital images created by CR readers are also edge-enhanced (Figure 9). While the degree of enhancement increases the conspicuity of edges in the image, it also increases the amount of perceived noise in the digital image. As previously mentioned, significant increases of perceived noise in pediatric images cannot be tolerated due to the need to detect hyaline membrane disease and other pediatric disease states that are masked by the presence of noise. Our particular CR reader provided an image-processing routine using multiple frequencies that allowed reasonable enhancement of edges without unacceptable increase in perceived noise. This processing capability is invaluable in providing images acceptable to our pediatric radiologists while also reducing patient radiation doses.
So how did our department develop image processing parameters that were patient-size-specific to create quality images from a lower radiation dose without collectively losing our minds? We obtained extensive feedback from our pediatric radiologists on their clinical requirements for our pediatric images. We developed a series of images with different levels of contrast using 10 pixels/mm that were thoroughly reviewed with our pediatric radiologists. Once the radiologists selected their preferred contrast scale, we created another sequence of images using that contrast scale with different amounts of edge enhancement, exploiting multifrequency processing. These images were discussed to identify the delicate balance between sharpness in the image and the level of perceived noise. Once the desired contrast, edge enhancement, and sharpness are obtained in the clinical images through display parameter adjustment, the final step involves adjusting the brightness of the image on the display monitor.
The additional cost of implementing CR imaging for pediatric patients
Quality costs. Clinical physics testing and X-ray equipment vendor support are needed to standardize the output and attenuation of all X-ray-producing machines. Unless this equipment is in substandard condition, this should be possible with ≤10 hours per machine divided between your physicist and X-ray machine vendor. Once uniformity between machines is obtained, the technologist's job of developing radiographic technique charts is simplified because only one chart for all rooms is necessary. This information can be loaded in each X-ray machine in a few hours; less time is needed if all X-ray machines are of similar ages and from the same vendor.
Acceptance testing of each CR reader and subsequent calibration requires 5 to 7 hours, split between the physicist and the CR reader vendor. A pair of monitors on a diagnostic display workstation can be acceptance tested and calibrated in 1 to 2 hours by a competent service technician or quality control technician. The optimization of display parameters as a function of patient size for each anatomical area of the body may take 3 to 4 weeks of work by a dedicated technologist who understands the display parameters of the CR system and who has the ready support of the CR vendor's support specialists.
The decision of CHB to obtain high contrast resolution using 10 pixels/mm to achieve better visualization of the small structures in the image also has a cost. What this means in practical PACS terminology is that each 43- × 35-cm CR uncompressed image has a file size of 32 MB. With JPEG lossless compression, each image averages 13 MB. Still, 10 versus 5 pixels/mm, the standard used by most hospitals, quadruples the size of images that must be transported across the institution's network requiring 100 Mbps network transmission outside the radiology department and, preferably, 1 Gbps network transmission within radiology. Therefore, storage requirements are effectively quadrupled. This not only means a larger capital acquisition cost for online storage and archival devices but also a proportionally larger expenditure each time storage is expanded. These high-pixel-density images also demand the best display monitors (currently 5 megapixel units with the highest prices on the market) on diagnostic workstations.
Conclusion
The process of achieving ALARA (as low as reasonably achievable) radiation dose in pediatric CR is a major undertaking. We at CHB believe it was worth the expense. Conferences on this subject, such as the one sponsored in February 2004 by the Society for Pediatric Radiology, do much to educate and encourage pediatric radiologists, medical physicists, radiolologic technologists, and imaging scientists and engineers to collaborate with each other to make this process easier and less resource-intensive. 3
REFERENCES
- Hall EJ. Lessons we have learned from our children: Cancer risks from diagnostic radiology. Pediatr Radiol.2002;32:700-706.
- Seibert JA, Shelton DK, Moore EH. Computed radiography X-ray exposure trends. Acad Radiol. 1996;3:331-338.
- Willis CE, Slovis TL. The ALARA concept in pediatric CR and DR: Dose reduction in pediatric radiographic exams-A white paper conference executive summary. Pediatr Radiol. 2004;34(suppl 3):S162-S164.
ADDITIONAL RECOMMENDED READINGS
- Huda W. Assessment of the problem: Pediatric doses in screen-film and digital radiography. Pediatr Radiol.2004;34(suppl 3):S173-S182.
- Hufton AP, Doyle SM, Carty HM. Digital radiography in paediatrics: Radiation dose considerations and magnitude of possible dose reduction. Br J Radiol. 1998;71:186-199.
- Seibert JA. Tradeoffs between image quality and dose. Pediatr Radiol.2004;34(suppl 3):S183-S195.
- Steven D. Radiosensitivity of children: Potential for overexposure in CR and DR and magnitude of doses in ordinary radiographic examinations. Pediatr Radiol.2004;34(suppl 3):S165-S172.
- Willis CE. Strategies for dose reduction in ordinary radiographic examinations using CR and DR. Pediatr Radiol.2004;34(suppl 3):S196-S200.
Dr. Dreyer is Vice Chairman of Radiology and Corporate Director of Enterprise Imaging, Harvard Medical School, Massachusetts General Hospital, Boston, MA. Dr. Berman is a Director of iAtrogenix, Inc., Tucson, AZ.
With experience in the academic, private-practice, and vendor communities, we have the pleasure of visiting numerous hospitals and their radiology departments throughout the world. We have evaluated myriad radiology information systems (RIS) and picture archiving and communication systems (PACS) to explore their effectiveness and frustration points. It has become apparent to us that PACS and RIS implementations in hospitals today vary widely in their features and functionality.
This variability is due to a host of factors, many of which center around capital budgets and hospital finances, the ages of legacy RIS and PACS, the degree of PACS and healthcare information systems deployment, network infrastructure robustness, level of information technology (IT) integration and support, data-storage design and management, and the proclivity for technology adoption culture within a healthcare institution or enterprise.
In a highly competitive healthcare service providers market, the impact of PACS and RIS to improve the management and access of patient information and to increase productivity, efficiency, and job satisfaction levels among clinicians and support staff can place a radiology department and/or imaging center at a tremendous advantage or disadvantage to its competitors. This is being documented anecdotally in radiology and informatics publications.
Healthcare facilities are wise to adopt the attitude that change is good. Both RIS and PACS should be kept current with the purchase of software upgrades. With the maturation of Digital Imaging and Communications in Medicine (DICOM) and Health Level 7 (HL-7) standards, innovative products from third-party vendors can be integrated more easily and economically.
PACS technology is in a continuous stage of evolution. We consider the following features to be state-of-the-art. If your department has not yet implemented PACS or RIS, you might use this list to differentiate offerings by various vendors. If you are already a PACS or RIS user, we recommend that you use this list to evaluate your current vendor's offering and to see where you stand compared with the leading-edge technologies that are materializing in 2005.
Advanced visualization
Considered novel several years ago, 3-dimensional (3D) and volume visualization has become mainstream. Its adoption is out of necessity. Massive amounts of data are streaming from multidetector computed tomography (MDCT), cardiac CT, positron emission tomography (PET)/ CT, high-Tesla magnetic resonance imaging (MRI), and 4-dimensional (4D) ultrasound modalities. However, the use of independent 3D processing systems external to a PACS is fraught with problems. The workflow efficiency of radiologists is hindered by the necessity of moving between PACS and dedicated 3D workstations. Proprietary hardware and expensive software prevent its wide deployment, even within the radiology department itself. Even nearly state-of-the-art thin-client solutions allowing for generic PC access to a centralized advanced visualization system require the addition of yet another redundant array of inexpensive disks (RAID) system in the ever-expanding medical image storage explosion.
There was a widespread introduction of advanced visualization software integrated into PACS workstations in 2004. The capabilities vary greatly. With some, the interpretation process can be slow. In addition, many of the basic PACS features are not available during the use of 3D analysis. Try, for example, to use hanging protocols to compare a "with and without" contrast multiplanar reconstruction series to a similar prior examination.
State-of-the-art institutions have optimized their use of 3D to such an extent that it represents a significant part of their daily volume. Such advanced visualization exists today as an integral part of some PACS vendors' offerings. Users of these systems have seamless access to complex 3D visualization techniques from any workstation. But beware, there is a significant increase in radiologist productivity when using 3D functionality designed into a PACS versus a 3D system that has simply been integrated with a PACS. A good test for seamless integration is to confirm that PACS 3D functionality is as widely available, and as easy to use, as window/level or zoom.
Remote interpretation
Whether it occurs in the form of nighthawk services, home teleradiology, or covering a department while visiting another part of the world, remote reading has become a radiological necessity. In our experience, the ability of an institution to perform remote interpretation is becoming essential for recruitment and retention of radiologists.
Efficient remote interpretation is completely dependent upon the PACS architecture of an institution or an enterprise. Web-based technologies, computer hardware and storage advancements, and global proliferation of high-speed network accessibility have revolutionized diagnostic image distribution. State-of-the-art systems exist in which remote interpretation is identical to that of interpretation in the reading room. Prior images, reports, patient clinical information, and 3D are as easy to access from a hotel room in Hawaii as they are from a reading room in the hospital.
Such state-of-the-art systems can now be easily integrated with a PACS and a RIS. A user is no longer limited to deploying his/her PACS vendor's Web-viewing product. In today's world, a hospital with remote image distribution functionality that is limited to clinical review functionality is obsolescence-challenged.
Remote order entry and decision support
Today we can schedule trips and order plane tickets on the Web, finding the best prices and shortest times, all without talking to a single person. So why is it that we continue to burden our referring physicians with inefficient, manually intensive examination ordering processes? While most RIS require ordering physicians to call or fax schedulers to negotiate through the ordering process, alternative systems are available that make this process as easy as booking a flight online.
State-of-the-art radiology examinations ordering and scheduling is done over the Web. Smart RIS have the flexibility of offering the soonest available times in one or multiple locations, of scheduling multiple examinations for the same day, and of advising of the order in which multiple examinations should be scheduled. They activate prequalification processes within the RIS and provide electronic forms for completion. Just as speech recognition has optimized the reporting process, remote order entry (ROE) has streamlined another laborious and error-prone process.
With the advent of Web ordering comes the opportunity for additional onboard clinical knowledge delivery. New radiology decision support (RDS) is providing clinicians with up-to-date clinical knowledge regarding appropriate examination usage. Many clinicians today cannot keep abreast of state-of-the-art diagnostic procedures that may be more effective than a familiar alternative. Or conversely, they may be so enamored with or feel so protected by utilizing the newest solutions that these high-cost procedures are ordered inappropriately. Radiology decision support systems based on the American College of Radiology's (ACR) appropriateness criteria are rapidly guiding clinicians through the morass of indications and imaging alternatives, directing them toward the most appropriate examination given their patient's clinical state.
When a clinician orders a study, a selection of clinical indications is presented. As soon as indications are selected, an appropriateness criterion rating is made, as well as recommendations for substitute or supplemental procedures. Radiology decision support systems not only educate clinicians, but they can also reduce the number of inappropriate studies performed and may be used to justify recommended procedures to medical insurance companies for rapid prequalification and approval.
Advanced reporting and artificial intelligence
We all do it. We scour through hundreds of images looking for intricate anatomy and detailed pathology in a variety of planes and reconstructions to render a diagnosis. Then, due to the limitations of many systems, most of us transcribe our visual findings into a narrative report. Wouldn't it be great if we could record and share this effort by easily capturing concise visual cues to present our findings to our ordering physicians? Well, a limited few are actually using state-of-the-art systems to do exactly that.
Tight integration of PACS and reporting systems have allowed for the seamless integration of text and images. While most of us still use human transcription to convert spoken words into computer text, others have successfully mastered speech recognition (SR), drastically decreasing the production costs and delivery time of critical diagnostic results. Recent advances in speech recognition and natural language understanding (NLU) are bringing even greater accuracy to the reporting process.
State-of-the-art systems are providing instantaneous structure to SR-generated reports by employing on-the-fly NLU. The result is a reporting application that understands what the radiologist is saying while he or she is dictating. The power that this can bring to the interpretation process is incredible.
One such example is the ability to automatically reference medical information during the interpretation process and add it directly to the resulting report. Because state-of-the-art reporting systems can now recognize what a radiologist is saying, they can respond automatically with critical information that might otherwise be unavailable to the interpreting radiologist. Such information might include differential diagnosis, disease details, or recommended follow-up processes. Similarly, if the name of an anatomical part evades memory, a radiologist can click directly on a displayed reference image and be enlightened instantly. Differential diagnosis, detailed pictorial atlases, teaching file cases, or even patient-specific clinical data mined directly from prior reports all represent clinical knowledge that is becoming instantaneously available simply by asking for it.
The universal PACS workstation
With the rapid penetration of digital mammography, cardiac CT, 4D ultrasound, PET/CT, and the emerging molecular imaging, some radiology departments have found it nearly impossible for all of these examinations to be read on a PACS diagnostic workstation. The need to purchase modality-optimized workstations has led some PACS-enabled radiology departments into an image management and workflow nightmare.
Imagine following the images generated by any combination of these modalities through your hospital's digital delivery systems to workstations dedicated to specific specialty or modality types. It is chaotic for radiologists, PACS and radiology department administrative staff, and the IT team. As mentioned above, specialty workstations also restrict image access to all the clinicians that need this access. Much like the 3D integration challenge, success will be achieved only by those systems that offer seamless viewing with powerful software of any modality, from any workstation, anywhere in the world. That was the original objective of PACS. That is the future objective of PACS.
The good and bad of radiology is that it will never stand still. What radiologists considered state-of-the-art yesterday provides limited functionality today, only to be unacceptably obsolete tomorrow. To maintain state-of-the-art, the radiology community of clinical professionals and vendors must continuously learn from each other and embrace the certainty that we can never stand still.
Dr. Matalon is Chairman, Department of Radiology, Albert Einstein Medical Center, Philadelphia, PA.
When picture archiving and communication systems (PACS) were first commercially deployed, the technology was not radiologist-friendly. PACS workstations were designed by engineers whose knowledge of radiologists' reading procedures and daily workflow was negligible at best. Over the ensuing years, radiologists' and technologists' input to the design process has yielded improvements. These improvements and the near-instant access to prior examinations have contributed to increasing productivity in the reading process. One area in which research is needed and improvements need to be made is in the creation of reading protocols designed to present images and associated information most efficiently and intelligently optimized for accurate and rapid diagnosis.
The hierarchy of hanging protocols is typically linked to specific user, modality, examination, or body-part-specific directions to the PACS to consistently "hang" images in a repeatable orientation on the display monitor. Most PACS allow various steps aimed at displaying images based on body part, modality, and series descriptor. While hanging protocols simply recapitulate the hard-copy environment of hanging films, reading protocols take advantage of the digital nature of images. Reading protocols can embrace a pure "digital" environment, where the capabilities of the computer system can be harnessed to automate the diagnostic process. They allow for manipulation of the images and for the display of information, such as scanned documents; in doing so, they theoretically enhance productivity as well as quality of interpretation.
Hanging protocol creation has historically been treated as a function of customization for a radiology department and/or for individual radiologists to perform. From the outset of PACS, vendors recognized the need to prefetch relevant examinations from storage archives to make them instantly accessible for comparative review. But the general wisdom, which unfortunately still predominates today, is that every institution (and/or radiologist) has its own protocol preferences and that these must be customized, just as each PACS design architecture is customized to the institution.
The increasing complexity of displaying computed tomography (CT) and magnetic resonance (MR) imaging examinations has led to an ongoing collaboration and codevelopment between radiologists and vendors of a steadily expanding library of sophisticated hanging protocol formats. This has not been an easy task, as modality vendors have had different descriptors for similar or the same acquisitions. The recent ability for examination and series descriptors to be renamed has led to more uniformity and ease of implementation. In fact, the complexity and diversity of series nomenclatures and the lack of a common agreed-upon standard for series naming have forced the PACS vendors to develop techniques to try and create standardized display protocols. It has also had an unfortunate impact on system customization by forcing users to customize the systems based upon the nomenclature in use and the mix of modalities. Clearly there is an opportunity for the radiology community to continue to define standardized series descriptors and names, and to rationalize the use of series types.
The modification of hanging protocols into reading protocols has nonetheless been the responsibility of the radiologist. Reading protocol creation is a time-consuming task. Increasingly, PACS vendors incorporate an easy wizard associated with the creation of a specific hanging protocol. But many still do not offer this idiot-proof ability. As a result, the burden of creating reading protocols is typically performed (under the direction of the radiologist) by the vendor's clinical applications training specialist. This usually occurs at the time of implementation, when the new-to-PACS radiologist does not have the experience to articulate what he/she really needs. The predominant vendor mentality, that the development of reading protocols is so individual that it should be left to the individual radiologist, has forced radiologists to start from ground zero with respect to understanding and analyzing the subtleties of hanging protocol display and image manipulation capabilities. This is unfortunate.
There is an opportunity through standardization in the area of series nomenclatures and vendor adaptation to start to deliver systems that, right out-of-the-box, employ a "best practices" reading protocol that can be refined easily based on use. Perhaps in the future, users will have the capability to share protocols more easily with other sites as a means to improve the practice of radiology. Today this is limited within the venue of PACS-vendor-specific user groups or e-mail discussion groups.
Reading protocols add intelligence to hanging protocols, because they assemble and display current and prior examinations in a consistent manner designed to best facilitate review and diagnosis. The large data sets produced by CT and MR, combined with integration of 3-dimensional (3D) visualization, have greatly stimulated research in this field.
However, computed radiography (CR) reading protocol development has largely been ignored. This may be attributed to several factors:
- PACS vendors presume that CR vendors have developed image processing algorithms to optimize image quality in terms of sharpness, contrast, spatial resolution, and dynamic range. This presumption is true. Such efforts are ongoing. 1
- Conventional radiography lacks pizzazz and sex appeal. You cannot stack, ruffle, and create 3D visualization from conventional radiographs. Conventional radiography lacks a fear factor. A radiologist is not confronted in the course of a day with the need to interpret procedure after procedure that have generated hundreds to several thousand images.
- Creating reading protocols for CR by type of examination, disease, or clinical indication is a daunting experience, even if good protocol-creation tools are available. CR generates images of every possible body part: the hand, each and every finger, toes, feet, ankle, knees, thighs, tibula and fibula, kidney/ureter/ bladder studies, and on and on. The sheer number of potential reading protocols to be optimized is huge. Since most systems display the current examination on the left and the most recent similar examination on the right, and the quality of the images is good, most radiologists just live with that. They take the time to manually perform additional image manipulation and do not think about the time they are wasting that could be automated for their workflow benefit. Nor has academic research focused on workflow analysis measuring this lack of efficiency from the failure to truly fine-tune the viewing process.
CR reading protocols are workstation-dependent. They will differ between a dual- and a single-monitor workstation with respect to display and arrangement of current and prior images or those with postprocessing filters applied (Figure 1).
One example of a CR reading protocol is that used for a patient who has a recently placed central venous catheter. In the clinical setting, a film of the chest is generally obtained with 2 questions posed to the radiologist: 1) What is the course and location of the tip of the catheter?; and 2) Is the catheter placement associated with a complication? What is usually of concern is a pneumothorax or a partially collapsed lung that might be secondary to a needle placement in the chest.
The conspicuity of a catheter that might be relatively difficult to see in the chest can be increased by applying an edge-enhancement filter to that image (Figure 2). The radiologist may also be able to better detect a very fine line associated with a pneumothorax or a partially collapsed lung (Figure 3). This is one example in which a reading protocol takes advantage of understanding the pathology associated with a particular disease process. Edge-enhancement filters are also frequently used to accentuate a questionable interstitial opacity and make it more apparent.
Similarly, bony structures are better seen using a reverse black-on-white instead of a black-on-white projection. At our hospital, a popular reading protocol is to take bony images, particularly those of the spine and pelvis, and look at those images in both modes side by side. The reverse image frequently depicts the bony and osteo structures clearly.
Because conventional radiography represents up to 70% of the total number of procedures performed at a hospital, the addition of specific CR reading protocols configured for easy selection would represent a true enhancement to diagnostic workstation functionality. Ideally, research will be done that will show that certain kinds of conditions and examinations are optimally viewed in a particular way. The PACS will be able to specify by CPT code alone or by looking at the ICD-9 to apply particular reading protocols that are driven by one of those two factors in combination. More experiments need to be performed, such as the one conducted in 1999 using morphological filters and active contour models to improve automatic detection of foreign objects in CR images. 2 PACS users-group meetings and Internet discussions provide a forum whereby CR reading protocols can be shared and ideas refined. Vendors can be encouraged to take another big step to make the workday easier.
REFERENCES
- Expert interview: Workflow optimization: Where soft-copy reading technology is headed. Fuji Insights Images. 2003;Summer/Fall:2-3. Available online at: www.fujimed.com
- Xuan J, Adali T, Wang Y, Siegel E. Automatic detection of foreign objects in computed radiography. J Biomed Opt.2000;5:425-431.
Dr. Dreyer is Vice Chairman of Radiology and Corporate Director of Enterprise Imaging, Harvard Medical School, Massachusetts General Hospital, Boston, MA.
Use of Internet technology as a picture archiving and communication system (PACS) strategy has changed dramatically during the past 5 years. Just a decade ago, when the World Wide Web was in its infancy, deployment of a PACS was limited to the confines of a hospital using a hard-wired local-area network (LAN). Radiology information systems (RIS) and PACS were very separate entities, although both were twin-like workflow systems designed for use in a radiology department.
The standard communications protocol for RIS is Health Level 7 (HL-7), while the standard for PACS is Digital Imaging and Communications in Medicine (DICOM). For many years, the only way to make these two standards communicate with each other was through a computer gateway. Since this solution was far from ideal, the Radiological Society of North America (RSNA) and Healthcare Information and Management Systems Society (HIMSS) joined together to create the Integrating the Healthcare Enterprise (IHE) initiative. This initiative was designed to provide a venue for the vendor, information technology (IT) development, and radiology IT research communities to enhance communication exchange, and to approve protocols to replace the gateway straddling the middle of the HL-7/DICOM interchange with more standards-based architectures. The IHE protocols continue to be approved. Their scope is expanding into other "-ologies," and the momentum for inclusion in commercially available products is accelerating.
The integrated digital radiology department of 2005 consists of a RIS, a PACS, a speech recognition system, and an interface through the RIS to the hospital electronic medical record (EMR) (Figure 1). Most of these are interfaced rather than fully integrated. This is, after all, 2005.
Missing from this illustration are clinicians making orders electronically from an EMR patient dashboard and results from the radiology department pumping directly into the EMR. In fact, many hospitals are still pushing text to clinicians rather than providing the clinicians with direct access to a computer information system. There is no need for a clinician to push an image into the PACS, but there are very important reasons to push the images out. Radiologists, after all, are not the only consumers of images. The ability to move images wherever they might be needed hits the well-known roadblocks of availability and affordability.
Traditional PACS communicated through rigid LANs and wide-area networks (WANs), and required dedicated workstations. This triggered the development of differentiated diagnostic, clinical, and review workstations common in PACS of the 1990s. At the time, the cost of making PACS accessible outside a radiology department was an extraordinarily expensive proposition. The outside world was typically linked through a dedicated teleradiology server.
DICOM is an excellent communication protocol to transfer large amounts of data from machine to machine. It manages verification, and remembers the order of what needs to be done, what has been completed, and what has not been successfully transferred. The DICOM standard does the "heavy lifting." It is excellent for machine-to-machine computer information transfer.
The Web is excellent for user connections. It uses standard hardware and standard networks. It offers a cost-effective software-only solution, which can be managed, upgraded, and serviced from a single central location. The Web scales well. The reason Web browsers run on everything is that they are used for everything. Web browser technology is free and ubiquitous. And from the perspective of maintaining the integrity and confidentiality of patient records, Web technology uses secure communication protocols and protection developed for online financial transactions.
Marrying Web technology to PACS has expanded the boundaries of DICOM communications. Web servers that sit on top of a traditionally designed PACS give it a shiny front end. It becomes affordable and feasible to empower any computer within and outside a hospital to access PACS information. Web technology running over the Internet can provide cost-effective, secure access to medical images, provided that technology such as virtual private networks (VPN) or secure socket layers (SSL) is employed. A Web server becomes an intrinsic part of the PACS, the source of patient images and reports, and the gatekeeper through which requests for archived information can be made and retrieved. This is the trend today, and it works quite well (Figure 2A). But there is a more ideal option available-the option to completely restructure the PACS and have an entirely Web-based system (Figure 2B).
A Web-based PACS is designed to run on top of the Internet. Its architecture is scalable, offering the ability to connect multiple facilities within a healthcare enterprise (Figure 3).
The Web-based PACS provides an easier connection to the EMR. The EMR, in concept, provides the portal by which a clinician can access information about his/her patient from the many clinical systems containing patient data-laboratory information systems, pathology information systems, drug management systems, cardiology information systems, etc. Recognizing the power of the Web, designers of EMRs have also created Web-based systems for easy clinician access.
Today's Web-based PACS provide capabilities that either cannot be achieved or are achieved with greater difficulty and expense by PACS using traditional architectures. Web-based PACS typically assign a unique universal resource locator (URL) to each piece of patient data. This makes it easier and less costly for an EMR to enable a clinician to access patient data through a Web portal or patient dashboard. Implementation and management of a common patient database representing multiple RIS and PACS is more feasible using Web-based technology. Updating software in diagnostic workstations, as well as in ordinary computers, is performed from a central location. Web-based document scanning and auto-filing systems facilitate the input of documents generated from diverse locations into a PACS, regardless of boundaries.
Virtual Radiologic Consultants (VRC) of Eden Prairie, Minnesota, is an excellent example of a radiology practice that spans the globe, and its facilities are connected through a Web-based RIS and a Web-based PACS. As Dr. Sean Casey, 1 founder and CEO, advised in the DXP conference's keynote presentation, VRC provides nighthawk teleradiology services to more than 350 client hospitals. Its 35 radiologists are located in North America, Europe, Australia, and Asia.
Exceptional IT is mandatory at VRC. They use a proprietary self-developed Web-based RIS called AutoRAD that distributes work evenly among the staff while directing studies to radiologists who possess the appropriate credentials to perform the interpretations. VRC's routers and servers are located in the same room as its telecommunications company's Sonet ring. The proximity to this high-speed Internet backbone loop speeds up VRC's Internet connection considerably. The ability to transfer images securely over the Internet in a cost-effective manner serves as the underlying economic engine for this nighthawk practice. 2
Medical imaging in the 21st century is Web-based, and its totally integrated functionality will blur the separate disciplines of the "ologies" that exist today. This, in fact, is happening now; traditional PACS vendors are now offering radiology and cardiology PACS software on the same workstation. It is safe to surmise that over the years, the term "PACS" will become as antiquated as the term "teleradiology." Today, teleradiology is merely an extension of an enterprise-wide PACS (Figure 4). In the future, PACS will be an element of an entirely Web-based, chronologically comprehensive, and all-inclusive patient information system. A PACS without a Web-based architecture will seem as archaic as the pioneering camera-on-a-stick telephone line teleradiology of the 1980s seems today.
REFERENCES
- Casey S. The impact of the Web on radiologist shortages. Presented as the Keynote speach at the 9th Annual Digital X-Ray and PACS: An Educational Forum. Scottsdale, AZ, February 26 - March 2, 2005.
- Brice J. Continued ITevolution boosts teleradiology. Diagn Imaging. 2004;Feb Suppl.
Dr. Avrin is Professor of Radiology and Adjunct Professor of Medical Informatics at University of Utah and Chief-Body Imaging, Department of Radiology, University of Utah Hospitals & Clinics, Salt Lake City, UT. Dr. Tellis is a Programmer/Analyst at the Laboratory for Radiological Informatics, University of California San Francisco, San Francisco, CA.
Lawrence Roberts and Tim Berners-Lee are not familiar household names to most of the world's population, yet our high-technology existence is indebted to them. Lawrence Roberts led the team that launched the Internet in 1969. 1,2 Twenty years later, Tim Berners-Lee invented the World Wide Web, 3 which he envisioned would be an Internet-based hypermedia initiative for global information sharing. These inventions have changed the way our 21st century civilization communicates, and during the past 5 years, they have also significantly altered the nature of radiology workflow.
The modern version of the Internet first appeared in the early 1980s and is based upon the transmission control protocol/Internet protocol (TCP/IP) . TCP/IP introduced the concept of the numeric IP address for uniquely identifying computers connected to the Internet. Another major breakthrough occurred in the mid-1980s with the advent of domain name server (DNS) system, which enabled computer-centric IP addresses to be mapped to human readable names (ie, www.rsna.org > 192.203.125.59).
In 1989, Tim Berners-Lee invented the World Wide Web with its underlying protocol: the hypertext transfer protocol (HTTP) . HTTP relies on uniform resource locators (URL) to connect a client application (such as a Web browser) to content hosted on a server. In 1991, exactly one Web server existed in the world.
A key function of the Internet is that it enables the interoperability of disparate systems. The standards (TCP/IP, DNS, HTTP) that compose the Internet allow computers (regardless of their physical location) to communicate using a standard software paradigm. Within the medical arena, this has meant that once-isolated clinical information systems can be integrated to allow for the rapid flow of data. For example, a speech recognition system can use the network to communicate with a radiology information system (RIS) to verify that both have opened files for the same patient.
In recognition of the importance of interoperability, Health Level 7 (HL-7) introduced the clinical content object workgroup (CCOW) standard in 2000. This standard leverages both Web standards and Microsoft Windows-specific application program interfaces (APIs) to achieve clinical desktop integration, where independent clinical applications can be made to work in concert. For example, CCOW enables single sign-on functionality. A user needs only to log in once to access multiple applications. The CCOW standard also provides content synchronization for the automatic display of the same patient's data within multiple applications.
This is merely a brief introduction to technology that has made Web-based workflow possible. In a radiology application, as long as a client PACS workstation has a Web browser, and appropriate services are set up on a Web server, it is possible to create custom workflows.
The value of integrating a PACS workstation with a Web application was demonstrated at the University of California San Francisco (UCSF) Hospital. 4 In 2001, the UCSF Department of Radiology was in the midst of a phased transition to an all-digital environment. While working at a PACS workstation, radiologists needed a way to determine which prior X-rays of a patient were available electronically and which were available only on film. One of the main workflow issues in the radiologists' efficiency in a conversion to PACS resulted from the fact that the PACS and RIS each maintained independent databases. The RIS recorded all studies performed, but the PACS was aware of only those stored in its digital archive. The UCSF radiologists did not want to have to log onto both the RIS and PACS to make time-consuming and tedious comparisons of a patient's records on each system. When using only the PACS, there was a disquieting uncertainty regarding the existence of relevant priors on film.
Using simplistic set theory, the "set" of examinations available only on film could be defined as:
Set fi lm = Set RIS - Set PACS
where Set film is the set of film studies, Set RIS is the set of studies on the RIS, and Set PACS is the set of studies on the PACS.
Fortunately, UCSF's PACS vendor incorporated an applications programming software toolkit in its product. The RIS-PACS query tool created at UCSF is a Web application consisting of dynamic Web pages written in the Java Server Page (JSP) language running in a Tomcat Web server. All interaction with the RIS-PACS query tool takes place through a Web browser embedded within the PACS workstation's graphical user interface (GUI). The browser is invoked using the vendor's proprietary scripting language, which is used to capture the current clinical context (patient ID) and pass it to the query tool using a standard URL. This URL points to a JSP page that performs a Digital Imaging and Communications in Medicine (DICOM) standard query of the PACS and an HL-7 query of the RIS for all examinations related to the patient ID passed in the URL. The results are then collated using the set operation described above and presented to the user in a tabular format (Figure 1).
To allow users to quickly discern which studies are available on PACS and which are available only on film, the results of the set operation are presented in a color-coded format, with the PACS-based studies in gray and the film-only studies in white. Users can also access the diagnostic report associated with each examination by clicking the appropriate button on the page. Doing so opens a second browser window, which displays a separate JSP page that retrieves the report from the RIS and presents it to the user (Figure 2).
Further expanding upon the concept of Web integration and PACS was the creation of an emergency department (ED) "wet-read" application to enable ED physicians and radiologists to communicate rapidly with each other about urgent exam results (Figure 3). This custom Web-based workflow application uses an embedded Web form to electronically capture wet reads and deliver them to the ED physician (Figure 4). As with the RIS-PACS application, the wet-read application uses the PACS vendor's scripting language to add a custom button to the PACS workstation GUI, as well as to pass the clinical content to the Web application.
The application supports a variety of methods for transmitting wet reads to the ED. These include printing them out on ED-networked printers, making them available on ED-PACS workstations, sending them to the electronic medical record in the ED, and displaying them on wireless network-enabled personal digital assistants (PDAs) carried by the ED physicians (Figure 5). The application relies on the Java Message Service (JMS) framework to reliably deliver the wet reads to all these destinations.
The wet-read application also contains Web forms for the ED physicians to enter their findings if they happen to see the images before the radiologist does, and a quality assurance (Q/A) form for radiology attendings to "grade" wet reads entered by radiology residents. The results from the Q/A process are automatically emailed to the appropriate resident. Cases in which a significant discrepancy existed between the resident's and the attending's findings are entered into an electronic folder and presented at a monthly resident conference.
Conclusion
While such Web-based applications are more commonplace today, they were very innovative for their time. They made out-of-box PACS more useful. Web-based custom workflow solutions have not received the publicity they deserve, but they are continuing to be implemented in hospitals that strive to fine-tune the capabilities of their PACS investment.
REFERENCES
- Internet History--Lawrence Roberts. Available on the Living Internet Web site at: http://livinginternet.com/ i/ii_roberts.htm. Accessed April 2005.
- Strowger award: Lawrence Robert. Available on the J.W. McClure School of Communication Systems Management at the Ohio University Web site at: www.mcclureschool.info/strowger/roberts.htm.
- Tim Berners-Lee. Available on the World Wide Web Consortium (W3C) Web site at: www.w3.org/ People/Berners-Lee. Accessed April 2005.
- Tellis WM, Andriole KP, Jovaise CS, Avrin DE. RIS minus PACS equals film. J Digit Imaging. 2002;15 (suppl 1):20-26.