Acute Wallerian Degeneration
By Llanes AC, Towbin RB, Schaefer CM, Towbin AJ
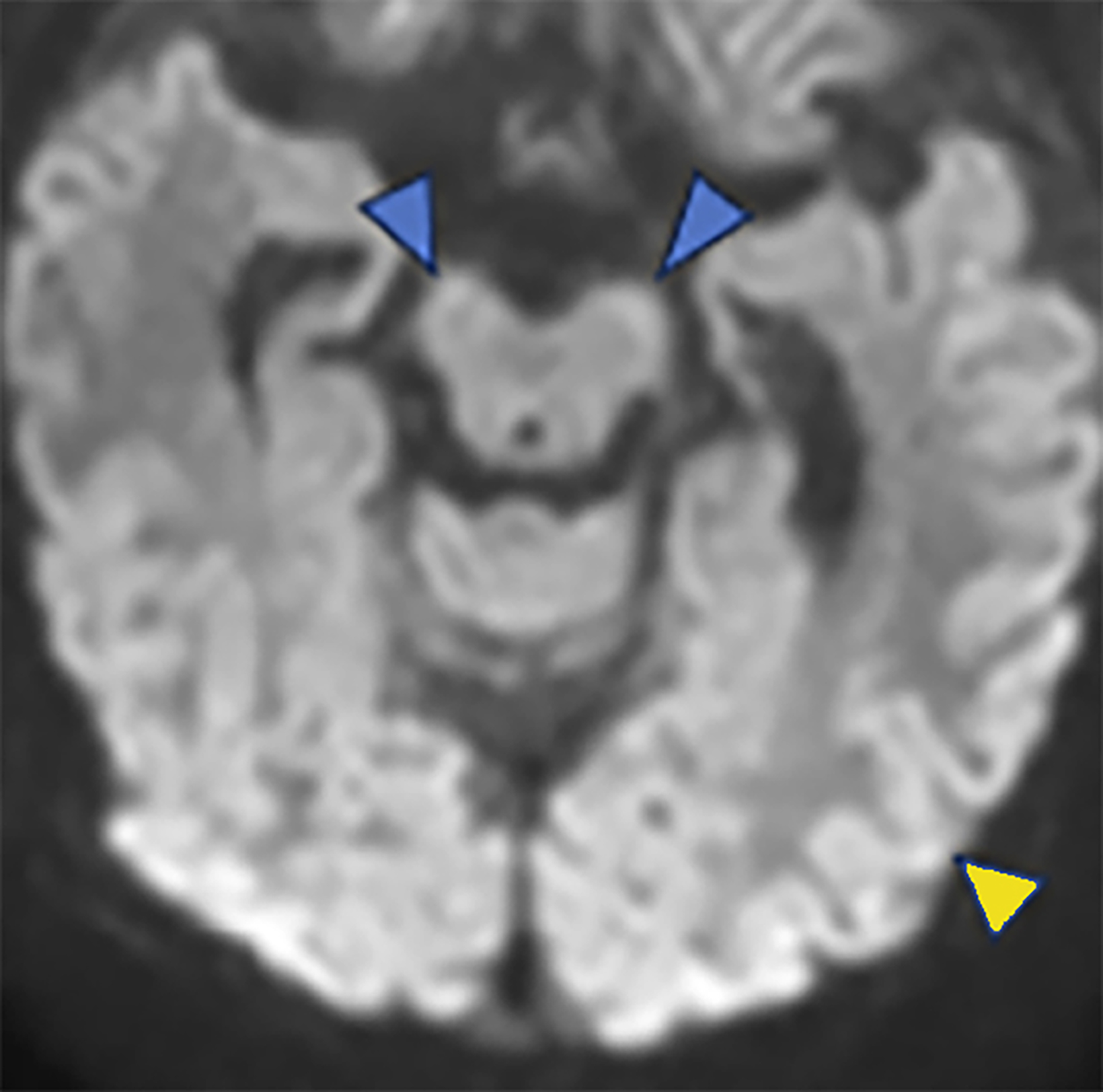
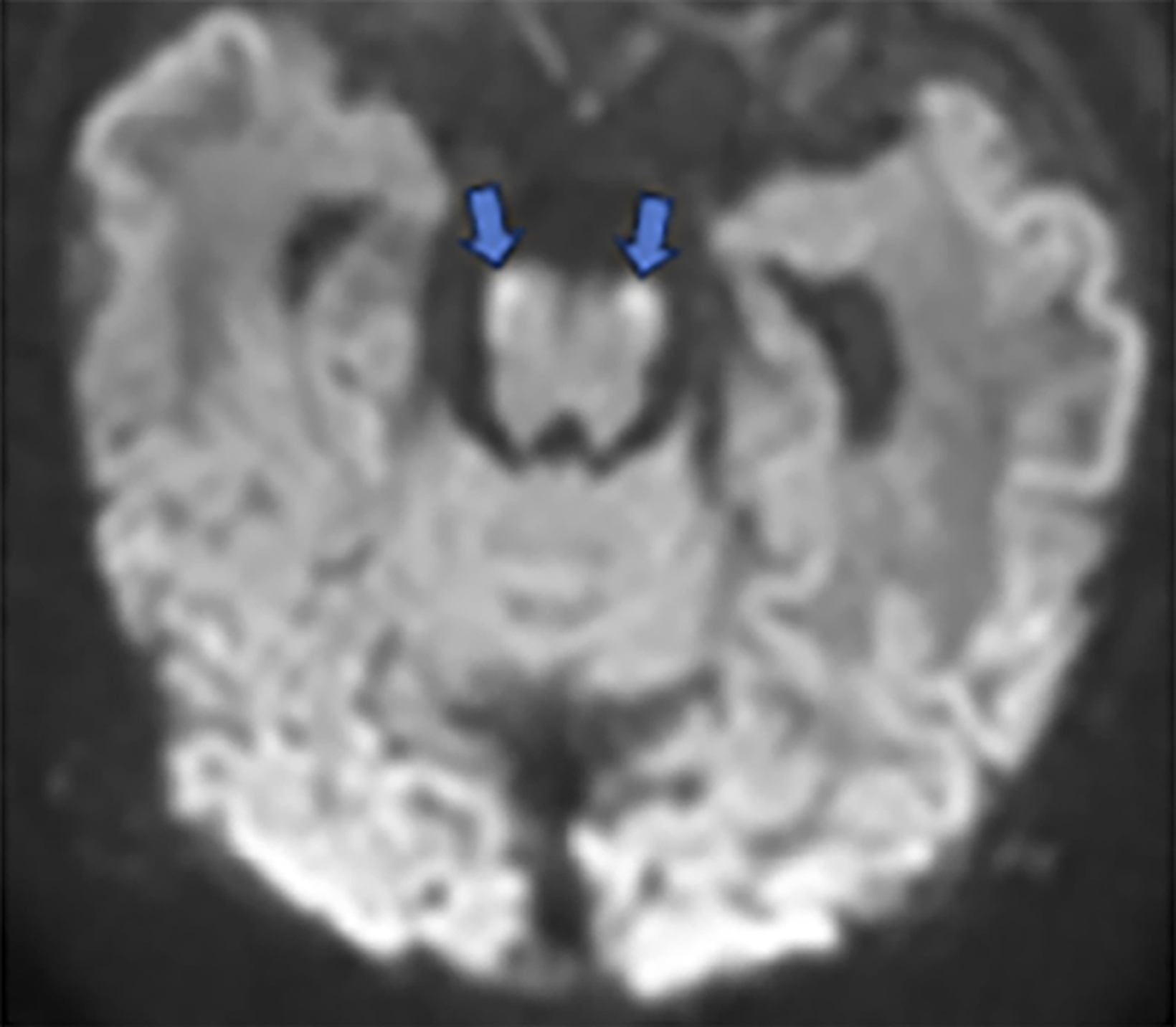
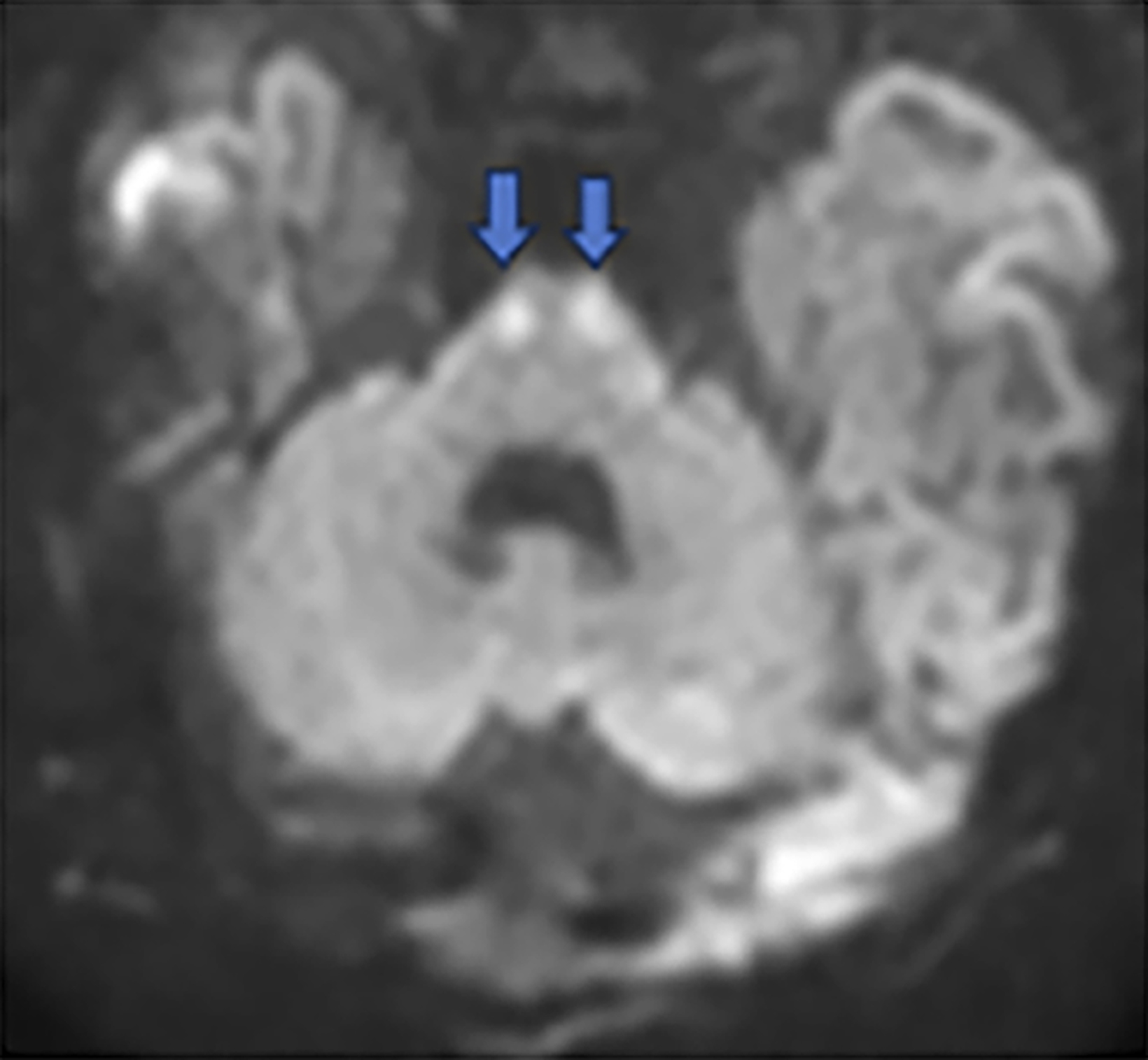
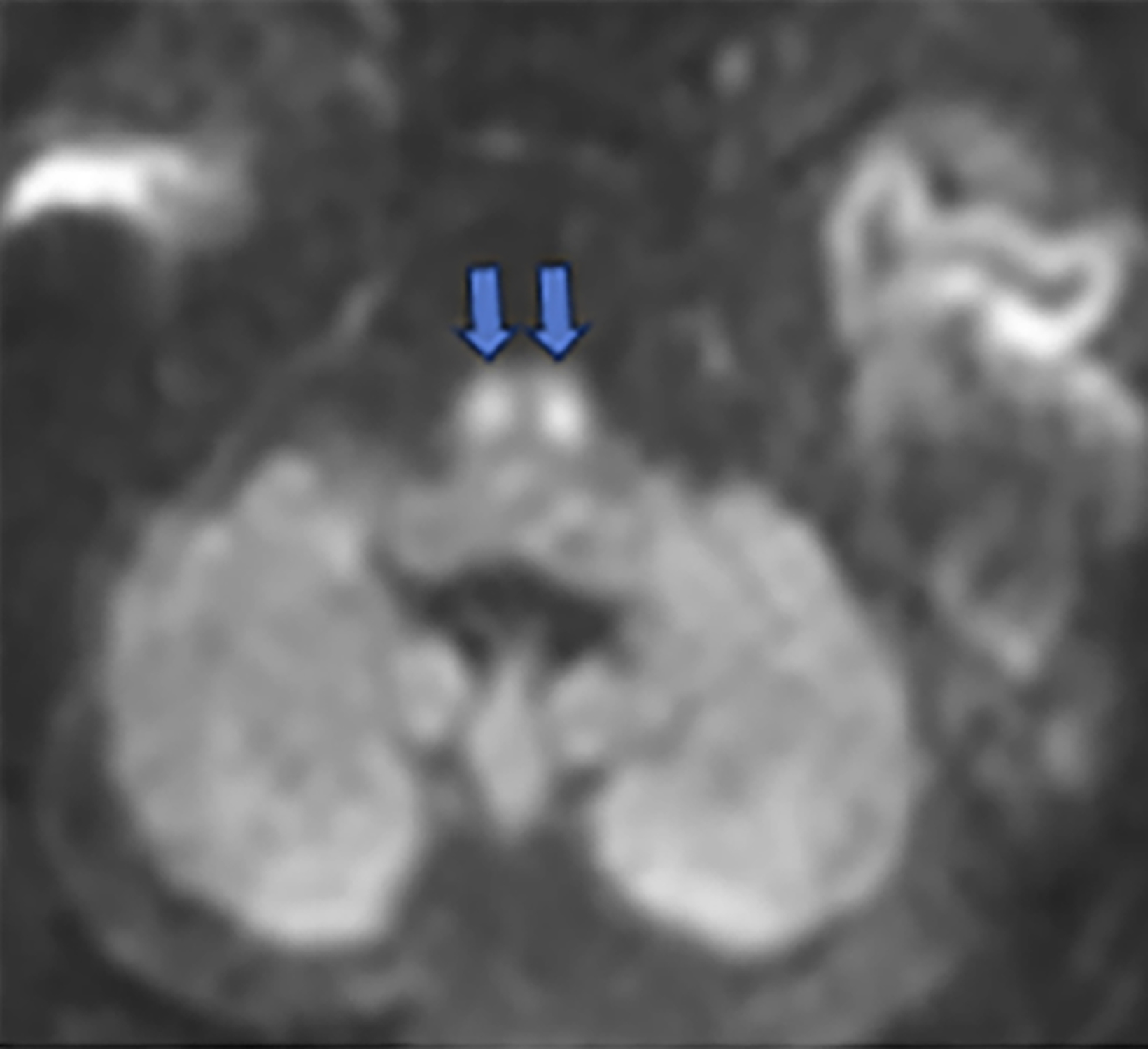
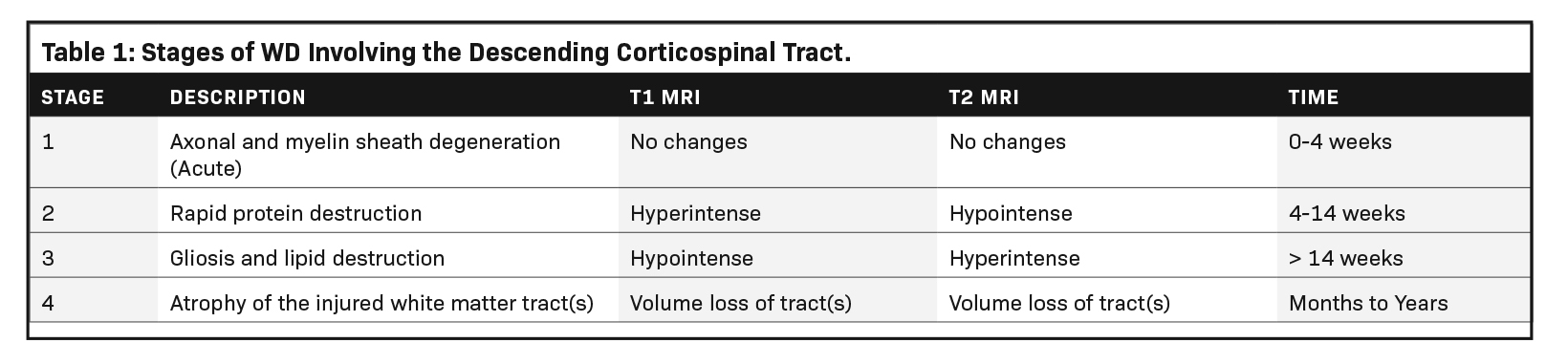
Case Summary
A term infant was born via emergency cesarean for fetal bradycardia. The neonate had Apgar scores of 0, 3, and 3 at 1, 5, and 10 minutes of age, respectively. Arterial umbilical cord gas showed a markedly low pH (6.510) and metabolic acidosis (base deficit 30.7) and the child was resuscitated. Physical examination revealed extreme hypotonia. The child was placed on a total body cooling protocol.
Imaging Findings
Brain MRI on day of life 19 demonstrated moderate lateral and third ventricular enlargement, diffuse bilateral periventricular white matter injury, and cortical and deep gray matter injury. In addition, restricted diffusion involved the entirety of the corticospi- nal tracts (CSTs), from the perirolandic cortices through the upper cervical spinal cord (Figure 1).
Diagnosis
Acute Wallerian degeneration.
Differential diagnosis includes traumatic injury, neurodegenerative disease, hemorrhage, cerebral infarction.
Discussion
Wallerian degeneration (WD) refers to the phenomenon of secondary degeneration of the associated axons and myelin sheaths in response to neuronal injury. The condition occurs in both the central and peripheral nervous systems as a reaction to axonal injury resulting from pathology, trauma, or neurodegeneration.1,2 However, neonatal brain injury is a common cause of WD and most often results from a profound hypoxic-ischemic injury, although neonatal arterial infarction, infection, and inflammation can also be causative. Regardless of etiology, the reaction proceeds through four distinct stages (Table 1).4
It has long been known that Schwann cells in the peripheral nervous system (PNS) and oligodendrocytes in the central nervous system (CNS) recruit macrophages and microglia, respectively, to clear microscopic debris resulting from axonal injury.6 Particularly in CNS-WD, recruitment of oligodendrocytes and microglia is also necessary for the formation of a glial scar, also known as gliosis.7 The clearance of molecular debris through phagocytosis is mediated by the interactions of complement receptor-3 (CR3) and scavenger receptor-AI/II (SRA) with complement-opsonized myelin.4
Additionally, interactions between CD47 on oligodendrocytes and signal-regulatory-protein-α (SIRPα) on healthy myelin are crucial for the inhibition of phagocytosis.4 Other discoveries have highlighted the role of modifier genes such as nicotinamide mononucleotide adenylyltransferase1 (NMNAT1) and sterile alpha and TIR motif containing 1 (SARM1) on WD initiation and the speed of the process.3 These genes hold potential as targets for further exploration of neurologic pathology, as well as candidates for therapy within translational research to promote axonal regeneration in CNS injury.
MRI has contributed to a better understanding of WD.8 Early studies of WD visualization demonstrated changes in signal intensities on diffusion-weighted MRI over weeks and months in patients who suffered cerebrovascular accidents to assess suspected WD.9 Additionally, studies have explored the potential of diffusion-weighted MRI to depict acute injury to descending CSTs not visible on CT or structural MRI sequences.10
These techniques have also proven valuable in prognostication of deficits and outcomes in WD. Although outcome of perinatal WD varies, as a rule, degree of impairment is highly associated with the degree of axonal damage and is also dependent on the regions affected. Groenendaal, et al, demonstrated a strong association between MRI-documented, acute Wallerian degeneration in neonates with hypoxic-ischemic injury and motor abnormalities.5
Hayakawa and colleagues found11 that acute WD detected on diffusion weighted imaging occurred in the CSTs and corpus callosum. Diffusion restriction in both areas was more often noted in the second week of life. The clinical outcome was unfavorable in infants with CST involvement.11 Greater degrees of hyperintense signal on T2 were associated with lower scores of evoked potentials, indicating loss of function.
While the process of WD has not yet been fully elucidated, efforts have been made to further understanding. For instance, a case report by Szymanski, et al, suggests that WD can occur before MRI-visible myelination, as they observed in the CSTs of a deceased neonate, challenging previous assertions that WD requires myelinated axons.12
Conclusion
Wallerian degeneration is a physiological response to axonal injury. The process involves the disintegration of the axons connected to the injury by fiber tracts. Acute WD can be identified with diffusion weighted imaging in the first two weeks of life and can be predictive of neurologic outcome.