Musculoskeletal infections of the extremities: A tour from superficial to deep
Images
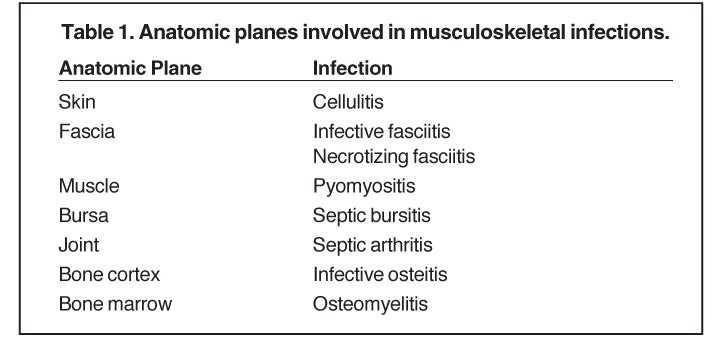
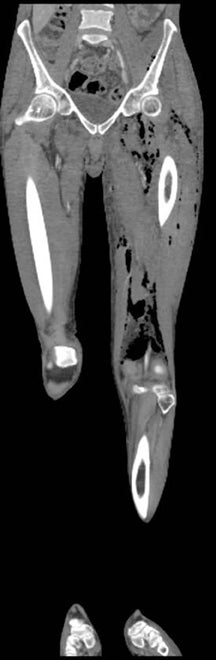
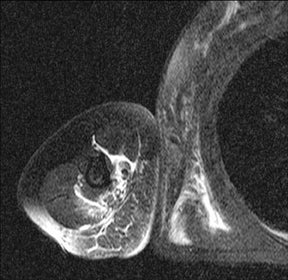
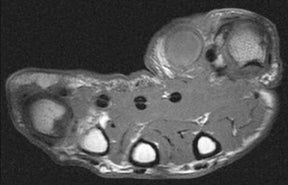
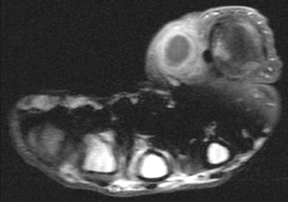
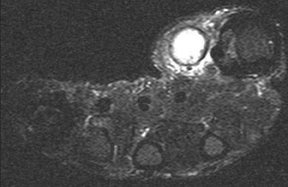
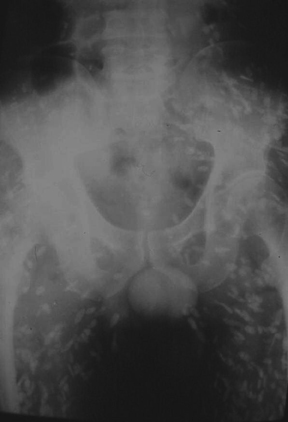
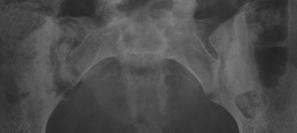
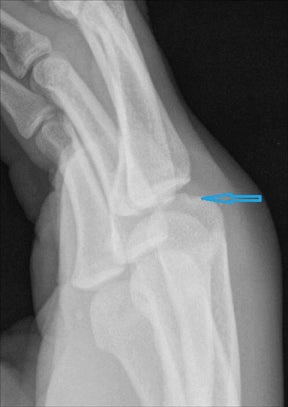
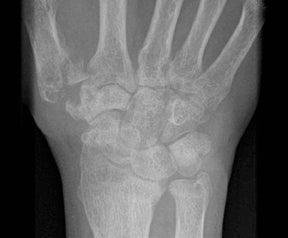
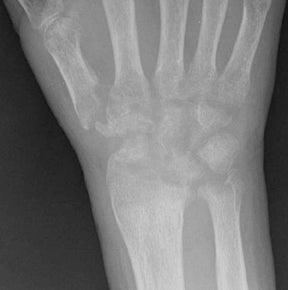
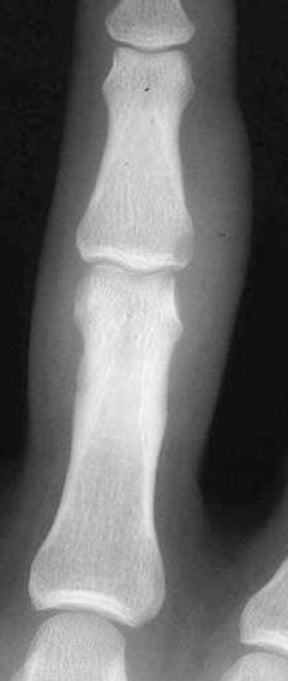
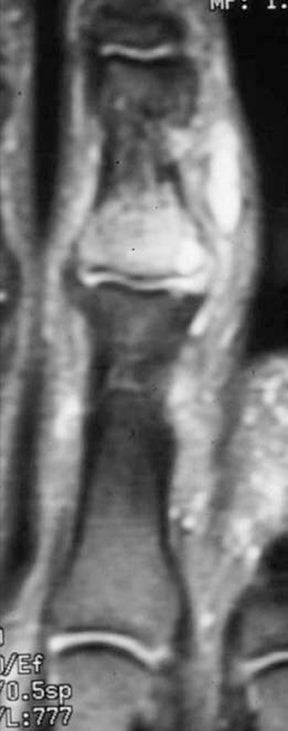
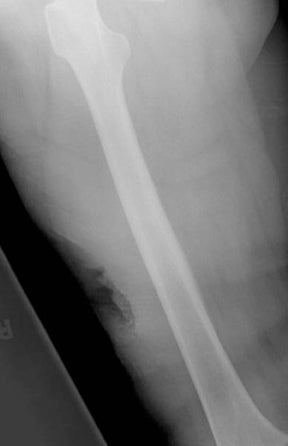
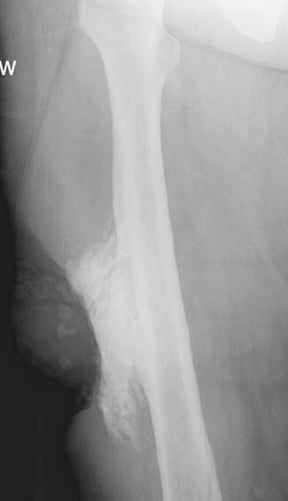
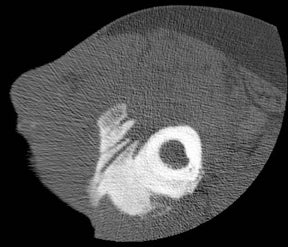
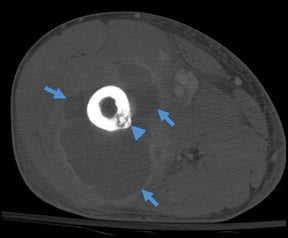
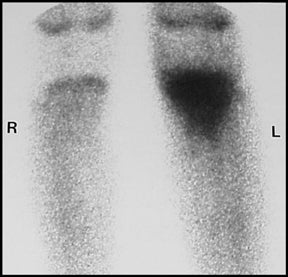
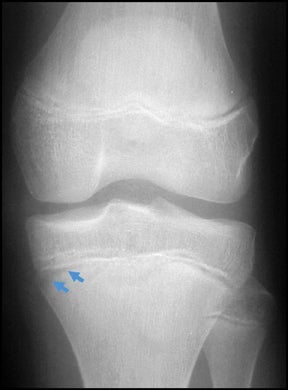
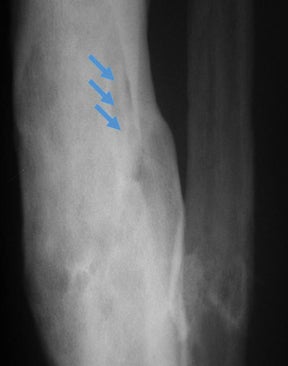
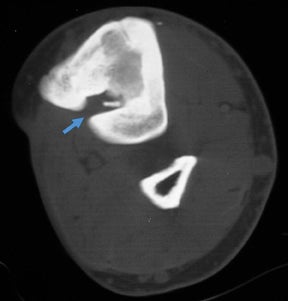
Dr. Stalcup is a Radiologist, Dr. Pathria is a Professor of Radiology, and Dr. Hughes is a Professor of Radiology and the Residency Program Director, in the Department of Radiology, University of California, San Diego, San Diego, CA.
Certain images and text from this publication were presented as an educational exhibit at the RSNA 2009 meeting.
Infection of the musculoskeletal system is commonly encountered in clinical practice. Treatment and prognosis depend upon the site of infection, the organism involved, and the underlying health of the patient. Delayed diagnosis can lead to significant morbidity and mortality, making rapid and accurate diagnosis crucial.1,2 Various anatomic planes can be involved (Table 1).
Infection of such tissue layers may coexist or develop sequentially, with osteomyelitis representing the most severe and deepest level. This article will review the imaging characteristics of infection involving the various layers of musculoskeletal tissue, starting in the superficial compartments and working progressively deeper to the bone marrow.
Cellulitis, fasciitis, soft -tissue abscess, and pyomyositis
Cellulitis is an acute infectious process limited to the skin and subcutaneous tissues.3 Risk factors include vascular insufficiency, soft-tissue ulcer (often secondary to diabetes), immunosuppression, recent trauma, and retained foreign bodies. Radiographic findings of cellulitis include skin thickening, nonspecific soft-tissue swelling, and obliteration of fat planes.4 On cross-sectional imaging, cellulitis results in thickening of the skin and the septae in the subcutaneous tissue. Occasionally, small fluid collections may be present in the subdermal areas or superficial to the fascia.4,5
Whereas “cellulitis” refers to infection superficial to the superficial fascia, the term “septic fasciitis” refers to infection that has extended to involve the fibrous fascia itself. Necrotizing fasciitis is a fulminant form of septic fasciitis associated with rapid spread of infection and prominent tissue necrosis.5 Necrotizing fasciitis is a surgical emergency, so rapid and accurate diagnosis is imperative.6 Occasional cases demonstrate small gas bubbles adjacent to fascial planes, affording specific radiographic diagnosis, but this finding has low sensitivity.5,6 Computed tomography (CT) and magnetic resonance imaging (MRI) are useful for noninvasive identification of perifascial gas and areas of tissue necrosis (Figure 1). Definitive diagnosis of necrotizing fasciitis is made by fascial biopsy, which should be undertaken if the imaging findings are equivocal.7
Chronic soft-tissue infection can result in the formation of an abscess. On radiographs, an abscess appears as a nonspecific soft-tissue mass; abscesses are difficult to diagnose with specificity, unless gas bubbles or gas-liquid levels are visualized.6 The CT appearance of an abscess is a heterogenous fluid collection with thick irregular margins that enhance after the administration of intravenous contrast.6 Inflammatory changes in the soft tissues adjacent to the abscess may lead to overestimation of its size. CT is particularly useful for detecting gas present within the abscess cavity. Both radiographs and CT can demonstrate retained foreign bodies that may be the cause of abscess, although CT is more sensitive.4
Soft-tissue abscesses have variable signal characteristics on MRI. The typical abscess shows central areas of low signal intensity on T1-weighted (T1W) images and high signal intensity on T2-weighted (T2W) images, though the proteinaceous granulation tissue on the inner margin may show intermediate signal on T1W images. The thick, irregular wall enhances after administration of intravenous gadolinium (Figure 2). Adjacent soft tissue and skin inflammation are generally present.2,6
Infection of muscle is referred to as “pyomyositis.” In the past, this disease process was most commonly encountered in children and in patients from the tropics; more recently, patients infected with HIV have been recognized to be at high risk for deep muscular infection.3,4,6 Diabetic patients and other patients with vascular compromise or immunosuppression are also at increased risk for muscle infection. The buttock and thigh are the most commonly affected locations, and Staphylococcus aureus (S. aureus) is the most common organism.3,4,6,8 The muscle is typically edematous, partially necrotic, and can contain multiple abscesses.
Muscle infection with extensive gas formation due to myonecrosis, also referred to as “gas gangrene,” is a rare condition that can be caused by multiple organisms, though it is classically associated with Clostridium perfringens.3 Patients are extremely ill, and the fatality rate is high.9
Parasitic infections of the soft tissues, particularly skeletal muscle, are rare in North America, though they are still commonly encountered in the developing world. The early infectious period produces a nonspecific focal myositis, but once the parasitic organisms die and calcify, they produce a characteristic appearance on radiographs. For example, cystercercosis (infection with Taenia solium, the pork tapeworm) produces calcified oval densities with lucent centers elongated in the longitudinal axis of the muscle fibers (Figure 3).10,11
Septic bursitis
“Septic bursitis” refers to infection of normally existing synovial-lined bursal cavities.12 Deep bursal infection is typically hematogenous, whereas the superficial bursae, such as those overlying the olecranon or patellar tendon, are often infected secondary to penetrating trauma.2 The most common organism is S. aureus,13 and the underlying joint is typically spared.2 Conventional radiographs typically demonstrate nonspecific soft-tissue fullness; gas formation within the bursa is uncommon. CT and MRI typically show a heterogeneous enhancing well-defined mass in the expected position of the bursa; surrounding inflammation is minimal.5 Septic bursitis can cause adjacent joint effusions, which are typically reactive, but the joint itself can be infected if the ligaments or tendons separating the bursa from the adjacent joint are disrupted.2 Aspiration of an adjacent inflamed joint may be necessary to exclude septic arthritis.
Septic arthritis
“Septic arthritis” can result from hematogenous spread to the synovial membrane, from direct inoculation, or from extension of contiguous metaphyseal osteomyelitis, particularly in children, in those locations where the metaphysis is intracapsular, such as the hip and shoulder.4 The most common organism causing septic arthritis across all age groups is S. aureus.4 Predisposing factors include intravenous drug abuse, immunocompromised state, rheumatoid arthritis, and foreign bodies such as joint prostheses.14 Pregnancy is an often overlooked risk factor. The increased laxity of the sacroiliac joints during pregnancy makes them prone to fluid accumulation and seeding during episodes of transient bacteremia (Figure 4).15
In children, septic arthritis involves the large joints of the extremities and is typically hematogenous in etiology.8 In adults, the etiology is usually direct inoculation from penetrating trauma, such as stepping on a nail or penetration of the metacarpophalangeal joint during a fight (Figure 5).4 Hematogenous spread in adults is less common than direct inoculation, but when it does occur it typically involves the 5 “S” joints: sacroiliac joint, symphysis pubis, sternoclavicular joint, spine, and acromioclavicular joint (shoulder).14,16 Major complications of septic arthritis include premature physeal closure, premature osteoarthritis, avascular necrosis, and adjacent osteomyelitis. These complications are more common and devastating in childhood septic arthritis.1,8
Radiographic findings of early septic arthritis include joint effusion, periarticular soft-tissue swelling, and aggressive periarticular osteopenia.5 Very early in the course of septic arthritis, particularly in young children whose joints are relatively lax, the joint space may be widened by a reactive effusion.4 Later in the course of the disease, joint space narrowing secondary to cartilage destruction and osseous erosions are identified.5 Joint-space loss is uniform and is not accompanied by sclerosis or productive bony changes, features which help differentiate infection from degenerative arthropathy. Any monoarticular destructive arthritis should be regarded as infectious until proven otherwise, and early diagnosis by aspiration is essential to prevent permanent joint damage.6
Tuberculous and fungal septic arthritis, as well as having a relatively indolent course have a distinctive radiographic appearance consisting of ill-defined erosions, profound osteoporosis, and relative preservation of joint space till late. This radiographic complex is referred to as “Phemister’s triad” (Figure 6).14,17 Any chronic inflammatory process of the synovium can lead to production of rice bodies, though tuberculosis is the classic process leading to their formation.18,19 Rice bodies represent thickened areas of synovial proliferation, typically detached from the underlying synovium. Rice bodies have a characteristic appearance on MRI, appearing as 5 mm to10 mm elongated fragments shaped like rice kernels, with intermediate T1W signal and low signal on T2W images.20 The differential diagnosis for rice bodies includes inflammatory arthropathies, such as psoriasis or rheumatoid arthritis, low-grade infections caused by fungus or tuberculosis, and sarcoidosis.18
CT is useful for identifying marginal erosions and guiding aspiration of joints with complex anatomy, such as the sacroiliac joint.6 On MRI, the findings of septic arthritis can be relatively minor, with a bland effusion seen in the early stage of the disease.6 At this stage, aspiration of the articulation is necessary for accurate diagnosis. Other MRI features of established septic arthritis include synovitis, periarticular soft-tissue inflammation, abscess formation, and periarticular bone edema.6 Differentiating between secondary osteomyelitis and reactive bone marrow edema with MRI can be extremely difficult. Cortical erosions, asymmetric edema on one side of the joint, and extensive marrow involvement suggest osteomyelitis rather than reactive marrow edema (Figure 7).21
Osteitis and cortical abscess
“Infective osteitis” refers to infection of the bone cortex, which can occur independently or concomitantly with osteomyelitis, an infection of the bone marrow.22 In patients with overlying soft-tissue ulcers, periosteal destruction leads to infective osteitis, which precedes transcortical penetration by organisms, and subsequent osteomyelitis.22 Differentiating true infection of the cortex from reactive periostitis secondary to overlying soft-tissue infection (Figure 8) is extremely difficult, as the rad-iographic appearance of these 2 entities overlaps considerably.22 Cortical infection should be suspected if the periostitis is ill-defined and irregular. There is intracortical lysis, particularly if the area of lysis is irregular and large, and if a lucent intracortical track leads away from an area of osteolysis.22 Cortical infection can lead to the development of an intracortical abscess, which can simulate the radiographic appearance of an osteoid osteoma6 (Figure 9), although an abscess is generally larger and more irregular.
Osteomyelitis
Infection of the bone marrow is referred to as “osteomyelitis.” The major routes of marrow infection are hematogenous, direct traumatic or iatrogenic implantation, and contiguous spread from infected soft tissues or adjacent articulations.2,23 Overall, S. aureus is the most common pathogen, though Group D streptococcus and Staph non-aureus are important causative organisms in the neonate.1 Patients with diaphyseal infarction due to sickle cell disease have an increased predilection for infection with Salmonella organisms,1 though S. aureus still remains the most common causative organism in sickle cell osteomyelitis.4,8
Osteomyelitis is distributed across all age groups, but is most common in early childhood. In children, the inoculation of bone is typically via the hematogenous route, and the metaphysis is the initial site of infection due to its slow, looping capillary blood supply.4 The blood vessels between the metaphysis and epiphysis do not close until after the first year of life, therefore, osteomyelitis in the infant can readily spread into the epiphysis and adjacent articulation.4 Involvement of multiple bones is also seen with increased frequency in infancy.4 Hematogenous osteomyelitis in the adult is less common than in childhood, with many adult infections resulting from direct spread from overlying soft-tissue infection. Adult osteomyelitis is associated with regional trauma (penetrating or surgical), intravenous drug abuse, immunosuppressed state, vascular compromise, and diabetes mellitus, particularly in the foot.2,3,5
Imaging characteristics
Radiographs are insensitive for early osteomyelitis. Radiographic findings of acute osteomyelitis are typically not present for 7 to 14 days following the onset of infection.1,5 The early radiographic manifestations of osteomyelitis consist of permeative metaphyseal osteolysis, endosteal erosions, intracortical fissuring and periostitis.3 Differentiation of osteomyelitis from other conditions producing periostitis and permeative bone lysis, such as Ewing sarcoma or stress fracture, can be difficult.2,6 Like radiography, CT is insensitive for early marrow infection, but is more sensitive for detection of early periostitis and trabecular or cortical erosion. In osteomyelitis, a difference of 20 Hounsfield units between the 2 extremities is suggestive of marrow infiltration with edema or cellular infiltrate and can be used as a marker of early osteomyelitis.2
Nuclear medicine detection of osteomyelitis is performed utilizing Technetium-99m methylene diphosphonate, either alone or in conjunction with Gallium-67 or Indium-111 labeled leukocytes.3 Scintigraphy is more sensitive than radiography and CT for early osteomyelitis and affords imaging of the entire skeleton (Figure 10).1,3 Uncommonly, marrow pressure may be sufficiently increased to produce hypoperfusion, resulting in a false-negative bone scan. In osteomyelitis, scintigraphy shows abnormal increased uptake on all 3 phases, with increasing activity on the delayed images.2,3 Gallium and Indium scanning are adjunct techniques that are particularly useful when there is an underlying disorder (e.g. trauma, tumor, surgery) that produces bone remodeling.1,6
MRI has been shown to be very sensitive for early osteomyelitis, and is currently the imaging examination of choice for detection of marrow infection.13 On MRI, osteomyelitis results in loss of the normal fatty signal of marrow on T1W images.5 This finding is less apparent in the infant and very young child, in whom the marrow is largely hematopoietic and little fatty marrow exists.2 On T2W and short tau inversion recovery (STIR) images, osteomyelitis results in increased signal intensity of the infected marrow space.6 Gadolinium enhancement is present, except in rare cases where the bone is poorly perfused due to vascular compromise from high marrow pressure or underlying vascular disease leading to bone infarction.6 MRI tends to overestimate the extent of infection due to difficulty distinguishing adjacent reactive edema from frank marrow infection.5
Chronic osteomyelitis
Chronic osteomyelitis can produce a variety of imaging abnormalities. A chronic medullary abscess, termed a “Brodie’s abscess,” is a lytic lesion with surrounding sclerosis, which represents a site of chronic active infection.6 Brodie’s abscesses are typically elongated in the long axis of the bone and are most commonly seen in the metaphysis of the proximal or distal tibia, characteristically surrounded by dense reactive bone sclerosis.1,2,6 The presence of a tortuous, linear channel connecting the lytic metaphyseal lesion to the physeal plate is pathognomonic of a Brodie’s abscess.6
Chronic osteomyelitis can result in the development of foci of necrotic cortical bone that act as a nidus for organisms and indicate ongoing active infection. The term “sequestrum” is applied to a segment of necrotic bone separated from the adjacent living bone by purulence and granulation tissue.2 The sequestered bone is not attached to the viable bone and is devascularized, making it difficult to treat with systemic antibiotics.4 Surgical resection is typically required for treatment of such necrotic bone fragments.1 The typical sequestrum is a longitudinally oriented fragment arising from the inner surface of the native osseous cortex. Radiographically, a sequestrum is denser than adjacent bone because it is devascularized and cannot participate in regional osteoporosis (Figure 11).6 CT is the preferred modality for identifying fragments of sequestered bone.1,4
The term “involucrum” refers to the layer of living bone that surrounds the sequestrum.23 The term “cloaca” refers to an open channel within the involucrum and is a site of drainage of pus and granulation tissue from the medullary space into the adjacent soft tissues to the skin.23 A cloaca is not present in all cases of chronic osteomyelitis.23 The purulent material draining outside the bone via the cloaca often produces a small focal abscess on the surface of the bone.6 Sinus tracks to the skin may develop after long standing chronic infection, and rarely long-standing tracks may develop squamous cell carcinoma (Marjolin’s ulcer) due to chronic skin irritation.24
Conclusion
Musculoskeletal infection can involve a number of different tissues, with different imaging appearances, depending on the depth and anatomic extent of tissue involvement. Such infection, particularly in children and in deep tissues such as the bone marrow, can lead to significant morbidity and mortality if not diagnosed accurately and treated appropriately. Cases of suspected musculoskeletal infection are commonly encountered by the diagnostic radiologist. It is hoped that this article will aid the radiologist in early diagnosis and categorization of the different layers of tissue involved with musculoskeletal infection.
References
- Turpin S, Lambert R. Role of scintigraphy in musculoskeletal and spinal infections. Radiol Clin North Am. 2001;39:169-189.
- Boutin RD, Brossmann J, Sartoris DJ, et al. Update on imaging of orthopedic infections. Orthop Clin North Am. 1998;29:41-66.
- Restrepo CS, Lemos DF, Gordillo H, et al. Imaging findings in musculoskeletal complications of AIDS. Radiographics. 2004;24:1029-1049.
- Kothari NA, Pelchovitz DJ, Meyer JS. Imaging of musculoskeletal infections. Radiol Clin North Am. 2001;39:653-671.
- Theodorou SJ, Theodorou DJ, Resnick D. Imaging findings of complications affecting the upper extremity in intravenous drug users: Featured cases. Emerg Radiol. 2008;15:227-239.
- Lalam RK, Cassar-Pullicino VN, Tins BJ. Magnetic resonance imaging of appendicular musculoskeletal infection. Top Magn Res Imaging. 2007;18:177-191.
- Trent JT, Kirsner RS. Diagnosing necrotizing fasciitis. Adv Skin Wound Care. 2002;15:135-138.
- Copley LAB. Musculoskeletal infection in children. Curr Opin Orthop. 2005;16:445-450.
- Resnick D, Niwayama G. Osteomyelitis, septic arthritis, and soft tissue infection: Organisms. In: Resnick D. Ed. Diagnosis of Bone and Joint Disorders, 3rd ed. Philadelphia, PA: W.B. Saunders Company; 1995:2448-2558.
- Garg RK. Neurocysticercosis: A pictorial review. Infect Dis Clin Pract. 2008;16:210-217.
- Herfel C, Strub WM, Leach JL. Twenty-four-year-old hispanic man with seizures: Imaging findings of cysticercosis. Infect Dis Clin Pract. 2006;14:93-96.
- Farooki S, Ashman CJ, Lee J, et al. Common bursae about the body: A review of normal anatomy and magnetic resonance imaging findings. Radiologist. 2002;9:209-221.
- Bureau NJ, Chhem RK, Cardinal, E. Musculoskeletal infections: US manifestations. Radiographics. 1999;19:1585-1592.
- Smith JW, Chalupa P, Hasan MS. Infectious arthritis: Clinical features, laboratory findings and treatment. Clin Microbiol Infect. 2006;12:309-314.
- Doita M, Yoshiya S, Nabeshima Y, et al. Acute pyogenic sacroiliitis without predisoposing conditions. Spine. 2003;28:E384-E389.
- Hagan IG, Burney K. Radiology of recreational drug abuse. Radiographics. 2007;27:919-940.
- Griffith JF, Kumta SM, Leung PC, et al. Imaging of musculoskeletal tuberculosis: A new look at an old disease. Clin Orthop Relat Res. 2002;398:32-39.
- Hsu CY, Lu HC, Shih TTF. Tuberculous infection of the wrist: MRI features. AJR Am J Roentgenol. 2004;183:623-628.
- Chau CLF, Griffith JF, Chan PT, et al. Rice-body formation in atypical mycobacterial tenosynovitis and bursitis: Findings on sonography and MR imaging. AJR Am J Roentgenol. 2003;180:1455-1459.
- Tan CHA, Rai SB, Chandy J. MRI appearances of multiple rice body formation in chronic subacromial and subdeltoid bursitis, in association with synovial chondromatosis. Clin Radiol. 2004;59:753-757.
- Karchevsky M, Schweitzer MR, Morrison WB, et al. MRI findings of septic arthritis and associated osteomyelitis in adults. AJR Am J Roentgenol. 2004;182:119-122.
- Resnick D, Niwayama G. Osteomyelitis, septic arthritis, and soft tissue infection: Mechanisms and situations. In: Resnick D. Ed. Diagnosis of Bone and Joint Disorders, 3rd ed. Philadelphia, PA: W.B. Saunders Company; 1995:2325-2418.
- Auh JS, Binns HJ, Katz BZ. Retrospective assessment of subacute or chronic osteomyelitis in children and young adults. Clin Pediatr. 2004;43:549-555.
- Smith J, Mello LFB, Neto NCN, et al. Malignancy in chronic ulcers and scars of the leg (Marjolin’s ulcer): A study of 21 patients. Skeletal Radiol. 2001;30:331-337.