Vueway™ (Gadopiclenol): Practical Considerations for Use
By Faulkner W
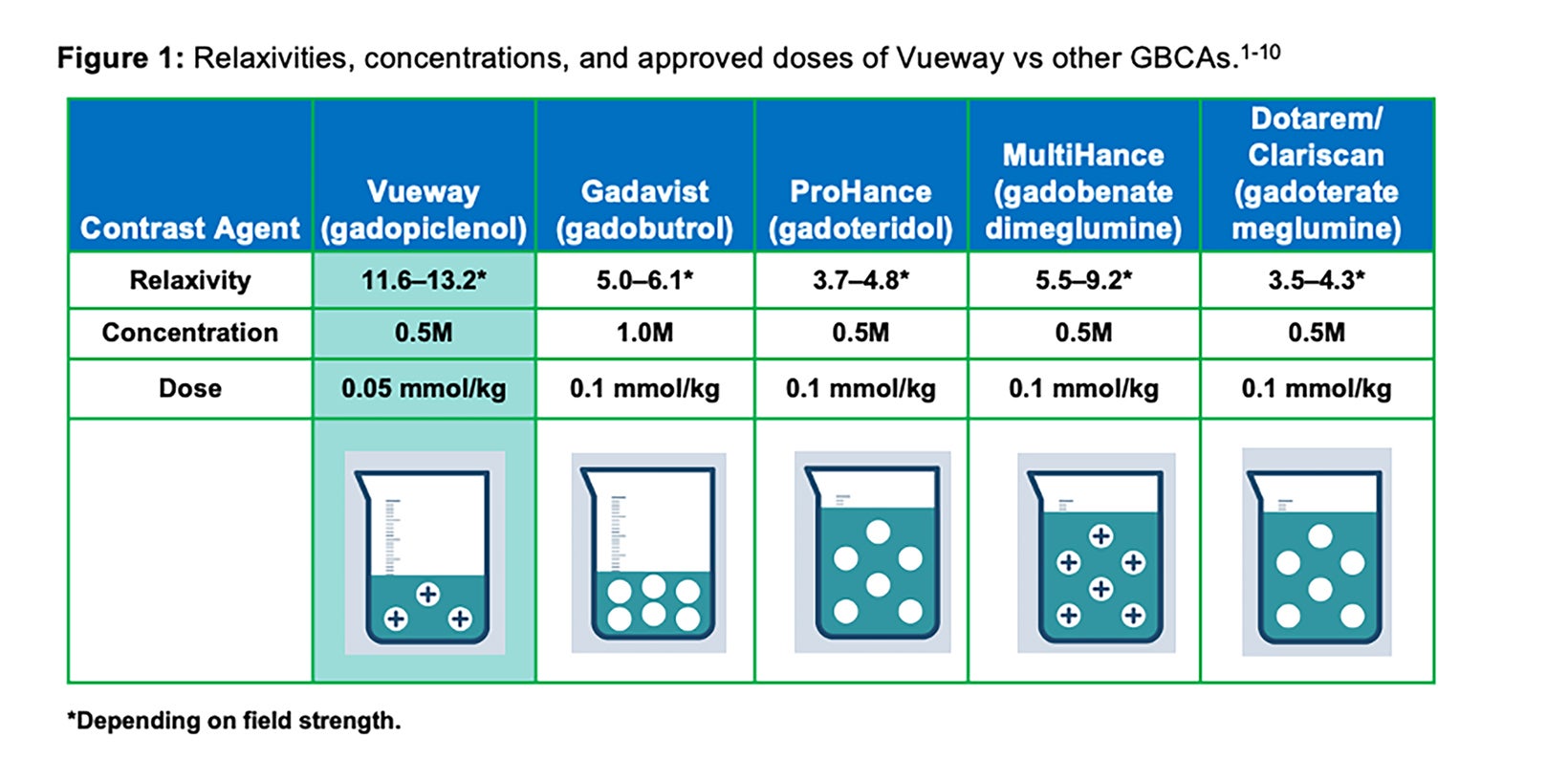
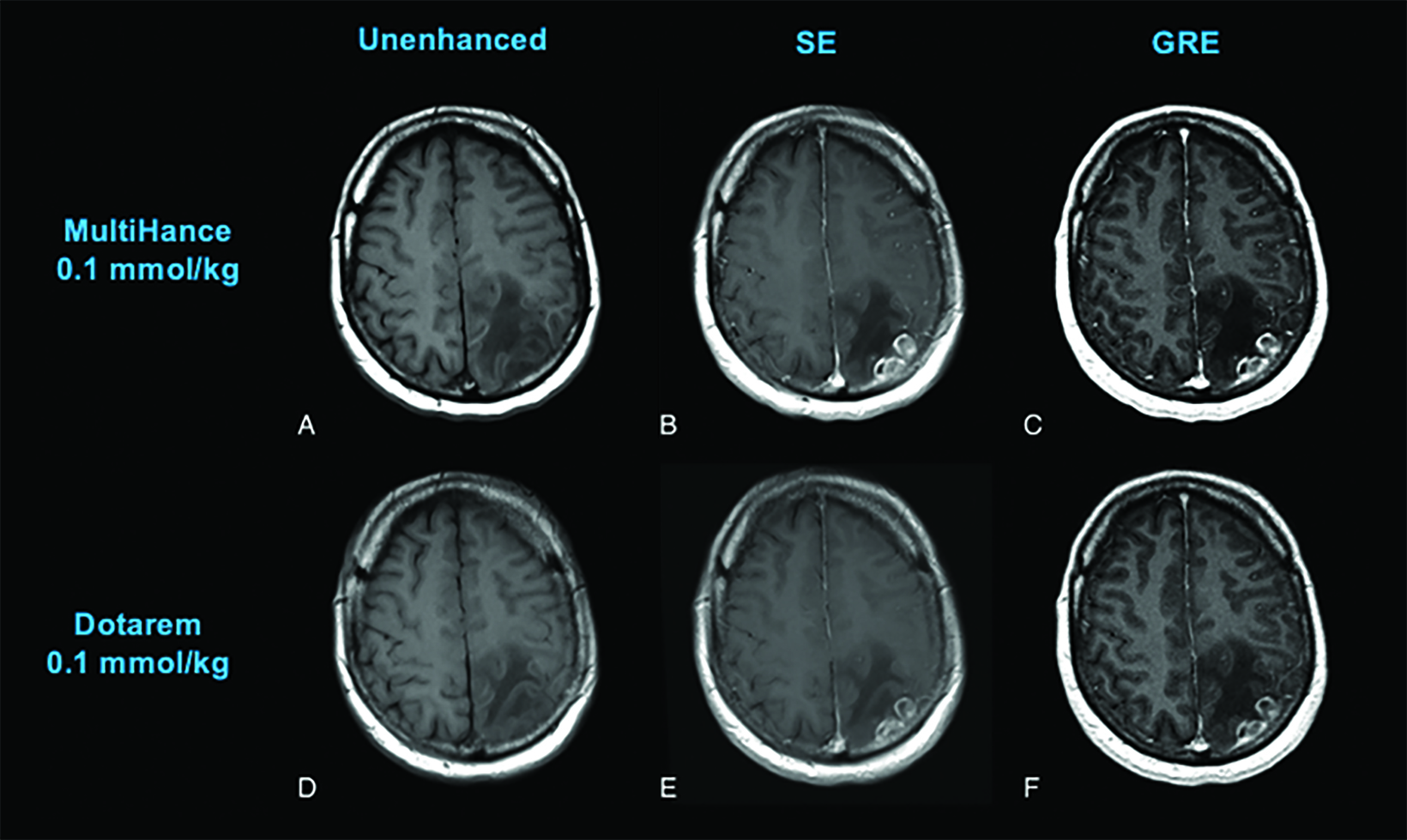
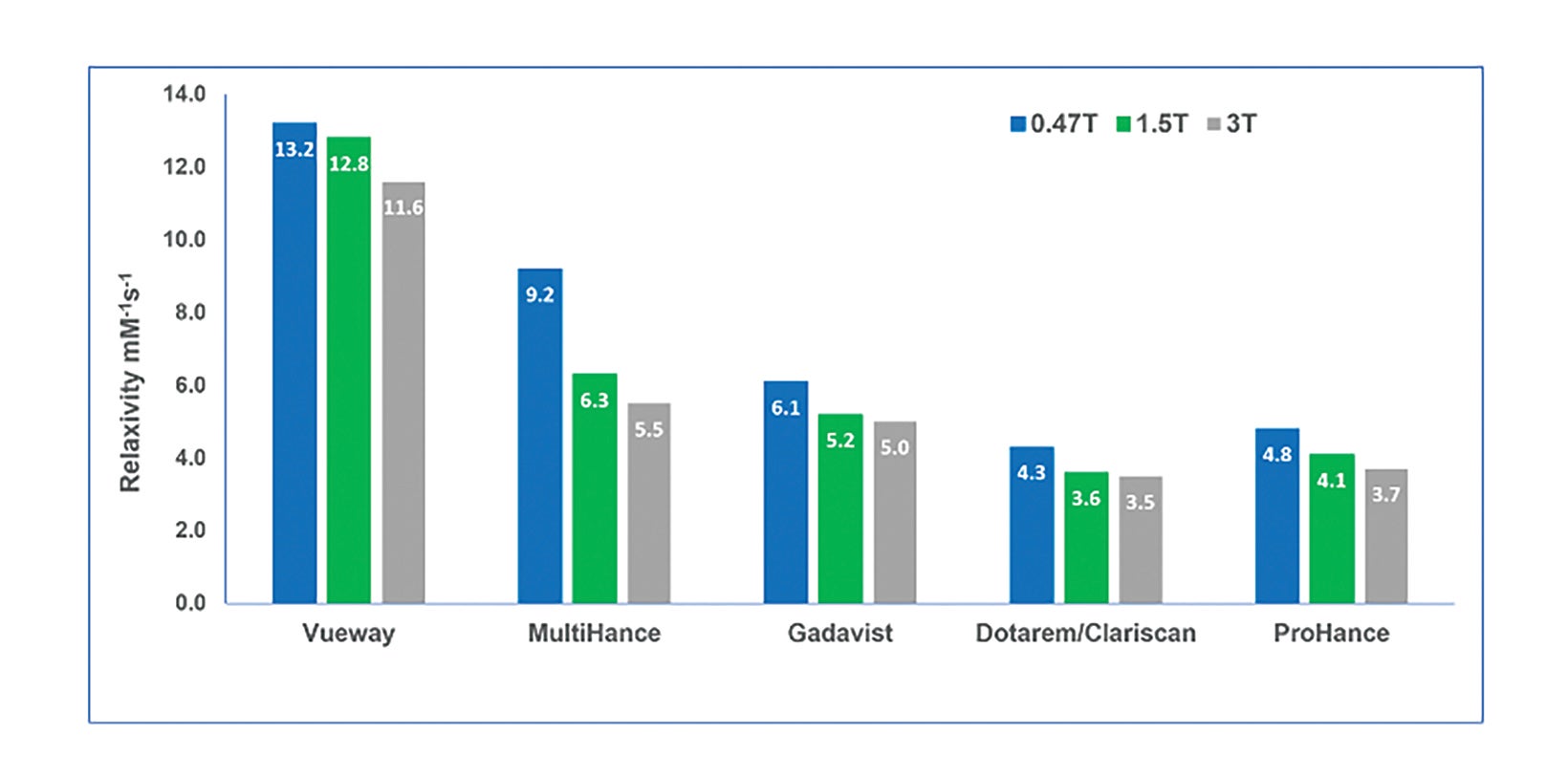
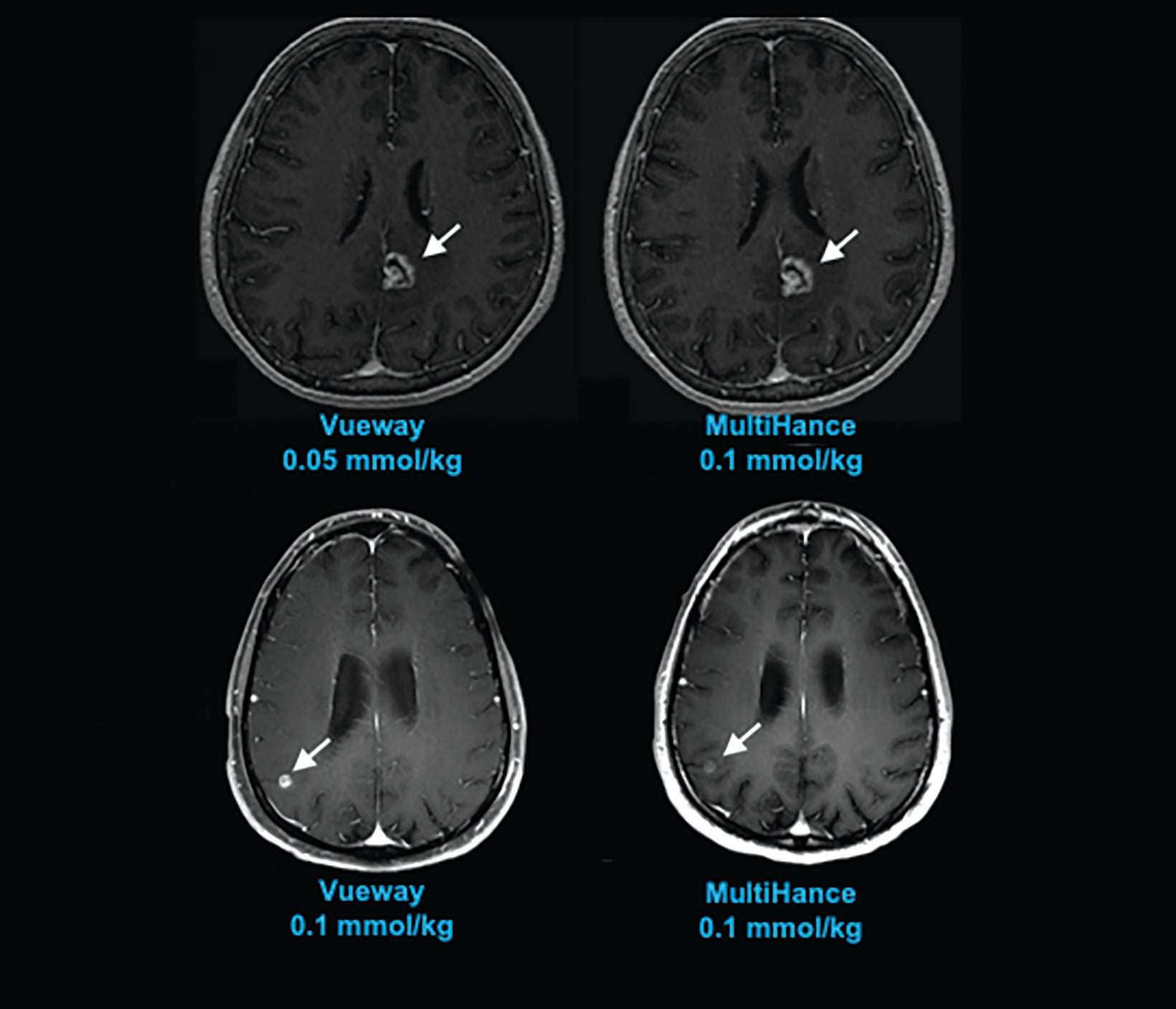
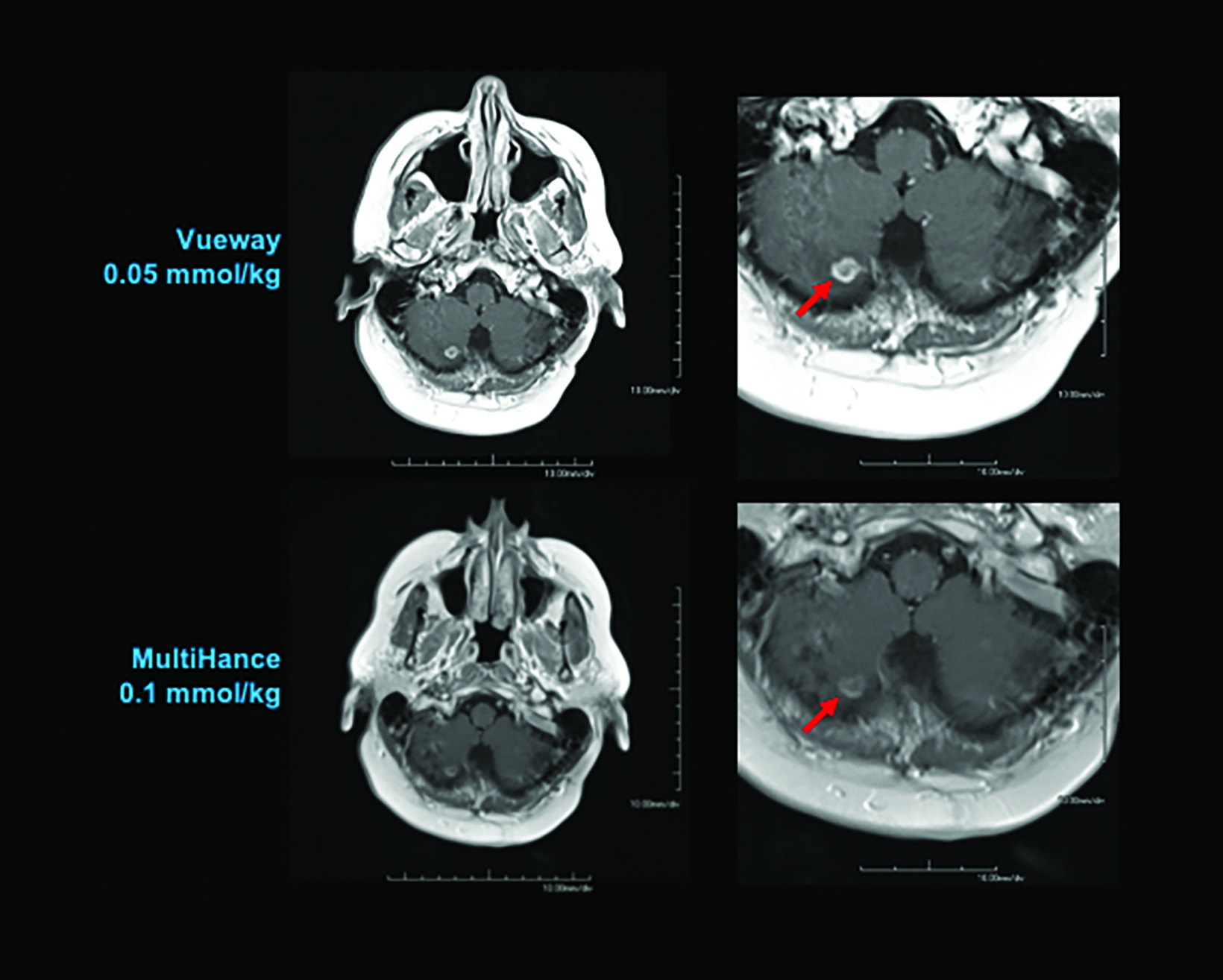
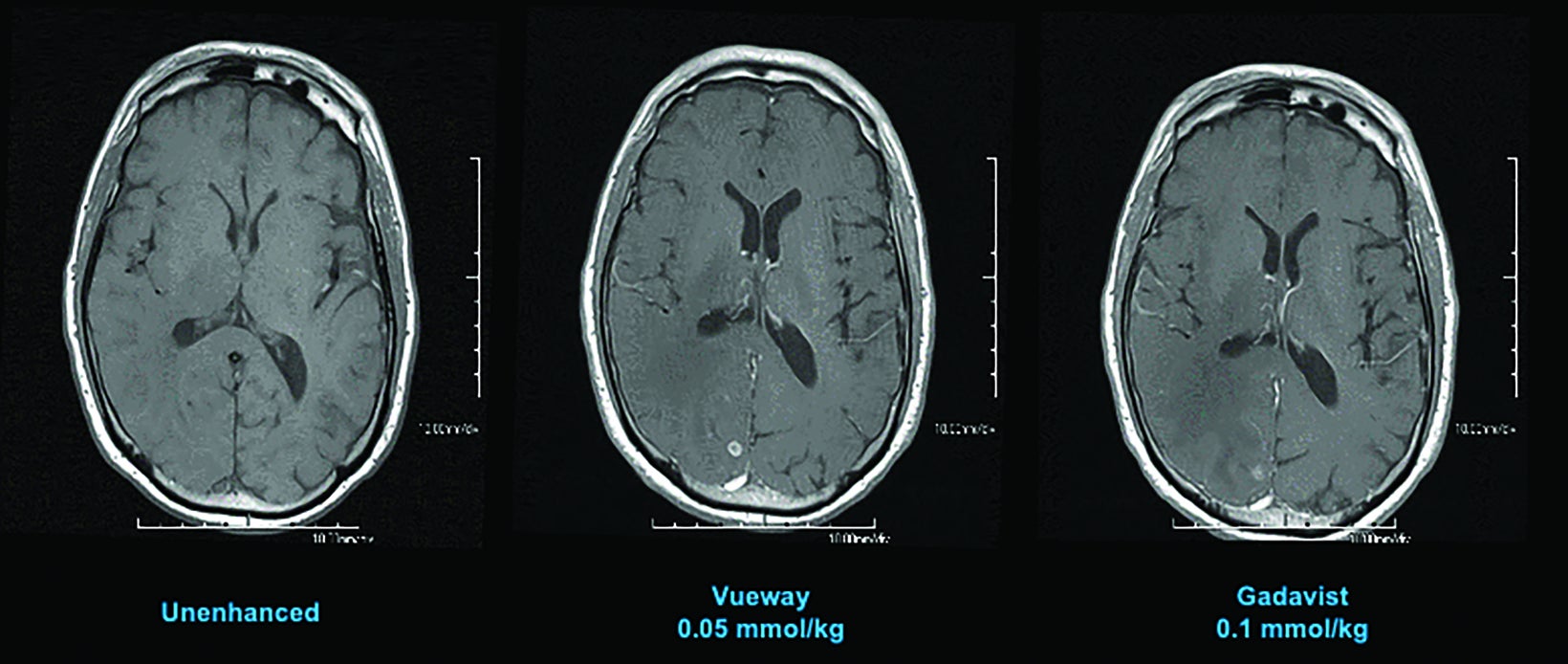
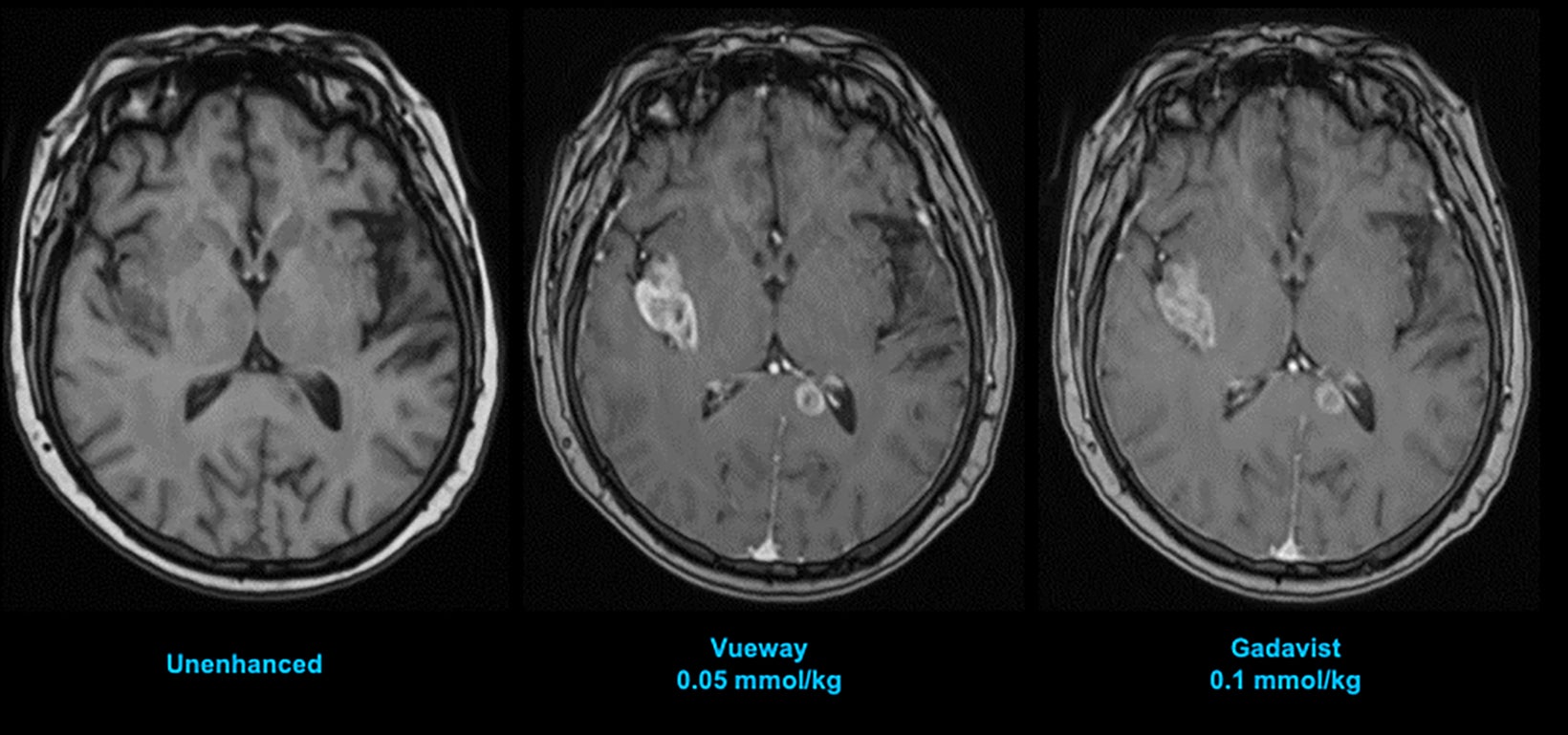
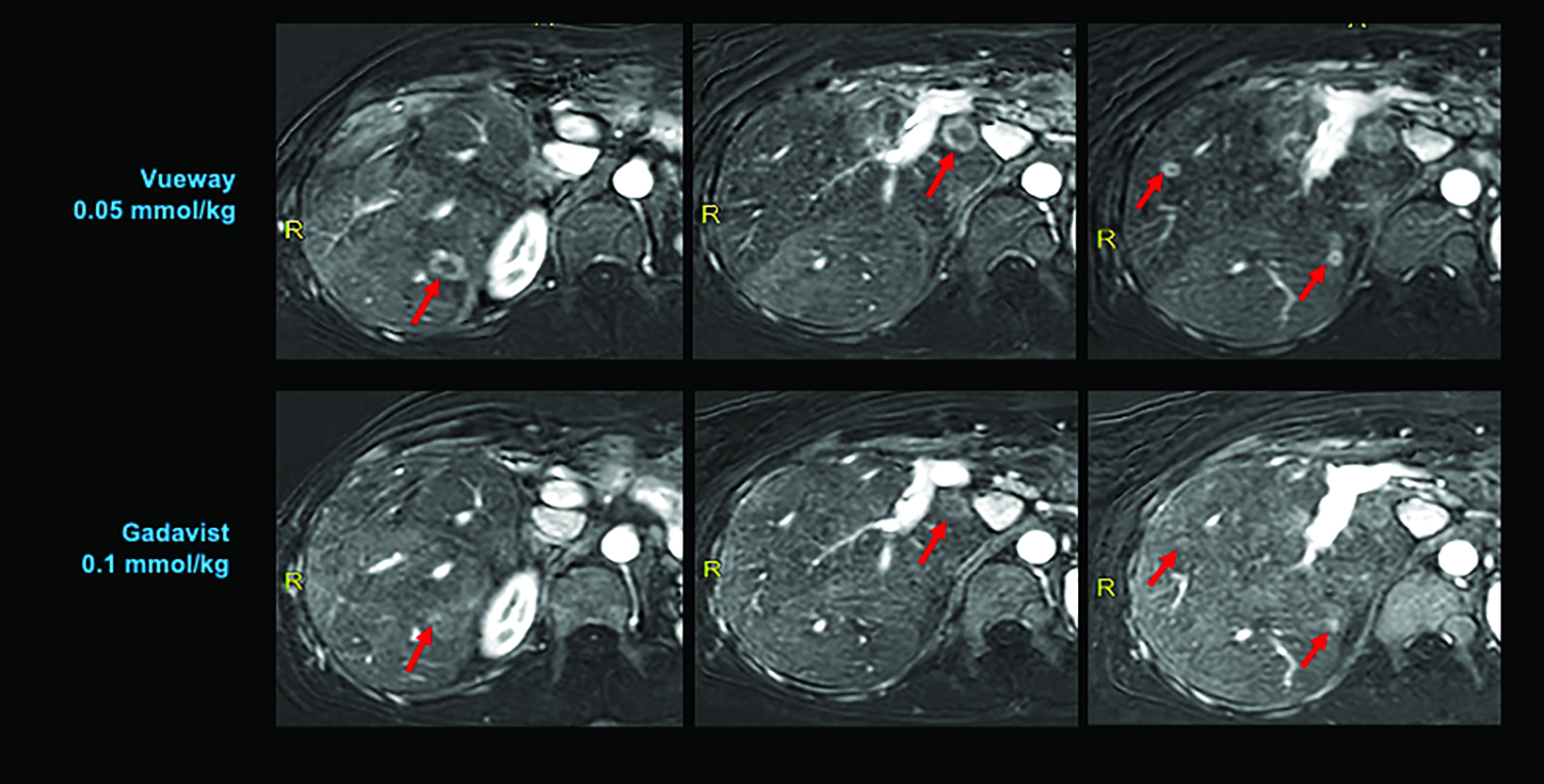
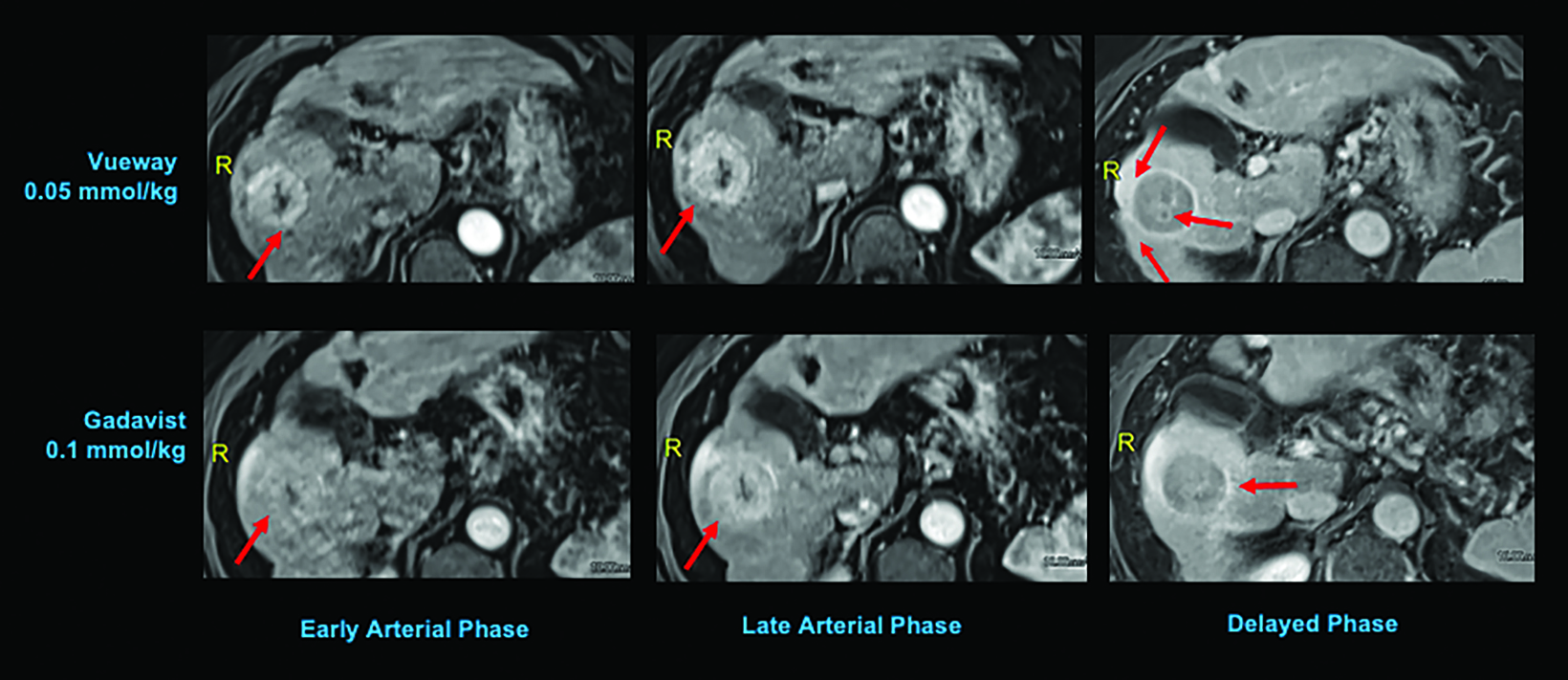
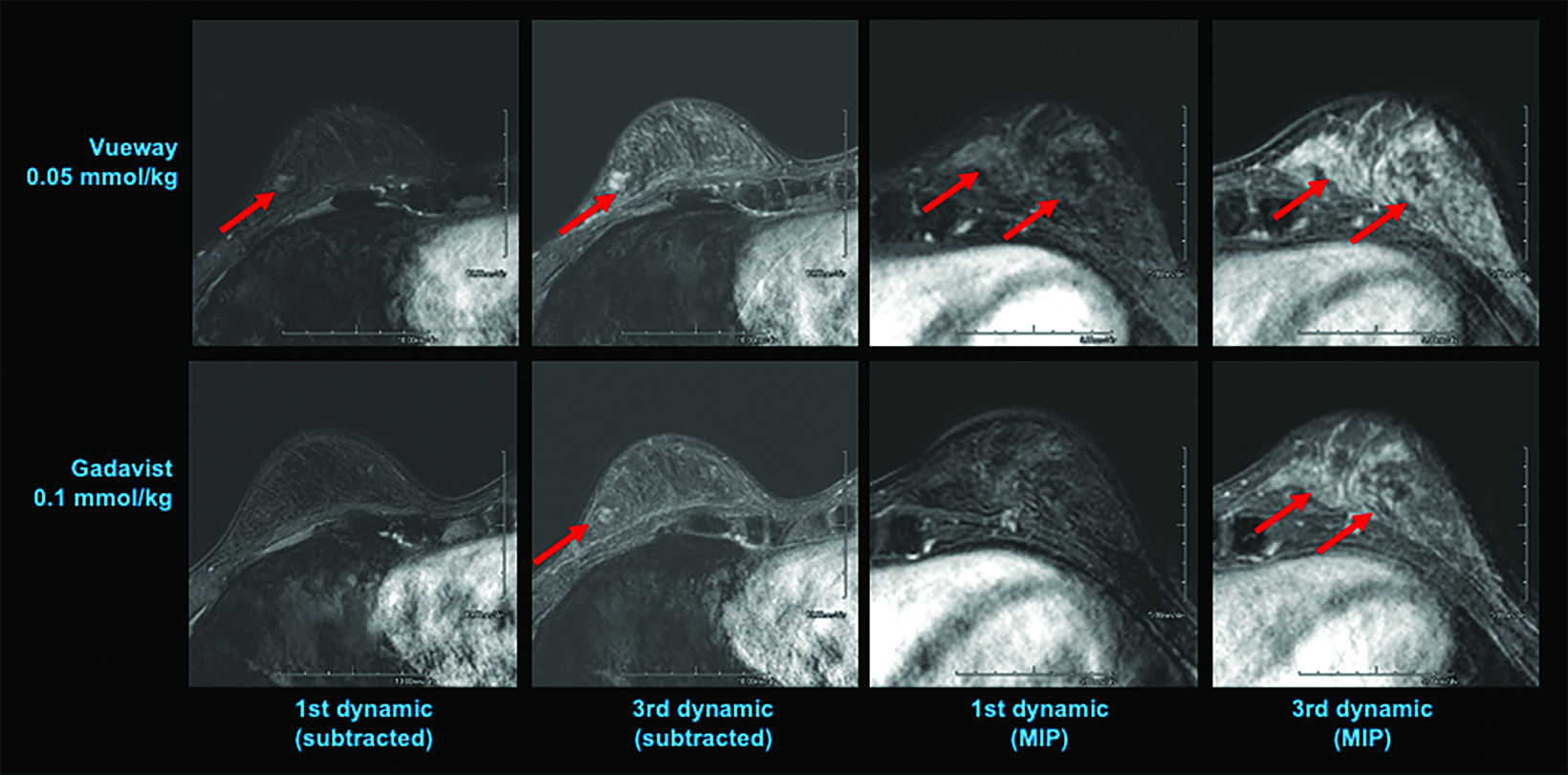
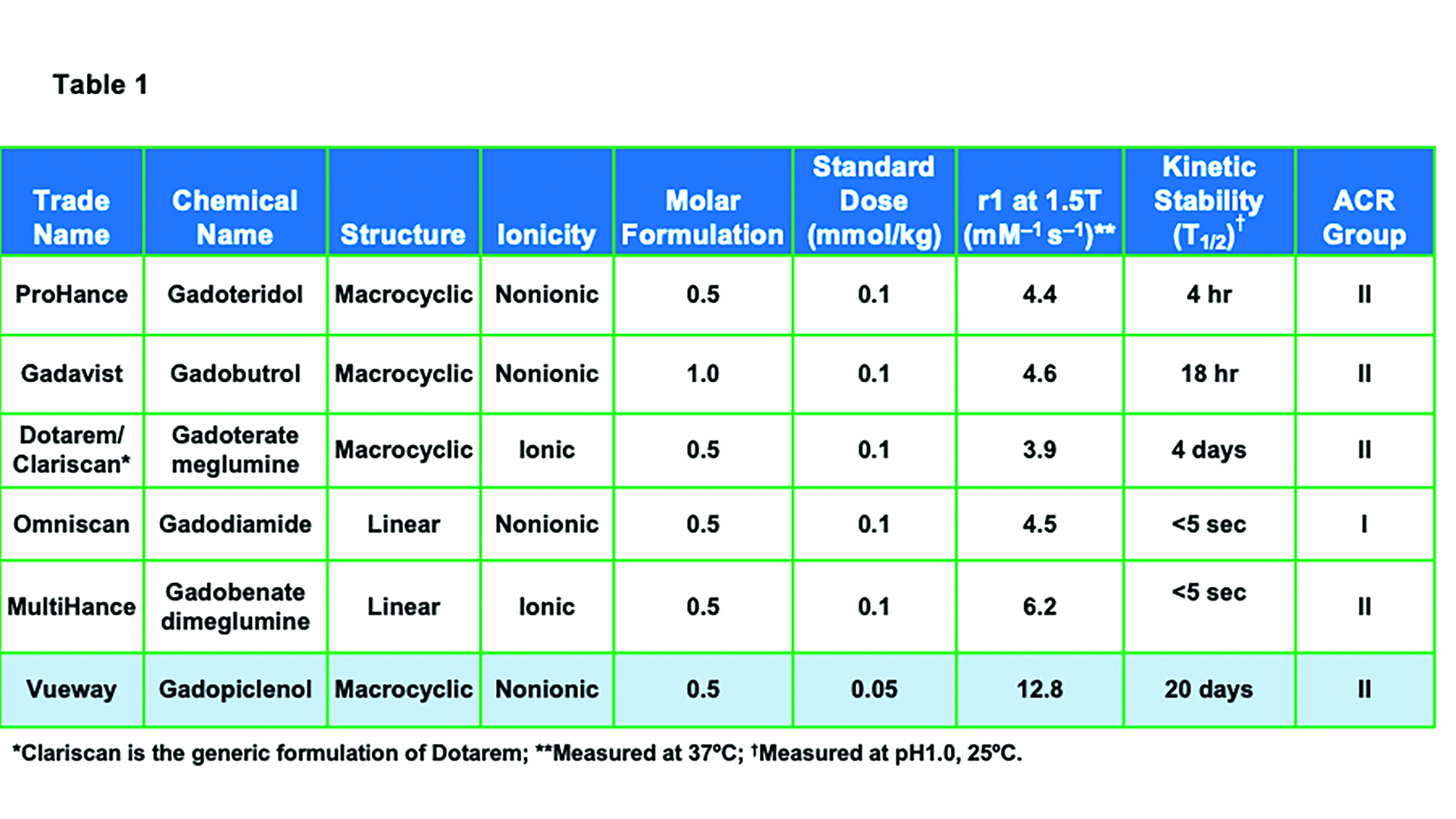
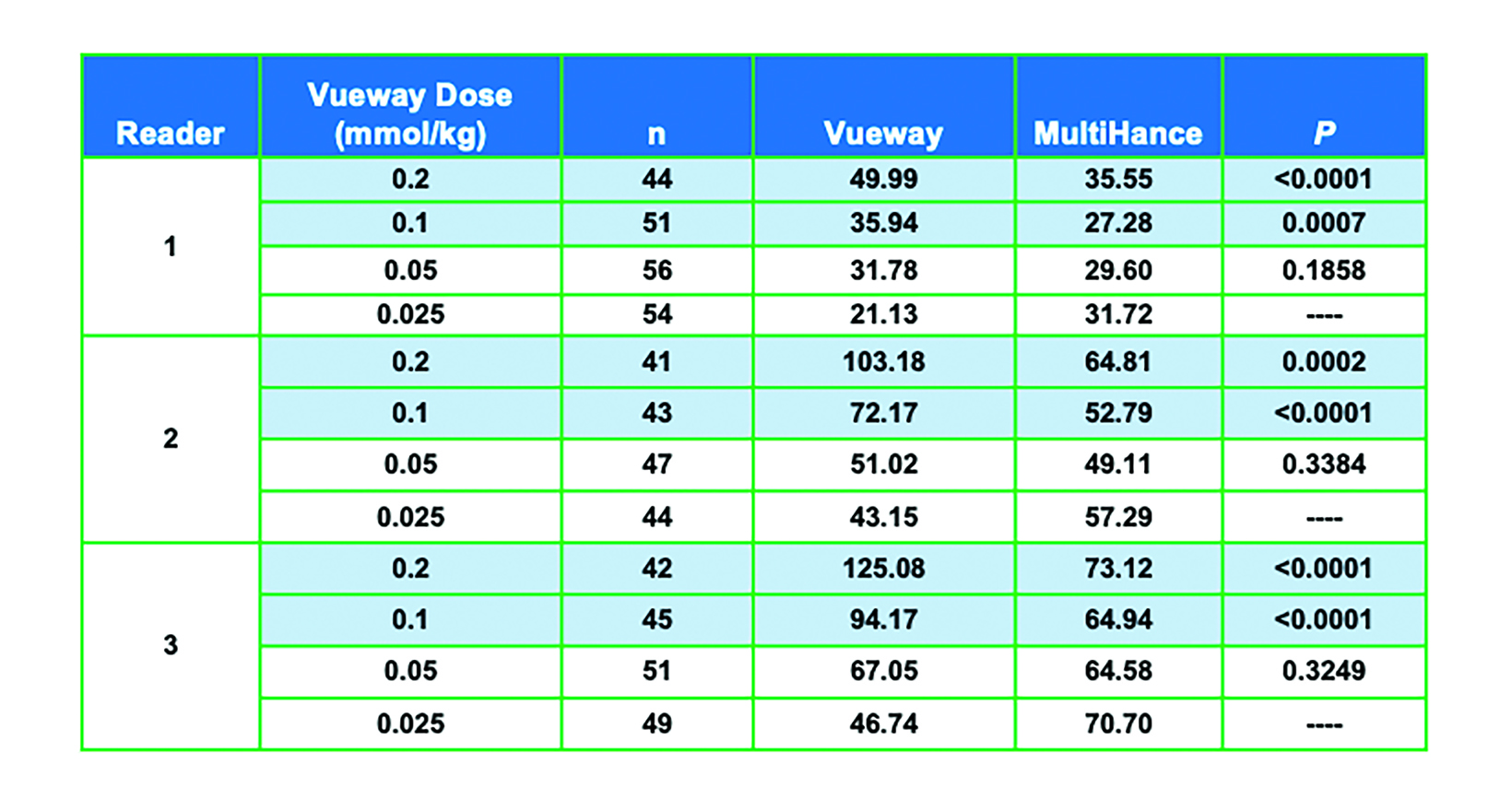
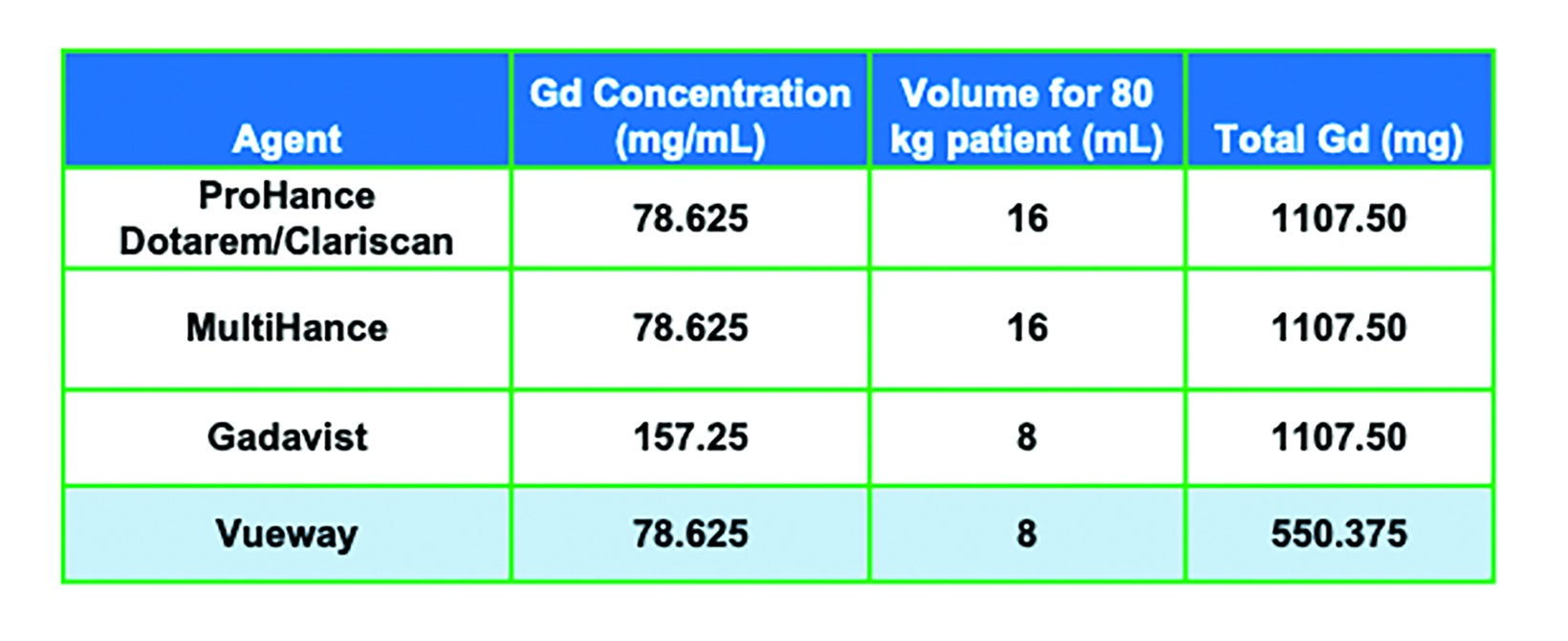
CE credits available here.
Introduction
During the past several decades, advances in hardware and software have resulted in significant improvements in magnetic resonance image (MRI) quality. The performance of gadolinium (Gd)-based contrast agents (GBCAs), meanwhile, has remained largely the same.
That changed first in 2004, with the approval of the high-relaxivity, linear agent MultiHance® (gadobenate dimeglumine), and then in 2023, when the high-relaxivity, macrocyclic agent Vueway™ (gadopiclenol) received US Food and Drug Administration (FDA) marketing approval. This paper reviews and compares the properties, mechanism-of-action, dosing, efficacy, and safety of Vueway with those of the six other GBCAs currently available in the US.
GBCA Properties, Mechanism-of-Action, Dosing, and Efficacy
Prior to the introduction of Vueway, six general-use, extracellular fluid GBCAs were available in the United States: ProHance® (gadoteridol), Gadavist® (gadobutrol), Dotarem® (gadoterate meglumine); Clariscan™ (gadoterate meglumine); Omniscan™ (gadodiamide); and MultiHance® (gadobenate dimeglumine).1-6 (Table 1) All of these agents utilize the Gd ion (64Gd) as the basis of enhancement.1-7 Gadolinium is a rare earth metal with strong paramagnetic properties due to the seven unpaired electrons in the orbital shell.11 The most common oxidized form has a +3 charge, which has a similar ionic radius as the divalent form of calcium, a critical mineral in the human body. Thus, Gd is a potent calcium antagonist in vivo; in order to be used safely as an MRI contrast agent, the Gd ion must be made physiologically inert.11-13 This is achieved by binding the Gd ion to a large organic molecule known as a ligand. The Gd ion plus the ligand are together referred to as a chelate. The chelate allows for safe elimination of the potentially toxic Gd ion from the body, primarily via the kidneys.11
As shown in Table 1, GBCAs vary in their chelate structure (ionic vs nonionic; macrocyclic vs linear) and molar concentration (the number of Gd molecules per mL).8,9 All are formulated at a concentration of 0.5M, with the exception of Gadavist, which is formulated at a concentration of 1M. (Figure 1) This difference in molar concentration is important in that, with respect to Gadavist vs the 0.5M agents, the same volume of each agent does not deliver the same dose of Gd. Indeed, the dose is determined not by the volume (mL) of the agent itself but by amount of Gd it delivers (in units of mmol/kg). Thus, administering an approved 0.1 mmol/kg dose of 1M Gadavist results in a lower volume of the agent being delivered, not a lower dose of Gd. This is depicted graphically in Figure 1: an approved dose of Gadavist delivers the same dose of Gd, just at half the volume. Note that Vueway is 0.5M, but due to its much higher relaxivity, an approved dose is half that of the other agents. This is discussed in more detail below.
Gadolinium-based contrast agents work by shortening the T1- and T2-relaxation times of water-based hydrogen protons.14 For the remainder of this article, only the T1-shortening effects will be addressed. When the large GBCA molecule comes within approximately 3 angstroms of a water molecule, the water molecule is incorporated into the inner sphere of the GBCA.15,16 The molecular tumbling rate of the water molecule slows, resulting in a significant shortening of the T1-relaxation time of the water-based hydrogen proton.
The relaxivity (r1) of a GBCA is a measurable characteristic of all GBCAs, reflecting the amount of T1-shortening of water-based protons for a given dose. The altered T1-relaxation time is inversely related to the relaxivity (r1) of the GBCA. The higher the relaxivity (r1), the shorter the T1-relaxation time of the water-based hydrogen protons. Higher relaxivity results in a greater signal-to-background ratio (i.e. higher contrast) when acquiring images with appropriate T1-weighting.15,16
Given that conventional GBCAs interact with a single water molecule, increasing the number of Gd molecules will involve more water molecules, thereby increasing the effect.15-18 This has been clearly demonstrated in ProHance dosing studies (up to 0.3 mmol/ kg).19 Note that as of this writing, ProHance is the only agent for which such triple dosing (0.3 mmol/kg) is included in the label.1
Until last year, the conventional relaxivity GBCAs were those with relaxivities (r1 at 1.5T) ranging from a low of 3.9 mM−1s−1, for Dotarem/Clariscan, up to 4.6 mM−1s−1 for Gadavist and 6.2 mM−1s−1 for the high-relaxivity agent MultiHance.1-10 (Table 1) The higher relaxivity of MultiHance results from its unique molecular structure, which includes an additional side chain that allows for weak and transient protein interaction, further increasing the effective size of the molecule. This results in a slower tumbling rate, greater T1 shortening, and almost twice the relaxivity of other conventional-relaxivity agents.20 (Table 1) As expected, when administered at the approved dose of 0.1 mmol/kg, MultiHance has been demonstrated in many intraindividual crossover studies (in which the same patient receives two MRI exams within several days, one with each GBCA) to result in increased lesion sensitivity and conspicuity.21-26 (Figure 2)
MultiHance can also be used to reduce the Gd dose, as a lower dose of MultiHance has demonstrated efficacy equivalent to the higher dose of a conventional-relaxivity GBCA.27-30 Note that Gadavist is marketed as a high-relaxivity agent, but it has no protein interaction, and its relaxivity is only slightly higher than the other nonprotein-interacting agents. (Table 1) Moreover, in intraindividual crossover studies, the efficacy of Gadavist has been shown to be comparable to conventional-relaxivity agents, but lower than the high-relaxivity GBCA MultiHance.26,32
GBCA Stability and Safety
Owing to differences in the molecular structure of GBCAs, the propensity of the ligand to release the Gd ion—ie, the agent’s stability varies. There are two major stability metrics, both measured in the laboratory (i.e., in vitro): the thermodynamic stability constant and the kinetic stability constant.33 Thermodynamic stability reflects the energy required to release the Gd ion and, when measured at a biological pH (pH=7.4), is referred to as the conditional thermodynamic stability constant. Kinetic stability reflects the rate or speed at which a given agent begins to disassociate the Gd ion from the ligand. Because the disassociation half-life for many agents is very long—hundreds of years — the kinetic stability of these agents is typically measured under chemically stressful conditions (pH=1). Of these two metrics, kinetic stability is considered to be the most significant in vivo.33 Based on these in vitro stability constants, the ionic, macrocyclic GBCAs have the highest stability, and the nonionic, linear GBCAs have the lowest stability, with the remainder having intermediate stability. (Table 1) Note that the key metric, the kinetic stability constant, is highest with the macrocyclic agents, regardless of ionicity.
In vivo assessments of stability focus on an organometallic reaction known as transmetallation. This is where a competitor, typically a metal found normally in the body (eg, iron, magnesium, copper, zinc, or calcium) displaces the Gd in the chelate and binds to the ligand, resulting in the release of free Gd.17,34 As expected, transmetallation has been shown to occur more readily with the less-stable GBCAs.17,34
GBCAs have long been considered extremely safe; however, concerns about their use began coming to light in 2006, particularly in vulnerable patient populations. Specifically, two related phenomena have been observed in patients administered lower-stability GBCAs. First, nephrogenic systemic fibrosis (NSF), a progressive and potentially fatal connective tissue disease, has been observed almost exclusively in patients with poor renal function who are administered repeat and/or higher doses of the least-stable GBCAs Omniscan, OptiMARK, and Magnevist (OptiMARK and Magnevist are no longer available in the US).10,35,36 Based on NSF risk, the American College of Radiology (ACR) has classified the available GBCAs into two groups; those with the highest risk for causing NSF (Group I: Omniscan, Magnevist, OptiMARK) and those with the lowest risk (Group II: MultiHance, Gadavist, Dotarem/Clariscan, ProHance, Vueway/Elucirem). In addition, the FDA has contraindicated the highest risk GBCAs in at-risk patients; ie, those with acute or chronic severe kidney dysfunction.10,37
Although the pathophysiology of NSF is not well understood, it is believed that GBCAs are not readily eliminated by patients with poor renal function. This, combined with the lower stability of the GBCA, is believed to initiate a fibrotic cascade in tissues.38 Nephrogenic systemic fibrosis has largely been eliminated, thanks to changes in patient management, including screening at-risk patients and limiting use of the less-stable GBCAs.39 Many practices have shifted away from linear agents in favor of macrocyclic agents, particularly in patients with poor renal function, in those requiring repeat scans, and in children,40 despite the fact that the linear agent MultiHance >belongs to Group II.
Further fueling the movement toward macrocyclic agents was the discovery that small amounts of Gd are retained in certain areas of the brain and body. While it has been known for decades that Gd can be found in bone and other organs following GBCA administration,34,41,42 retention in the brain of patients without blood-brain barrier disruption was completely unexpected.43 Sensitive assays have detected Gd in the brain after administration of all GBCAs;44 however, similar to that seen with NSF, the less-stable agents were associated with higher levels of Gd retention.43,45-47 Notably, there is no evidence to date of clinically significant sequelae related to Gd retention.
Nevertheless, the ACR and the FDA have issued warnings that GBCAs should be administered only when deemed necessary and only at the lowest dose necessary for diagnosis.10,48
Given all that is known regarding the characteristics and safety of GBCAs, the need for a macrocyclic, high-relaxivity GBCA that can limit patient exposure to Gd without compromising image quality has become increasingly apparent.
Vueway
Vueway is a macrocyclic GBCA (like ProHance, Gadavist, and Dotarem/Clariscan) with high kinetic stability (higher than ProHance, Gadavist, and Dotarem/Clariscan).8 (Table 1) Owing to the agent’s high stability, the latest version of the ACR Contrast Media Manual has classified Vueway as a Group II agent, the safest class of GBCAs.10 In addition, it has very high relaxivity (over twice that of the high-relaxivity GBCA MultiHance), and this high relaxivity persists at higher field strengths.8 (Table 1; Figure 3) With respect to Gd retention in the brain, a study in rats demonstrated that, like other macrocyclic GBCAs, only a very small amount of retention was attributable to Vueway.49
There are several bases for the higher relaxivity seen with Vueway. First, it has a q value of 2, which means it incorporates two water molecules within its inner hydration sphere, as opposed to the other agents, which allow only one water molecule within their inner hydration sphere.8,18 Second, the Vueway molecule has three hydrophilic arms, allowing for interaction with more water molecules in its outer sphere.8 Finally, the larger molecule size of Vueway results in an even slower molecular tumbling rate.8,18
Note that the recommended dosing for Vueway included in the labeling could have been equivalent to the approved dose of the other GBCAs (0.1 mmol/kg), with the expectation that contrast enhancement would be superior. However, due to concerns about Gd exposure in patients, the recommended dosing is actually half (0.05 mmol/kg) the recommended dose of the other extracellular agents; therefore, the recommended volume delivered to achieve approved dosing is comparable to the 1M agent Gadavist.7 (Table 3)
One would expect from the higher relaxivity of Vueway that it would perform better than MultiHance or conventional GBCAs at a comparable dose and perform comparably at a lower dose. In an adult phase 2 dosing study, 272 subjects with known or suspected brain lesions were randomized to receive one of four body-weight doses of Vueway—0.2 mmol/kg; 0.1 mmol/kg; 0.05 mmol/kg; or 0.025 mmol/kg—vs a single 0.1 mmol/kg dose of MultiHance.50
The results from three blinded readers are shown in Table 2. When comparing 0.1 mmol/kg MultiHance to the lowest dose of Vueway (0.025 mmol/kg), all three readers rated the contrast enhancement greater with MultiHance; however, images acquired using 0.05, 0.1, or 0.2 mmol/kg Vueway all showed greater enhancement than those acquired using MultiHance, with 0.1 and 0.2 mmol/kg doses demonstrating significantly better enhancement.50 Examples of paired images (0.1 mmol/kg MultiHance vs 0.05 or 0.1 mmol/kg Vueway) are shown in Figures 4 and 5.50
Phase III intraindividual clinical trials comparing Gd retention in the brain and other parts of the body with Vueway vs. Gadavist have also been performed. Specifically, the safety and effectiveness of Vueway for lesion visualization was evaluated in two prospective, double-blind, randomized, crossover clinical studies.7,51,52 Study 1 (NCT03996447; the PICTURE Trial) was performed in 256 adults with known or highly suspected CNS lesions with focal areas of disruption of the blood-brain barrier.51 Study 2 (NCT03986138; the PROMISE Trial) was performed in 273 adults with suspected enhancing abnormalities in at least one body region among the head and neck, thorax, abdomen, pelvis, and musculoskeletal system.52 In each study, patients received both 0.05 mmol/kg Vueway and 0.1 mmol/kg Gadavist in randomized order separated by 2 days to 14 days.7,51,52 Magnetic resonance imaging was performed before and after administration of each contrast agent. Precontrast and paired (consisting of both precontrast and postcontrast images for the same drug) image sets were independently evaluated by three central readers who were blinded to the identity of the contrast agent.7,51,52 Readers scored up to three lesions per patient for border delineation, internal morphology, and contrast enhancement, each on a scale from 1 to 4.7,51,52 In all cases, the approved 0.05 mmol/kg dose of Vueway performed better at the quantitative level than the approved 0.1 mmol/kg dose of the conventional-relaxivity comparator Gadavist.7,51,52 Analysis of visualization endpoints led to non-inferiority.7,51,52 Examples of paired images from these brain and body studies are shown in Figures 6-10.51,52 Moreover, in both the PICTURE and the PROMISE trials, the safety profile of Vueway was similar to that of Gadavist, with rare and mostly mild side effects.51,52
Conclusion
With its macrocyclic structure, high relaxivity and stability, and lower approved dose, Vueway is the ideal contrast agent for MRI. The risk of NSF and Gd retention is low, and the lower approved dosing reduces patient exposure to Gd without sacrificing image quality or lesion conspicuity.
References
- ProHance® (Gadoteridol) Injection, 279.3 mg/mL Full Prescribing Information and Patient Medication Guide. Monroe Twp., NJ: Bracco Diagnostics Inc.; January 2024.
- Gadavist® (gadobutrol) Injection. Full Prescribing Information. Bayer HealthCare Pharmaceuticals Inc. Whippany, NJ; April 2022.
- Dotarem® (gadoterate meglumine) Injection. Full Prescribing Information. Guerbet LLC. Princeton, NJ; April 2022.
- Clariscan™ (gadoterate meglumine) injection for intravenous use. Full Prescribing Information. GE Healthcare. Chicago, IL; February 2020.
- Omniscan™ (gadodiamide) injection [prescribing information]. Princeton, NJ: GE Healthcare; August 2013.
- MultiHance® (gadobenate dimeglumine) injection, 529 mg/mL Full Prescribing Information and Patient Medication Guide. Monroe Twp., NJ: Bracco Diagnostics Inc.; January 2024.
- Vueway® (gadopiclenol) solution for injection, 485.1 mg/mL Full Prescribing Information and Patient Medication Guide. Monroe Twp., NJ: Bracco Diagnostics Inc.; January 2024.
- Robic C, Port M, Rousseaux O, et al. Physicochemical and pharmacokinetic profiles of gadopiclenol: a new macrocyclic gadolinium chelate with high T1 relaxivity. Invest Radiol. 2019;54:475-484.
- Shen Y, Goerner FL, Snyder C, et al. T1 relaxivities of gadolinium-based magnetic resonance contrast agents in human whole blood at 1.5, 3, and 7 T. Invest Radiol. 2015;50:330-338.
- American College of Radiology (ACR) Committee on Drugs and Contrast Media. ACR Manual on Contrast Media, 2023. Available at: https://www.acr.org/Clinical-Resources/Contrast-Manual. Accessed February 14, 2024.
- Blomqvist L, Nordberg GF, Nurchi VM, Aaseth JO. Gadolinium in medical imaging—Usefulness, toxic reactions and possible countermeasures—A review. Biomolecules. 2022;12:742.
- Bourne GW, Trifaró JM. The gadolinium ion: a potent blocker of calcium channels and catecholamine release from cultured chromaffin cells. Neuroscience. 1982;7:1615-1622.
- Rogosnitzky M, Branch S. Gadolinium-based contrast agent toxicity: a review of known and proposed mechanisms. Biometals. 2016;29:365-376.
- Wahsner J, Gale EM, Rodríguez-Rodríguez A, Caravan P. Chemistry of MRI contrast agents: Current challenges and new frontiers. Chem Rev. 2019;119:957-1057.
- Dumas S, Jacques V, Sun WC, et al. High relaxivity magnetic resonance imaging contrast agents. Part 1. Impact of single donor atom substitution on relaxivity of serum albumin-bound gadolinium complexes. Invest Radiol. 2010;45:600-612.
- Jacques V, Dumas S, Sun WC, Troughton JS, Greenfield MT, Caravan P. High-relaxivity magnetic resonance imaging contrast agents. Part 2. Optimization of inner- and second-sphere relaxivity. Invest Radiol. 2010;45:613-624.
- Clough TJ, Jiang L, Wong KL, Long NJ. Ligand design strategies to increase stability of gadolinium-based magnetic resonance imaging contrast agents. Nat Commun. 2019;10:1420.
- Bendszus M, Laghi A, Munuera J, Tanenbaum LN, Taouli B, Thoeny HC. MRI Gadolinium-based contrast media: Meeting radiological, clinical, and environmental needs. J Magn Reson Imaging. 2024 Jan 16.
- Runge VM, Wells JW, Nelson KL, Linville PM. MR imaging detection of cerebral metastases with a single injection of high-dose gadoteridol. J Magn Reson Imaging. 1994;4:669-673.
- Spinazzi A, Lorusso V, Pirovano G, et al. Safety, tolerance, biodistribution, and MR imaging enhancement of the liver with gadobenate dimeglumine: results of clinical pharmacologic and pilot imaging studies in nonpatient and patient volunteers. Acad Radiol. 1999;6:282–291.
- Maravilla KR, Maldjian JA, Schmalfuss IM, et al. Contrast enhancement of central nervous system lesions: multicenter intraindividual crossover comparative study of two MR contrast agents. Radiology. 2006;240:389-400.
- Rowley HA, Scialfa G, Gao PY, et al. Contrast-enhanced MR imaging of brain lesions: a large scale intraindividual crossover comparison of gadobenate dimeglumine versus gadodiamide. AJNR Am J Neuroradiol. 2008;29:1684-1691.
- Rumboldt Z, Rowley HA, Steinberg F, et al. Multicenter, double-blind, randomized, intraindividual crossover comparison of gadobenate dimeglumine and gadopentetate dimeglumine in MRI of brain tumors at 3 Tesla. J Magn Reson Imaging. 2009;29:760-767.
- Vaneckova M, Herman M, Smith MP, et al. The benefits of high relaxivity for brain tumor imaging: results of a multicenter intraindividual crossover comparison of gadobenate dimeglumine with gadoterate meglumine (the BENEFIT study). AJNR Am J Neuroradiol. 2015;36:1589-1598.
- Martincich L, Faivre-Pierret M, Zechmann CM, et al. Multicenter, double-blind, randomized, intraindividual crossover comparison of gadobenate dimeglumine and gadopentetate dimeglumine for Breast MR imaging (DETECT Trial). Radiology. 2011;258:396-408.
- Seidl Z, Vymazal J, Mechl M, et al. Does higher gadolinium concentration play a role in the morphologic assessment of brain tumors? Results of a multicenter intraindividual crossover comparison of gadobutrol versus gadobenate dimeglumine (the MERIT Study). AJNR Am J Neuroradiol. 2012;33:1050-1058.
- Schneider G, Maas R, Schultze Kool L, et al. Low-dose gadobenate dimeglumine versus standard dose gadopentetate dimeglumine for contrast-enhanced magnetic resonance imaging of the liver: an intra-individual crossover comparison. Invest Radiol. 2003;38:85-94.
- Cheong BY, Duran C, Preventza OA, Muthupillai R. Comparison of low-dose higher-relaxivity and standard-dose lower-relaxivity contrast media for delayed-enhancement MRI: a blinded randomized crossover study. AJR Am J Roentgenol. 2015;205:533-539.
- Wang J, Yan F, Liu J, et al. Multicenter, intra-individual comparison of single dose gadobenate dimeglumine and double dose gadopentetate dimeglumine for MR angiography of the peripheral arteries (the Peripheral VALUE Study). J Magn Reson Imaging. 2013;38:926-937.
- Li Y, Li X, Li D, et al. Multicenter, intraindividual comparison of single-dose gadobenate dimeglumine and double-dose gadopentetate dimeglumine for MR angiography of the supra-aortic arteries (the Supra-Aortic VALUE study). AJNR Am J Neuroradiol. 2013;34:847-854.
- DeLano MC, Spampinato MV, Chang EY, et al. Dose-lowering in contrast-enhanced MRI of the central nervous system: aretrospective, parallel-group comparison using gadobenate dimeglumine. J Magn Reson Imaging. 2021;54:1660-1675.
- Maravilla KR, Smith MP, Vymazal J, et al. Are there differences between macrocyclic gadolinium contrast agents for brain tumor imaging? Results of a multicenter intraindividual crossover comparison of gadobutrol with gadoteridol (the TRUTH study). AJNR Am J Neuroradiol. 2015;36:14-23.
- Port M, Idée JM, Medina C, Robic C, Sabatou M, Corot C. Efficiency, thermodynamic and kinetic stability of marketed gadolinium chelates and their possible clinical consequences: a critical review. Biometals. 2008;21:469-490.
- Gibby WA, Gibby KA, Gibby WA. Comparison of Gd DTPA-BMA (Omniscan) versus Gd HP-DO3A (ProHance) retention in human bone tissue by inductively coupled plasma atomic emission spectroscopy. Invest Radiol. 2004;39:138-142.
- Sadowski EA, Bennett LK, Chan MR, et al. Nephrogenic systemic fibrosis: risk factors and incidence estimation. Radiology. 2007;243:148-157.
- Thomsen HS. NSF: still relevant. J Magn Reson Imaging. 2014;40:11-12.
- Food and Drug Administration Website. FDA Drug Safety Communication: New warnings for using gadolinium-based contrast agents in patients with kidney dysfunction. 02/06/2018. Available at: https://www.fda.gov/drugs/drug-safety-and-availability/fda-drug-safety-communication-new-warnings-using-gadolinium-based- contrast-agents-patients-kidney. Accessed February 14, 2024.
- Mathur M, Jones JR, Weinreb JC. Gadolinium deposition and nephrogenic systemic fibrosis: A radiologist’s primer. Radiographics. 2020;40:153-162.
- Wertman R, Altun E, Martin DR, et al. Risk of nephrogenic systemic fibrosis: evaluation of gadolinium chelate contrast agents at four American universities. Radiology. 2008;248:799-806.
- Mithal LB, Patel PS, Mithal D, Palac HL, Rozenfeld MN. Use of gadolinium-based magnetic resonance imaging contrast agents and awareness of brain gadolinium deposition among pediatric providers in North America. Pediatr Radiol. 2017;47:657-664.
- Tweedle MF, Wedeking P, Kumar K. Biodistribution of radiolabeled, formulated gadopentetate, gadoteridol, gadoterate, and gadodiamide in mice and rats. Invest Radiol. 1995;30:372-380.
- White GW, Gibby WA, Tweedle MF. Comparison of Gd(DTPA-BMA) (Omniscan) versus Gd(HP-DO3A) (ProHance) relative to gadolinium retention in human bone tissue by inductively coupled plasma mass spectroscopy. Invest Radiol. 2006;41:272-278.
- McDonald RJ, Levine D, Weinreb J, Kanal E, Davenport MS, Ellis JH, Jacobs PM, Lenkinski RE, Maravilla KR, Prince MR, Rowley HA, Tweedle MF, Kressel HY. Gadolinium Retention: A Research Roadmap from the 2018 NIH/ACR/RSNA Workshop on Gadolinium Chelates. Radiology. 2018;289:517-534.
- Murata N, Murata K, Gonzalez-Cuyar LF, Maravilla KR. Gadolinium tissue deposition in brain and bone. Magn Reson Imaging. 2016;34:1359-1365.
- Robert P, Violas X, Grand S, et al. Linear gadolinium-based contrast agents are associated with brain gadolinium retention in healthy rats. Invest Radiol. 2016;51:73-82.
- Jost G, Frenzel T, Boyken J, Lohrke J, Nischwitz V, Pietsch H. Long-term excretion of gadolinium-based contrast agents: linear versus macrocyclic agents in an experimental rat model. Radiology. 2019;290:340-348.
- Kobayashi M, Levendovszky SR, Hippe DS, et al. Comparison of Human Tissue Gadolinium Retention and Elimination between Gadoteridol and Gadobenate. Radiology. 2021;300:559-569.
- Food and Drug Administration Website. FDA Drug Safety Communication: FDA identifies no harmful effects to date with brain retention of gadolinium-based contrast agents for MRIs; review to continue. 12/19/2017. Available at: https://www.fda.gov/drugs/drug-safety-and-availability/fda-drug-safety-communication-fda- identifies-no-harmful-effects-date-brain-retention-gadolinium. Accessed February 14, 2024.
- Funke SKI, Factor C, Rasschaert M, et al. Long-term gadolinium retention in the healthy rat brain: comparison between gadopiclenol, gadobutrol, and gadodiamide. Radiology. 2022;305:179-189.
- Bendszus M, Roberts D, Kolumban B, et al. Dose finding study of gadopiclenol, a new macrocyclic contrast agent, in MRI of central nervous system. Invest Radiol. 2020;55:129-137.
- Loevner LA, Kolumban B, Hutóczki G, et al. Efficacy and safety of gadopiclenol for contrast-enhanced MRI of the central nervous system: The PICTURE randomized clinical trial. Invest Radiol. 2023;58:307-313.
- Kuhl C, Csőszi T, Piskorski W, Miszalski T, Lee JM, Otto PM. Efficacy and safety of half-dose gadopiclenol versus full-dose gadobutrol for contrast-enhanced body MRI. Radiology. 2023;308:e222612.