MRI of the thoracic aorta
Images
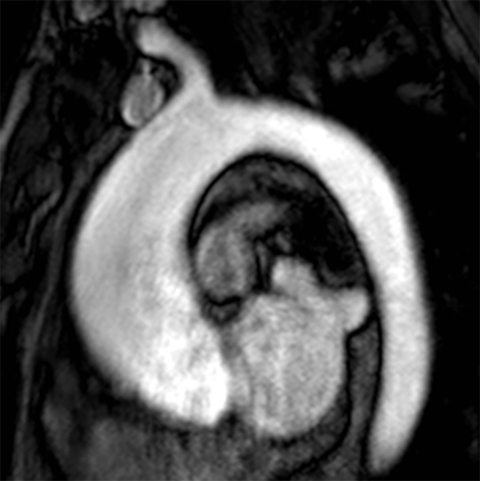
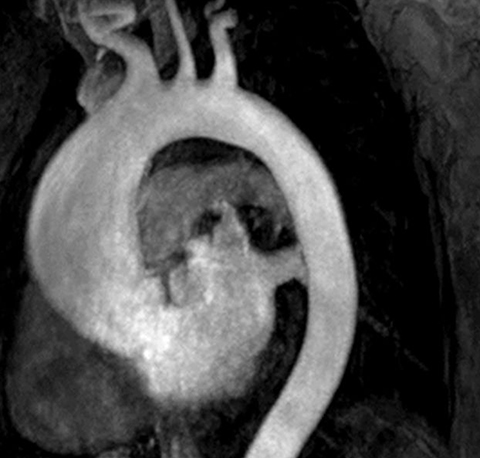
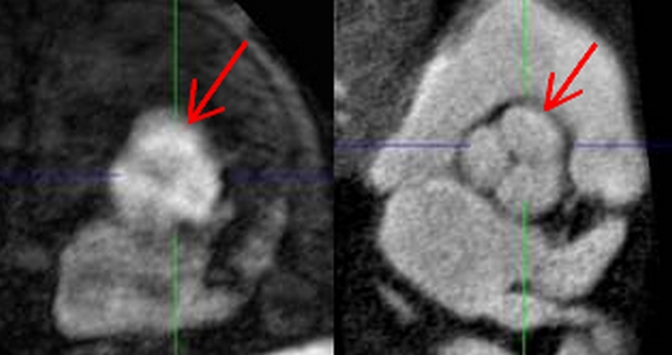
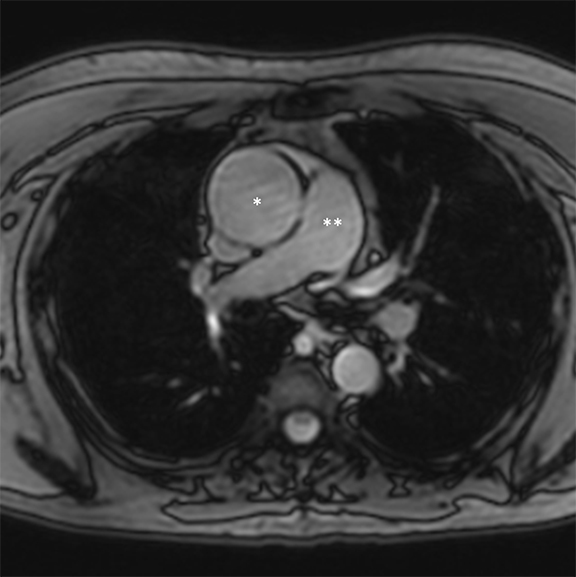
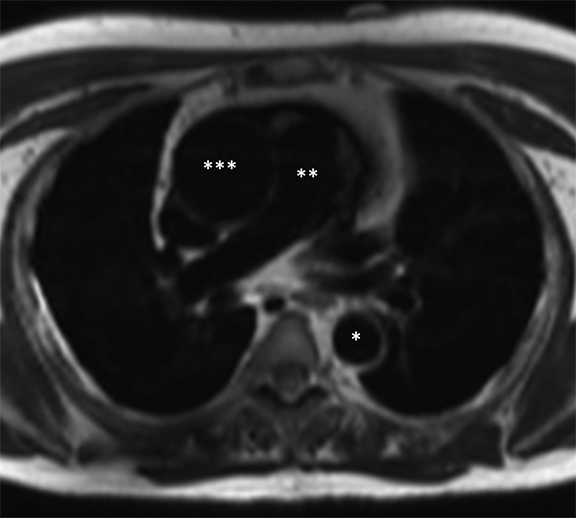
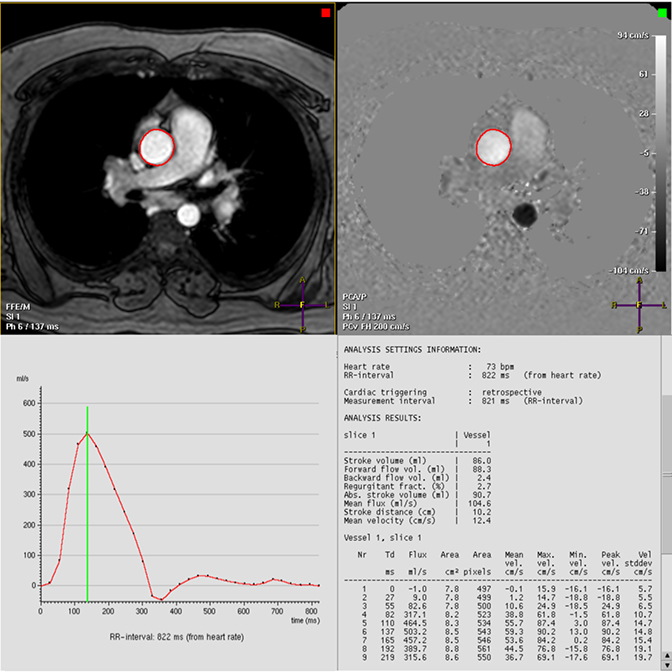
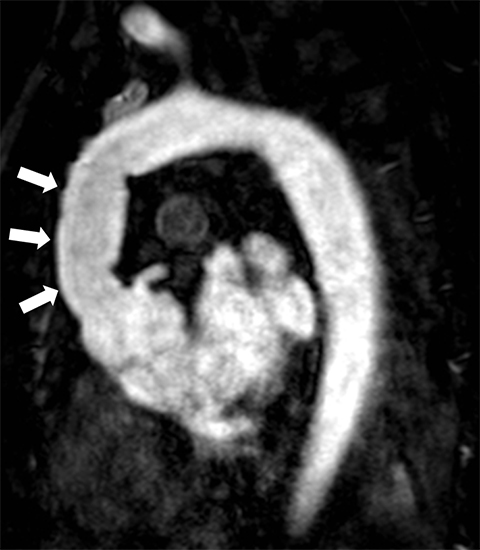
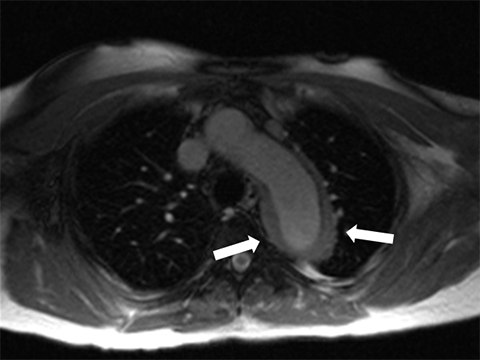
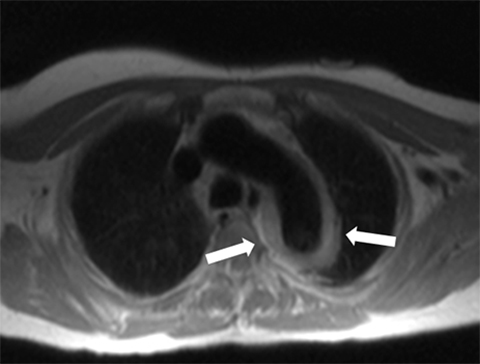
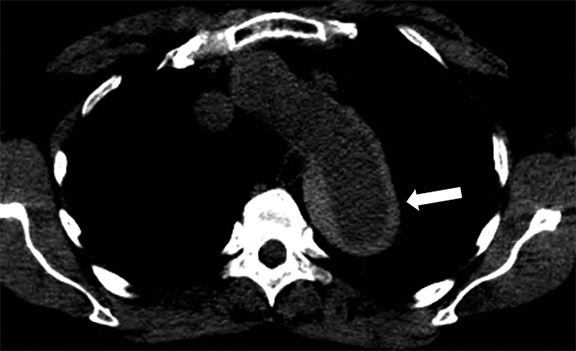
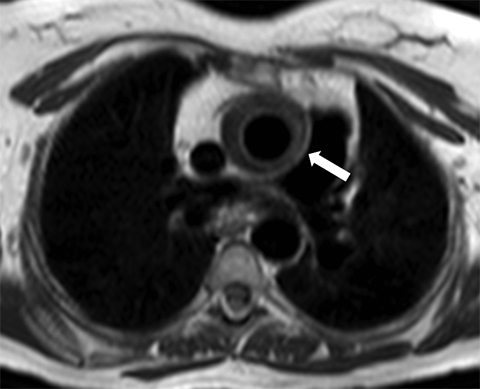
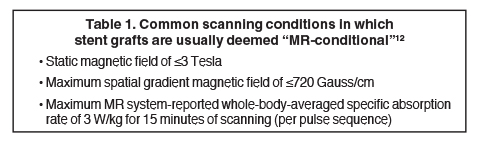
Imaging plays a key role in the clinical evaluation of aortic pathology.1 Magnetic resonance imaging (MRI) is a versatile modality in this setting, providing precise information about aortic anatomy and pathology, as well as physiologic flow and functional data. The combination of targeted sequences, multiplanar imaging, and lack of ionizing radiation with MRI allows for the assessment of a diverse range of aortic conditions without exposing the patient to ionizing radiation, and in some instances, without the need for intravenous contrast. In this article, we will review the role of MRI in thoracic aortic assessment, review a few common clinical applications, and describe the benefits and drawbacks of this modality in comparison to computed tomographic angiography (CTA).
MRI techniques
The technique most commonly used to image the lumen is contrast-enhanced MR angiography (CE-MRA).2 With this method, a gadolinium-based contrast material is injected into a peripheral vein (typically an antecubital vein); image acquisition occurs when the contrast reaches the arterial system for the first time (first-pass circulation) after circulating through the right heart chambers, pulmonary circulation, and left heart chambers. A 3D T1-weighted sequence is used for acquisition, yielding good contrast between the thoracic aorta and the veins and background tissues (Figure 1). Images are acquired during breath holds, typically of approximately 20 seconds. Multiplanar reformatting is performed to measure the aortic diameter in a true perpendicular plane to the vessel. CE-MRA can be performed with or without electrocardiogram (ECG) triggering, although there is evidence that ECG triggering may increase the precision of root and ascending segment depiction (Figure 2).3
The aortic lumen can also be imaged without intravenous administration of a contrast material.4,5 A commonly used sequence for this method is the balanced steady-state free-precession (bSSFP) sequence, with contrast determined by the T2/T1 ratios of the different tissues.6 As blood intrinsically has a high T2/T1 ratio when compared with the ratio of stationary tissues, this technique yields bright-blood images without the use of intravenous contrast. It must be noted that veins will also appear bright with this technique. bSSFP can be implemented as a 2D or 3D sequence (Figure 3). The 2D sequence can be obtained with ECG synchronization to provide cine images depicting aortic blood flow, as well as generating dark signal in the setting of dephasing caused by nonlaminar flow associated with valve stenosis or coarctation. The 3D sequence, which is most commonly used as a substitute for CE-MRA, can be used with or without ECG triggering. Because of the long scan time required for 3D implementation, respiratory triggering is used to remove respiratory motion artifacts. Research has shown that compared with CE-MRA, bSSFP in patients with thoracic aortic diseases provides equivalent measurements of aortic diameter, comparable sensitivity and specificity, and better visualization of the aortic root.7 Use of ECG-triggered technique does depend on a regular heart rate for successful implementation.
Dark-blood MRI techniques can be obtained with spin echo or inversion recovery sequences; with ing of signal from moving blood, the aortic wall is clearly depicted. The aortic wall anatomy and tissue characteristics may be defined based on T1, T2, and T2* signal characteristics. Many different methods are used to acquire black-blood images, including breath-hold, respiratory-triggered, and rapid single-shot techniques (Figure 4).
Phase-contrast (PC) sequences can be used to quantify aortic flow. PC quantification was founded on the observation that protons passing through a magnetic field gradient undergo a phase change in proportion to velocity. Aortic blood flow can be quantified by integrating these measured velocities within the aortic lumen during the cardiac cycle (Figure 5). This technique allows for evaluation of aortic forward flow and stenotic and regurgitant valves and is helpful in the assessment of congenital heart disease. PC imaging is most commonly acquired as a single through-plane or in-plane direction, with more recent developments allowing for flow analysis in multiple directions, albeit with longer acquisition time.
Selected MRI clinical applications
Thoracic aortic aneurysm
A thoracic aortic aneurysm (TAA) is defined as a permanent localized dilation of the thoracic aorta, in which the diameter is 50% larger than the normal aortic diameter.1 TAAs are usually asymptomatic and may develop undetected until an acute emergent event occurs, such as dissection or rupture.1 These acute events carry a high mortality rate, and studies have demonstrated that treatment of asymptomatic, stable TAAs leads to better results than treatment of dangerous acute events.1 Therefore, patients with known TAAs that have not yet met surgical size criteria are usually followed up with serial imaging studies to assess the maximum caliber and growth rate of the aneurysm, which allows clinicians to determine the correct timing for surgical intervention. Because patients are not exposed to ionizing radiation and nephrotoxic con trast media with this technique, MRI is an attractive option in this clinical setting8,9 particularly for younger patients with conditions placing them at higher risk for TAAs such as bicuspid aortic valve and Marfan, Ehlers-Danlos, and Loeys-Dietz syndromes.1,8
The MR method of choice to diagnose or follow up a TAA in patients with normal renal function is CE-MRA because of the contrast levels achieved and the depiction of large branch vessels that can be obtained with this technique. Multiplanar reformatting should be performed and the aortic diameter should be measured in reproducible anatomic positions, in a plane perpendicular to the direction of flow. Diameter should be measured from outer wall to outer wall.1 Cases in which severe or end-stage chronic renal disease is present (ie, estimated glomerular filtration rate [eGFR] <30 mL/min/1.73 m2), gadolinium-based materials should not be administered.10 A noncontrast MRA technique can be employed in these cases. Dark-blood techniques typically have supplementary roles in TAA examinations (e.g, when there is concomitant concern for aortic wall pathology).
TAAs can be corrected by open surgery or by endovascular stent placement.11 Open surgery is typically performed by implanting an impermeable Dacron tube, which is nonmetallic, MRI safe, and not associated with artifact; or by performing aortoplasty, which involves resection of a portion of the aortic wall (Figure 6). Thoracic endovascular stents, on the other hand, are usually constructed with a stainless steel mesh and are considered “MRI conditional” according to the current terminology of the American Society for Testing and Materials, meaning “an item that has been demonstrated to pose no known hazards in a specified MRI environment with specified conditions of use.”12-14 Conditions in which many of the available commercial stents were found to be safe are listed in Table 1.12 Specific and detailed information for each product can be obtained from the manufacturer. Although most endovascular stents can be safely imaged by an MRI system immediately after implantation in typical clinical conditions, susceptibility artifact often will degrade the images and render the treated aortic segment and its lumen impossible to evaluate, limiting the usefulness of MRI as a follow-up tool in this setting.
Because bicuspid aortic valve has been found to be an independent risk factor for TAA, many young patients with known bicuspid aortic valve are serially screened for developing aneurysms and may therefore benefit from the use of MRI.15 When these patients are imaged, the aortic valve can also be evaluated with cine and phase-contrast imaging to delineate leaflet opening and flow quantification, respectively.8 Further, left ventricle size and systolic function may be accurately quantified with cine bSSFP imaging when there is concern about ventricular remodeling associated with chronic valve dysfunction.
Acute aortic syndromes
Acute aortic syndromes are defined as a sudden occurrence of chest or back pain that is caused by an aortic disease. Acute aortic syndromes include intramural hematoma, aortic dissection, and penetrating aortic ulcer. Intramural hematoma is a consequence of the rupture of the vasa vasorum into the media of the aorta, without intima rupture.16 Dissection involves rupture of the intima and media of the aorta, with bleeding within and along the wall of the aorta.1 Penetrating aortic ulcer is defined as a focal atherosclerotic lesion that ulcerates, disrupting the internal elastic lamina of the aortic wall.17,18 These three presentations of acute aortic syndromes have similar symptoms, and each carries the risk of aortic rupture. The imaging method of choice for acute aortic syndromes in most instances is CTA because of its ready availability, precise anatomic depiction, and fast acquisition time. However, for cases in which administration of iodinated contrast material is contraindicated, MRA without intravenous contrast can be employed with good results if the patient’s condition is stable. The increased time of acquisition for MRI, alterations in ECG signal, and difficulty providing care to patients in the MRI scanner are limitations to consider for this technique. Because of the previously mentioned risk of radiation exposure with CTA, MRI may be considered for the follow-up of acute aortic syndromes. MRI can also be useful for evaluating intramural hematoma because of this technique’s good soft tissue delineation of the aortic wall (Figure 7).
Large vessel vasculitis
Large vessel vasculitis (LVV) refers to inflammatory diseases that involve the aorta and its major branches. There are several common subtypes of LVV. Takayasu arteritis most often occurs in women between the ages of 15 and 45 years, whereas giant cell arteritis is most commonly diagnosed in patients older than 50 years.19 Arterial wall inflammation and thickening in patients with LVV can lead to luminal stenosis or occlusion and associated end-organ ischemia (Figure 8). Less commonly, aneurysm formation and aortic valve insufficiency may ensue, in some cases leading to acute aortic pathology such as dissection or rupture.20 MRI is well suited to the serial evaluation of LVV, providing luminal and vessel wall assessment, with the potential to provide assessment of aortic flow and stiffness (by means of pulse wave velocity calculation) as part of the same comprehensive examination. 20
MRI and CTA in thoracic aorta assessment
CTA is the most commonly used method to image the thoracic aorta because of its availability in most healthcare facilities, rapid imaging acquisition times, relative ease of acquisition and interpretation, and good spatial resolution. In addition, ordering and interpreting physicians are generally familiar with this imaging method. However, MRI offers some potential advantages over CTA for thoracic aorta imaging.8,9 MRI does not expose the patient to ionizing radiation and uses gadolinium-based contrast agents, which have excellent safety profiles in properly screened patients.10 Although gadolinium-based contrast agents should not be used in patients with end-stage and severe chronic renal disease (eGFR <30 mL/min/1.73 m2) because of the risk of nephrogenic systemic fibrosis, these agents are not considered to be nephrotoxic.10 MRI allows for improved soft tissue characterization compared to CTA and can obtain aortic flow information as part of the same examination. Finally, MRI can, in some instances, be successfully performed without the administration of contrast media, whereas CTA requires the use of a contrast agent to depict the aortic lumen.
Conclusion
MRI is a versatile tool for clinical assessment of the thoracic aorta. CTA remains a ubiquitous imaging option in this setting because of its ease of use and rapid acquisition time. However, MRI is a viable option when there is concern about ionizing radiation or contrast toxicity or there is interest in a comprehensive imaging examination offering improved aortic wall characterization and with the potential to quantify aortic flow measurements and left ventricular function.
Acknowledgment
The authors wish to thank Megan Griffiths, scientific writer for the Imaging Institute, Cleveland Clinic, for her editorial assistance.
References
- Hiratzka LF, Bakris GL, Beckman JA, et al. 2010 ACCF/AHA/AATS/ACR/ASA/SCA/SCAI/SIR/STS/SVM Guidelines for the diagnosis and management of patients with thoracic aortic disease. A report of the American College of Cardiology Foundation/American Heart Association Task Force on Practice Guidelines, American Association for Thoracic Surgery, American College of Radiology, American Stroke Association, Society of Cardiovascular Anesthesiologists, Society for Cardiovascular Angiography and Interventions, Society of Interventional Radiology, Society of Thoracic Surgeons, and Society for Vascular Medicine [erratum in J Am Coll Cardiol. 2013;62(11):1039-1040]. J Am Coll Cardiol. 2010;55(14):e27-e129.
- Prince MR. Gadolinium-enhanced MR aortography. Radiology. 1994;191(1):155-164.
- Groves EM, Bireley W, Dill K, et al. Quantitative analysis of ECG-gated high-resolution contrast-enhanced MR angiography of the thoracic aorta. AJR Am J Roentgenol. 2007;188(2):522-528.
- Morita S, Masukawa A, Suzuki K, et al. Unenhanced MR angiography: Techniques and clinical applications in patients with chronic kidney disease. Radiographics. 2011;31(2):E13-E33.
- Miyazaki M, Lee VS. Nonenhanced MR angiography. Radiology. 2008;248(1):20-43.
- Chavhan GB, Babyn PS, Jankharia BG, et al. Steady-state MR imaging sequences: Physics, classification, and clinical applications. Radiographics. 2008;28(4):1147-1160.
- François CJ, Tuite D, Deshpande V, et al. Unenhanced MR angiography of the thoracic aorta: initial clinical evaluation. AJR Am J Roentgenol. 2008;190(4):902-906.
- Booher AM, Eagle KA. Diagnosis and management issues in thoracic aortic aneurysm. Am Heart J. 2011;162(1):38-46.
- Litmanovich D, Bankier AA, Cantin L, et al. CT and MRI in diseases of the aorta. AJR Am J Roentgenol. 2009;193(4):928-940.
- ACR Committee on Drugs and Contrast Media. ACR manual on contrast media. Version 9. American College of Radiology; 2013. http://www.acr.org/Quality-Safety/Resources/Contrast-Manual. Accessed September 24, 2014.
- Hoang JK, Martinez S, Hurwitz L. Imaging of the postoperative thoracic aorta: The spectrum of normal and abnormal findings. Semin Roentgenol. 2009;44(1):52-62.
- American Society for Testing and Materials (ASTM) International. Active Standard ASTM F2503: standard practice for marking medical devices and other items for safety in the magnetic resonance environment. West Conshohocken, PA: ASTM International; 2013, http://www.astm.org/Standards/F2503.htm. Accessed September 24, 2014.
- Shellock FG, Woods TO, Crues JV 3rd. MRI labeling information for implants and devices: Explanation of terminology. Radiology. 2009;253(1):26-30.
- MRISafety Web site. http://www.mrisafety.com. Accessed September 24, 2014.
- Agarwal PP, Chughtai A, Matzinger FR, et al. Multidetector CT of thoracic aortic aneurysms. Radiographics. 2009;29(2):537-552.
- Chao CP, Walker TG, Kalva SP. Natural history and CT appearances of aortic intramural hematoma. Radiographics. 2009;29(3):791-804.
- Bischoff MS, Geisbüsch P, Peters AS, et al. Penetrating aortic ulcer: Defining risks and therapeutic strategies. Herz. 2011;36(6):498-504.
- Hayashi H, Matsuoka Y, Sakamoto I, et al. Penetrating atherosclerotic ulcer of the aorta: Imaging features and disease concept. Radiographics. 2000;20(4):995-1005.
- Matsunaga N, Hayashi K, Sakamoto I, et al. Takayasu arteritis: MR manifestations and diagnosis of acute and chronic phase. J Magn Reson Imaging. 1998;8(2):406-414.
- Gotway MB, Araoz PA, Macedo TA, et al. Imaging findings in Takayasu’s arteritis. AJR Am J Roentgenol. 2005;184(6):1945-1950.