Implementation of digital mammography in the workplace
Images
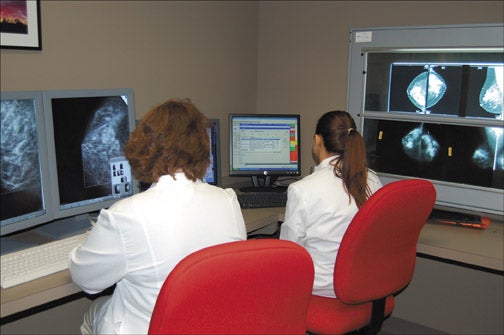
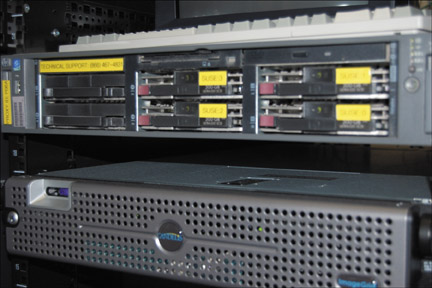
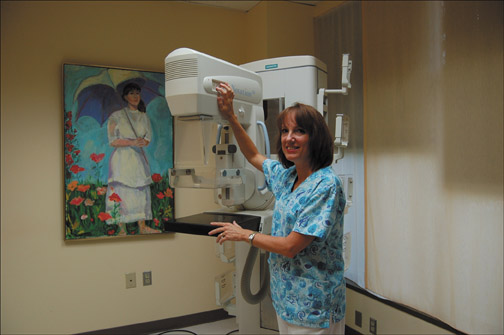
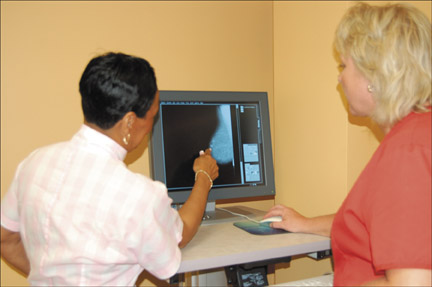
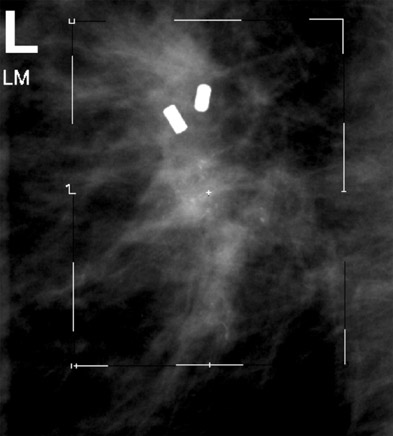
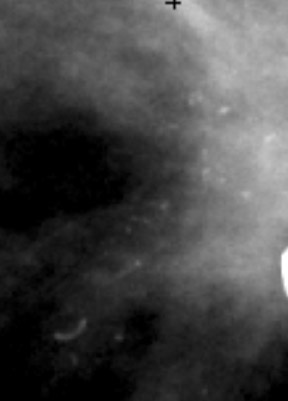
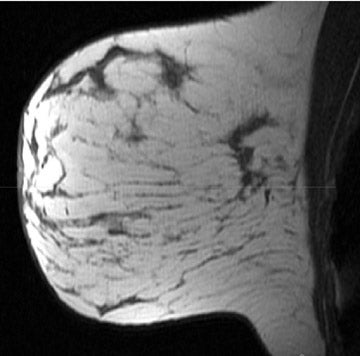
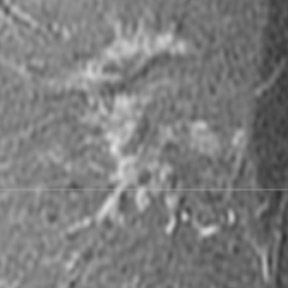
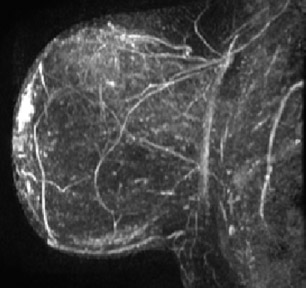
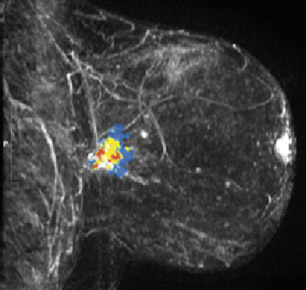
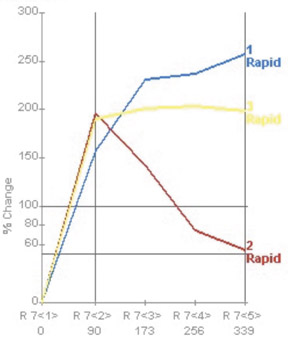
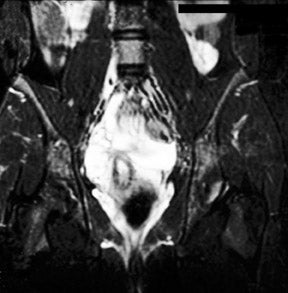
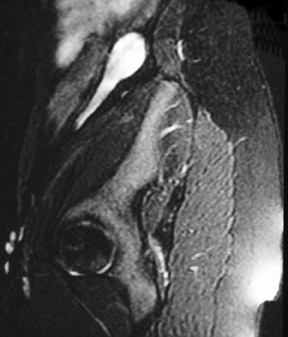
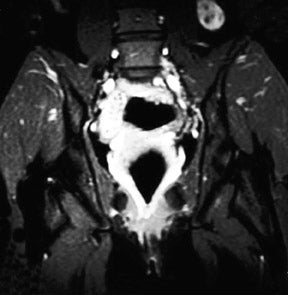
Dr. Paredes is the Founder and Director of The Paredes Institute for Women's Imaging, Glen Allen, VA. She is also a member of the editorial board of this journal.
Since the Food and Drug Administration (FDA) approval of full-field digital mammography (FFDM) for clinical use and the publication of the ACRIN Digital Mammographic Imaging Screening Trial (DMIST) trial results,1 the number of facilities transitioning from film screen mammography to digital mammography has grown rapidly. The DMIST trial confirmed the accuracy of digital mammography to detect breast cancer.1 Digital mammography has numerous advantages over film screen technology, and because of this, many new units being installed are FFDM equipment.
Because of the resolution requirements for mammography compared with other aspects of radiology, the development of technology for digital breast imaging has been challenging. There is a trade-off between a pixel size that is small enough for adequate resolution and a detector size that is large enough for breast imaging. In order to achieve a resolution that provided image quality at least as good as (if not better than) film screen mammography, some early detectors were smaller than those now in use. Unlike film screen mammography, for which 2 different film sizes are available, FFDM units are equipped with only 1 size of detector.
Many digital mammography workflow considerations exist and are different from those with film screen mammography. Workstation technology and display protocols have improved and have had a great impact on workflow for the radiologist. In this article, I will discuss the workflow considerations in the implementation of digital mammography in the clinical setting.
Clinical efficacy of digital mammography
First and foremost, for the new technology to be implemented, its accuracy cannot be less than that of a former technology. For digital mammography to receive FDA approval, this technology had to be validated as being at least as accurate as film screen mammography.
Several clinical trials have been conducted to evaluate the accuracy of digital screening mammography in breast cancer detection. The first clinical trial, conducted from 1997 to 2000, was the Colorado-Massachusetts trial of digital screening mammography.2,3 In this trial, 4521 women were evaluated by undergoing screening mammography on the same date on both a film screen unit and a prototype GE Healthcare digital unit (GE Healthcare, Waukesha, WI). There was no significant difference in cancer detection rates between analog and digital mammography, but there was a difference in recall rates. With digital mammography, the recall rate was significantly lower than that of film screen mammography.
The Oslo I trial evaluated 3683 women with both film and digital screening mammography.4 The study used a GE Healthcare digital unit and a workstation that was improved over that used in the earlier Colorado-Massachusetts study. Although more cancers were detected on film than on digital mammography, the result was not statistically significant. The Oslo II trial evaluated 25,263 women with either film or digital screening mammography.5 More cancers were detected with digital mammography than with film, but these results were not statistically significant.
In October 2005, the results of the ACRIN DMIST study were published by Dr. Etta Pisano.1 This keynote paper in the radiology literature reported the accuracy of digital screening mammography in breast cancer detection. Both film screen and digital screening mammograms were performed on the same day in 49,528 women. The types of digital equipment evaluated included GE Healthcare, Hologic, Fisher, and Fuji systems. Studies were read by independent radiologists, and patients with any abnormalities on either film or digital studies were recalled and evaluated.
The trial showed that there was no significant difference in breast cancer detection rates using analog versus digital equipment in the entire group of women studied. However, in subgroup analysis, there was a significant difference between digital and analog mammography. Digital mammography was superior to film screen mammography in the detection of breast cancer in 3 groups of women: those women younger than 50-years-old, premenopausal and perimenopausal women, and women with dense breasts.
Digital technology
Digital technology includes acquiring images either on a detector with digital radiography (DR) or on a plate with computerized radiography (CR). Currently, both types of systems are approved by the FDA. The images may be interpreted on high-resolution workstations or as laser-printed films.
There are 3 basic components to the imaging process: image acquisition, image review, and image storage (Table 1). With film screen mammography, all of these components are achieved using film. Therefore, all 3 components must be considered in the development or production of the film, and no individual component can be optimized. With digital mammography, these 3 components are separated and, therefore, each component may be individually optimized. This separation of imaging factors translates to the advantages realized with digital mammography.
Various types of detectors for digital mammography have been developed, and some types no longer exist. Early digital units involved "stitching" together multiple small charge-coupled devices (CCDs) or used a small oblong detector that "scanned" across the breast. With these techniques, the pixel size was small, providing a high resolution. However, problems with artifacts occurred in some cases, and these tech-nologies have since been discontinued. Current DR technology basically includes 3 types of units. The GE Healthcare unit has a 100-mm-resolution detector composed of a photodiode array over a chemical-shift imaging plate. The Hologic and Siemens DR detectors are direct digital detectors that acquire the image on an amorphous selenium plate and have a resolution of 70 mm. All of these units may be integrated with computer-aided detection (CAD) for an overread. The Fuji CR system is integrated with a mammographic unit, with which the image is captured on a CR plate and interpreted on laser-printed images.
Addition of digital mammography to an existing breast imaging facility
When planning to add digital mammography to an existing service, the following primary factors must be considered: choosing equipment, training staff, defining a process for patient selection for each unit, and developing a plan for image interpretation, networking, and archiving. A full list of considerations is shown in Table 2.
When choosing equipment, it is important to carefully consider access to service and speed of service response. Initially, the technologists will need more applications and service back-up when the equipment is first in use. One technologist should be selected for extended training and then designated to trouble shoot minor operational problems. Prior to performing digital mammography, to be accredited, all of the staff-including the radiologists-will need to complete continuing medical education credits specifically in digital mammography.
When organizing the workflow, it is very important to plan how to triage patients to film versus digital mammography. If all of the facility's analog units are being replaced with digital units, this is not an issue; but if some analog units will remain, triaging is exceedingly important. Various facilities handle the issue of triage differently. In some facilities, patients are triaged to digital mammography based on the reason for their visit (screening versus diagnostic). Others triage based on patient request, technologist and room preference, or a random order. Ideally, according to the results of the DMIST trial, triaging should be performed based upon patient age, menopausal status, and breast density. However, this method requires much more attention by the schedulers and technologists and can be difficult to implement.
The design and layout of the work area is different from that for analog mammography. No darkroom is needed for facilities that are fully digital, but a location for a laser printer is required. The technologists' workstation can be smaller because the images are not reviewed on view-boxes, and films are not handled and labeled. The digital mammography room is slightly larger than an analog room.
The reading room design for digital mammography is different from that for interpreting film mammography. The radiologists' reading station includes 2 high-resolution interpretation workstations (2.5 × 2.0K), a monitor that is used to view the worklist of patients and to control the workstation, a set of viewboxes for review of prior films, and a computer for the radiology reporting system. For easy image comparison, it is ideal to place the viewboxes at a 90˚ angle from the work station (Figure 1).
If prior images will be reviewed on films, it is critical to establish a method to organize the old films. It is helpful to have someone handle the prior films while the digital images are being read or to have a multiviewer loaded with the films. Alternatively, the prior images may be digitized for comparison purposes.
Digital mammography workflow is generally an improvement compared with that of analog mammography. Advantages in workflow with digita systems include the following: faster and more accurate retrieval of prior digital images, faster mammography room throughput, improved technologist productivity, and rapid transmission of images for interpretation, even remotely. The technologist focuses her attention on talking with the patient, collecting her history, and performing the mammogram. With digital imaging, the time-consuming aspect of darkroom work and film processing is eliminated. Images may be laser printed, which is an additional step, but this is not a routine procedure in the vast majority of facilities.
When digital mammography is implemented, radiologist productivity may be reduced, at least initially. A radiologist who is not used to working with a picture archiving and communication system (PACS) workstation may be quite slow at first in reading at the workstation. However, we have found the radiologists in our practice to be more efficient at the workstation than with film.
The plan for image archiving is a critical step that ensures that images can be retrieved. Options for storage are archiving in an on-site PACS, an off-site storage solution, or even keeping laser-printed films. However, storing printed films defeats one of the great advantages of digital mammography, which is improved archiving and the ability to rapidly retrieve images and files. In our own facility, images are stored on a central server (Figure 2), which contains mammograms from the prior 15 months. Images are also maintained permanently in 2 remote archives. Redundancy in the archive is extremely important to ensure that digital images are not lost. Off-site storage in digital archives can be an excellent option for smaller outpatient facilities that are not linked to a hospital PACS system.
The network for transmission of images to the PACS, to off-site storage, to the reading room, and to off-site interpretation workstations (in the case of telemammography) must be carefully defined prior to implementing the digital technology. Proper support for the network is also critical to the success of the program.
In creating a business plan for digital mammography, a simple financial analysis that focuses on the cost of the equipment is inadequate. Numerous factors influence the financial viability of the digital mammography project (Table 3). The impact of the improved workflow of digital mammography greatly affects the overall financial analysis. In our practice, the technologists are easily able to perform 1.5 to 2 times more mammographic studies with digital equipment (Figure 3) than they were with analog systems. This is, in part, because of the immediate image review in the mammography room for positioning and technique (Figure 4) and because there is no need for film processing and handling. We have also observed a 2- to 2.5-fold increase in the number of studies being performed on a digital unit versus an analog unit.
Therefore, in implementing digital mammography, fewer units are needed than would be needed for film screen mammography.
While planning a new digital mammography project, marketing efforts are helpful to establish study volume. Certainly, the referring physicians should be informed of the new program. For many women, digital mammography is an important, sought-after technology, so informing the public will help to build the mammography volume. The addition of digital mammography reflects a state-of-the-art service, and it is helpful to inform the potential population of clinicians and patients.
As with any new technology that is added to an imaging department, there is a learning curve with digital mammography. We have found that careful planning and preparation have made the implementation of digital mammography easier. The staff will quickly become comfortable and efficient with the new technology, and the workflow will reflect the assimilation of digital mammography into the clinical setting.
REFERENCES
- Pisano ED, Gatsonis C, Hendrick E, et al. Digital Mammographic Imaging Screening Trial (DMIST) Investigators Group. Diagnostic performance of digital versus film mammography for breast-cancer screening. N Engl J Med. 2005;27:353:1773-1783. Erratum in: N Engl J Med. 2006;355:1840.
- Lewin JM, Hendrick RE, D'Orsi CJ, et al. Comparison of full-field digital mammography to screen-film mammography for cancer detection: Results of 4,945 paired examinations. Radiology. 2001;218:873-880. Comment in: Radiology. 2001;221:273.
- Lewin JM, D'Orsi CJ, Hendrick RE, et al. Clinical comparison of full-field digital mammography to screen-film mammography for detection of breast cancer. AJR Am J Roentgenol. 2002;179:671-677.
- Skaane P, Young K, Skjennald A. Population-based mammography screening: Comparison of screen-film and full-field digital mammography with soft-copy reading-Oslo I Study. Radiology. 2003;229:877-884.
- Skaane P, Skjennald A. Screen-film mammography versus full-field digital mammography with soft-copy reading: Randomized trial in a population-based screening program-the Oslo II Study. Radiology. 2004;232:197-204.
Prepared by Dr. Margolies, Assistant Professor of Radiology and Director of Breast Imaging, The Mount Sinai Medical Center, New York, NY.
CASE SUMMARY
The patient is a 32-year-old nulliparous woman who complained of a new painless palpable mass in her left breast. She had no family history of breast cancer. Mammography was ordered.
IMAGING FINDINGS
On mammography, multiple masses with numerous microcalcifications were found. Two areas were selected for stereotactic biopsy, and both samples were revealed to be poorly differentiated breast cancer (Figure 1). Preoperative magnetic resonance imaging (MRI) showed the cancer as a mass with irregular margins (Figures 2 through 4). The mass had heterogeneous enhancement including washout kinetics, which is typical for breast cancer (Figure 5). The index mass exhibited all 3 classic MRI features of breast cancer: abnormal morphology, abnormal enhancement, and an abnormal washout kinetic enhancement pattern (Figures 2 through 5). No additional lesions were identified.
DIAGNOSIS
Ductal carcinoma and infiltrating lobular carcinoma
SURGICAL FINDINGS
At surgery, the patient was found to have a mixed, infiltrating, predominantly poorly differentiated ductal carcinoma and infiltrating lobular carcinoma with an extensive intraductal component and extensive lymphatic invasion. The tumor was estrogen- and progesterone-recep-tor-positive, and the immunohistochemical stain for HER-2-NEU onco- protein was positive. The sentinel lymph node indicated microscopic metastatic disease. While the patient was a candidate for breast conservation therapy, she elected to undergo a mastectomy.
DISCUSSION
Approximately 5% of newly diagnosed breast cancers occur in women under the age of 401 who do not undergo screening unless they are at high risk. In this age group, women who have breast cancer most often present with a painless palpable mass. Patients younger than age 36 usually have larger tumors and more nodal involvement and are likely to have stage II or III cancers at presentation. Poorly differentiated cancers are more common in the younger population, and these patients are more likely to undergo mastectomy.1,2 In one study, 12% of these young patients were found to have a contralateral breast cancer at the time of diagnosis or to have developed it later.3
Multiple studies have reported the ability of breast MRI to detect breast cancers that mammography and sonography have missed. In an analysis of multiple published papers, Peters et al4 found a 90% sensitivity and a 72% specificity for contrast-enhanced MRI. In women who have been found to have breast cancer, additional cancers in the same breast have been found in 11% to 31% of women.5 A study by Pediconi and colleagues6 reported that 24% of 118 patients with breast cancer had a contralateral breast cancer that was detected only by MRI-ie, missed by mammography and ultrasound. The majority of the additional cancers occurred in patients with heterogeneously dense or extremely dense breasts.6 Some advocate preoperative MRI for the assessment of tumor extent and to determine the presence or absence of additional foci of tumor in the same or the contralateral breast. Others, however, are concerned that the additional foci of cancer that are found may not be significant and may not impact survival. They note that there have been no randomized trials to show an improvement in disease-free survival.7,8 The role for preoperative MRI in breast cancer is yet to be determined; more studies are forthcoming.
CONCLUSION
Breast cancer in women who are too young for mammographic screening is often detected by self-examination. It is often diagnosed at a later stage and carries a worse prognosis than screening-detected cancers. It is incumbent on radiologists to remind our primary care colleagues to pursue radiology work-up of palpable findings in young women.
MRI finds cancers that are missed by mammography and ultrasound. Preoperative MRI shows additional foci of cancer, including ipsilateral and contra lateral cancers in a significant number of patients, and should be considered for presurgical planning for patients newly diagnosed with breast cancer.
REFERENCES
- Surveillance, Epidemiology, and End Results Program, 1973-2002. Bethesda, MD: Division of Cancer Control and Population Sciences, National Cancer Institute;2005.
- Gajdos C, Tartter PI, Bleiweiss IJ, et al. Stage 0 to stage III breast cancer in young women. J Am Coll Surg. 2000;190:523-529.
- Max MH, Klamer TW. Breast cancer in 120 women under 35 years old. A 10-year community-wide survey. Am Surg. 1984;50:23-25.
- Peters NH, Borel Rinkes IH, Zuithoff NP, et al. Meta-analysis of MR imaging in the diagnosis of breast lesions. Radiology. 2008;246:116-124.
- Morrow M, Freedman G. A clinical oncology perspective on the use of breast MR. Magn Reson Imaging Clin N Am. 2006;14:363-378.
- Pediconi F, Catalano C, Roselli A, et al. Contrast-enhanced MR mammography for evaluation of the contralateral breast in patients with diagnosed unilateral breast cancer or high-risk lesions. Radiology. 2007;243:670-680.
- Solin LJ, Orel SG, Hwang WT, et al. Relationship of breast magnetic resonance imaging to outcome after breast-conservation treatment with radiation for women with early-stage invasive breast carcinoma or ductal carcinoma in situ. J Clin Oncol. 2008;26:386-391.
- Morrow M. Magnetic resonance imaging in the breast cancer patient: Curb your enthusiasm. J Clin Oncol.2008;26:352-353. Comment on: J Clin Oncol. 2008;26:386-391.
Prepared by Martin C. Price, MD, Henry Ford Hospital, Detroit, MI, and Edsa M. Negussie, MD, Providence Hospital, Department of Radiology, Southfield, MI.
CASE SUMMARY
The patient is a 12-year-old girl who had presented to her pediatrician with chronic, intermittent left lower quadrant abdominal pain. The patient's medical history included gastritis confirmed by endoscopic gastroduodenoscopy, mild pervasive development disorder, and menarche at age 12 along with metrorrhagia. Prior imaging with computed tomography (CT) revealed a 3-cm well-demarcated, heterogeneous, soft tissue mass that was visualized in the left retroperitoneum (not shown). The mass was thought to represent a possible complex gastrointestinal duplication cyst. An ultrasound of the pelvis revealed a prominent normal-appearing right ovary posterior to the uterus and no identifiable left ovary (not shown). Abdominal magnetic resonance imaging (MRI) was performed (Figure 1).
IMAGING FINDINGS
MRI of the pelvis was performed using localizer coronal spin-echo T1-weighted (T1W) (time to repetition [TR] 500 msec, echo time [TE] 15 msec), fat-saturated coronal and sagittal spin-echo T2-weighted (T2W) (TR 4466 msec, effective [eff] TE 102 msec) and axial spin-echo T1W (TR 616 msec, TE 21 msec) and T2W (TR 4000 msec, TE 105 msec) sequences prior to intravenous gadolinium contrast administration and postgadolinium fat-saturated coronal and axial T1W (TR 650 msec, TE 20 msec) sequences. This displayed a 3-cm heterogeneous mass, lateral to the psoas muscle and posterior to the descending colon, corresponding to the earlier CT findings (Figure 1). Several high T2 signal foci within the mass were representative of follicular changes. A soft tissue structure representing the elongated utero-ovarian ligament was identified extending from the mass inferiorly to the left adnexal region (Figure 1B). This mass was determined to be an undescended left ovary based on its follicular pattern, position, and relation to the utero-ovarian ligament. The other findings included a normally positioned uterus with a single endometrial canal. The right ovary was visualized in its normal position with follicular changes present.
DIAGNOSIS
Embryologic ectopic ovary
DISCUSSION
Ectopic ovary is a very rare condition, with <50 cases reported in the literature. A classification system has been developed regarding ectopic ovaries and was first used by Wharton1 in his original 1959 report. Wharton categorized ectopic ovaries as either supernumerary (the presence of ovarian tissue-without an attachment to a normal ovary-in addition to 2 ovaries) or accessory (an ovary with an attachment to a normalovary).1 A subsequent recategorization according to Lachman et al,2 has established the nom enclature of ectopic ovary with subclassifications of either postsurgical implant, postinflammatory implant, or true (embryologic) ovary.
According to the modified categorization, the anomaly presented in this case report (given its attachment to the utero-ovarian ligament) fits into the later category of an embryologic ovary. In this report, we prefer to refer to this case as an undescended ovary subtype of embryologic ovary, as this patient has 1 ovary in a normal location with the other in an abnormal location subsequent to failed embryological descent. The gubernaculum, a cord of mesenchyme attached to the lower pole of the ovary, gives rise to the ovarian ligament and the round ligament of the uterus, while the parameso-nephric duct gives rise to the fallopian tube and uterine canal. The ovary, guided by the gubernaculum, normally begins its descent into the pelvis at approximately the 20th week of gestation.3 The ovary reaches its final destination at term. Hence, failure of the ovary to descend is usually associated with failure of the fallopian tube to attach to the uterus and lack of appropriate development of the distal, fimbriated end adjacent to the ovary.4 The maldescended ovary is in a high position and associated with a short infundibulopelvic ligament and meso-ovarium and an elongated utero-ovarian ligament.5
Ectopic ovary is commonly associated with other malformations of the genital tract and urinary tract.6 In literature reports of the patients who were discovered to have ectopic ovaries, unilateral ectopic ovary was the most common finding.4 Cases of bilateral undescended ovaries have been reported, albeit much less commonly.4 Regarding congenital Müllerian duct syndromes, unilateral ectopic ovary is most commonly associated with unicornuate uterus.7 Additionally, Rokitansky-Küster-Hauser syndrome, which involves congenital absence of the uterus, is sometimes associated with an ectopic ovary.4
The complications produced by an ectopic ovary are few, as it is generally asymptomatic. The most common symptoms are menstrual irregularities and abdominal pain.3 The true incidence of this entity is not known, as the reports in the literature evaluated a subset of patients who usually presented with infertility. Infertility is often cited as a consequence of ectopic ovary, although it has not been substantiated in cases of ectopic ovary in the absence of other congenital anomalies. Another known complication of ectopic ovary is an increased risk of ectopic pregnancies because of the positioning of the fallopian tube on the side of the ectopic ovary.4 As for neoplastic complications, transformations including mucinous cystadenomas and Brenner tumors have been reported infrequently, and a serous cysta denofibroma has been reported in a single case in the literature.8
CONCLUSION
Management of ectopic ovary has generally not been given much consideration in previous reports. Laparoscopic surgery and improved diagnostic imaging have afforded more options for the diagnosis and management of ectopic ovary. Conservative management is recommended, as the majority of cases of ectopic ovary are asymptomatic. MRI is an excellent noninvasive modality for initial diagnosis and follow-up in patients with infertility or recurrent pain. For patients with continual complaints or pathologic conditions such as neoplastic transformation or ovarian torsion, surgical removal is indicated.9 However, there is no literature citing an increased risk of pathology compared with that of a normally positioned ovary.
The importance of cross-sectional imaging-especially MRI-in the diagnosis and follow-up of ectopic ovary cannot be emphasized enough in this age when diagnostic imaging and noninvasive-imaging-guided therapeutic procedures are widely performed. MRI is the preferred modality because of its superior soft tissue contrast, multiplanar capability, lack of ionizing radiation, and noninvasiveness. Considering this entity in a young patient with chronic or unusual abdominal pain may be crucial and clinically significant.10
REFERENCES
- Wharton LR. Two cases of supernumerary ovary and one of accessory ovary, with an analysis of previously reported cases. Amer J Obstet Gynecol. 1959;78:1101-1119.
- Lachman MF, Berman MM. The ectopic ovary. A case report and review of the literature. Arch Pathol Lab Med. 1991;115:233-235.
- Watkins BP, Kothari SN. True ectopic ovary: A case and review. Arch Gynecol Obstet. 2004;269:145-146.
- Van Voorhis BJ, Dokras A, Syrop CH. Bilateral undescended ovaries: Association with inferility and treatment with IVF. Fertil Steril. 2000;74:1041-1043.
- Verkauf B, Bernhisel MA. Ovarian maldescent. Fertil Steril. 1996;65:189-192.
- Ombelet W, Grieten M, DeNeubourg P, et al. Undescended ovary and unicornuate uterus: Simplified diagnosis by the use of clomiphene citrate ovarian stimulation and magnetic resonance imaging (MRI). Hum Reprod. 2003;18:858-862. Erratum in: Hum Reprod. 2003;18:1362.
- Dabirashrafi H, Mohammad K. Moghadami-Tabrizi N. Ovarian malposition in women with uterine anomalies. Obstet Gynecol. 1994;83:293-294.
- Whitaker C, Tawfik O, Weed JC Jr, et al. Serous cystadenofibroma arising in an ectopic ovary. Kansas Med. 1997;98(2):24-26
- Peedicayil A, Sarada V, Jairaj P, Chandi SM. Ectopic ovary in the omentum. Asia Oceania J Obstet Gynaecol. 1992;18(1):7-11.
- Cohen JA, Holzman. A giant ectopic ovary. J Laparoendosc Adv Surg Tech A. 2001;11(1):31-35.
Products used
- Signa 1.0T MRI scanner, GE Healthcare, Waukesha, WI
- Omniscan, GE Healthcare, Princeton, NJ