The Use of Contrast-enhanced MRI in At-risk Patient Populations
Images
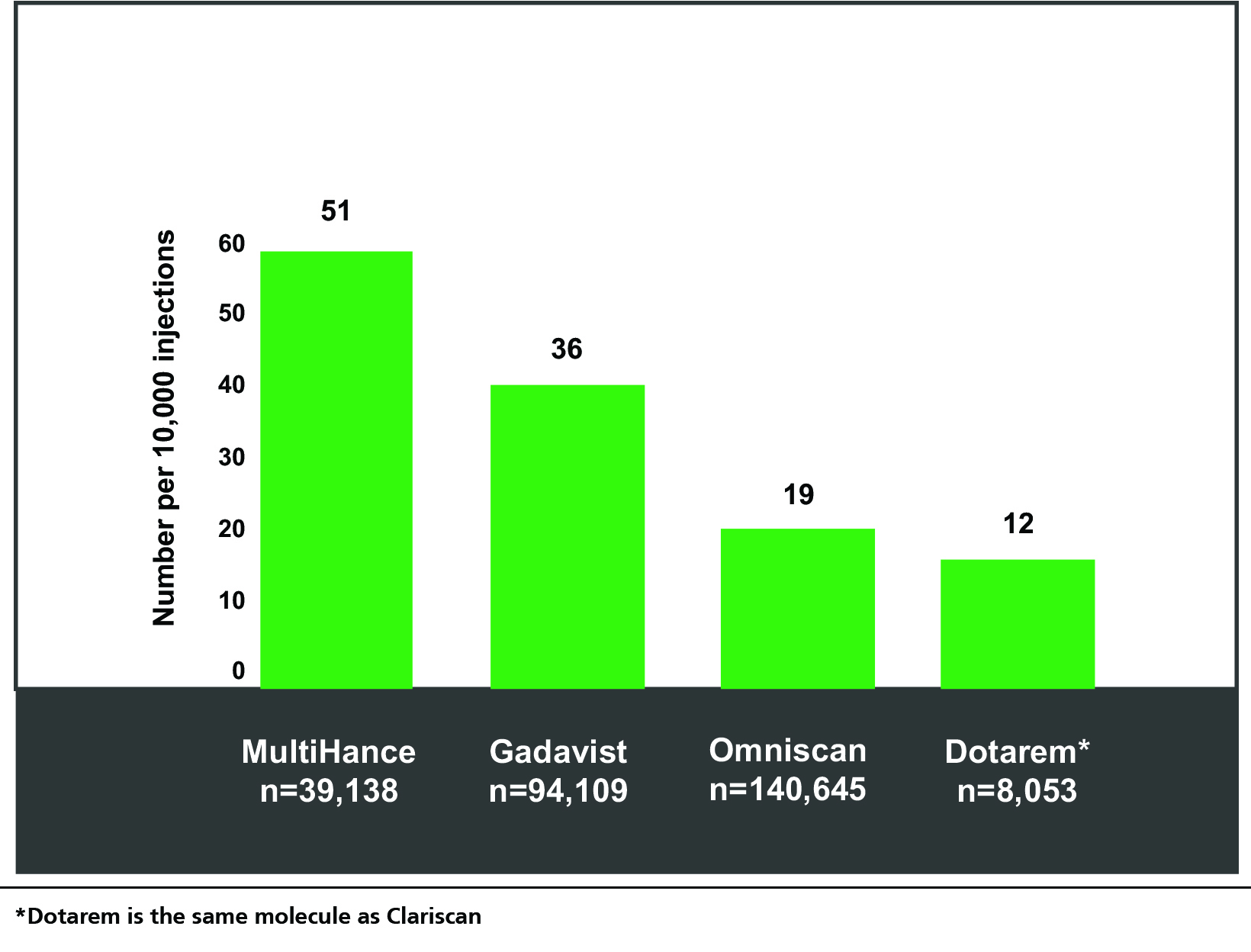
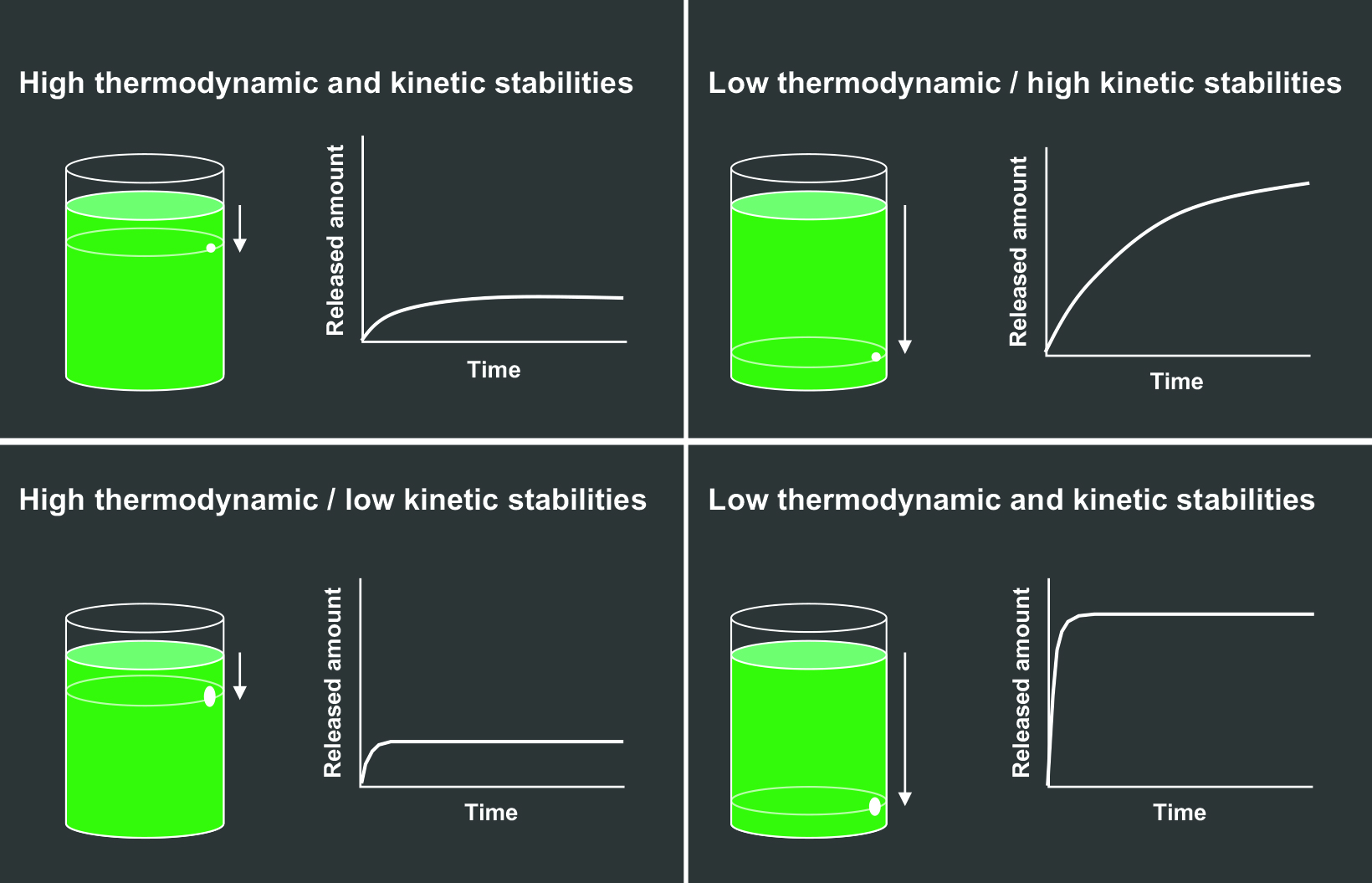
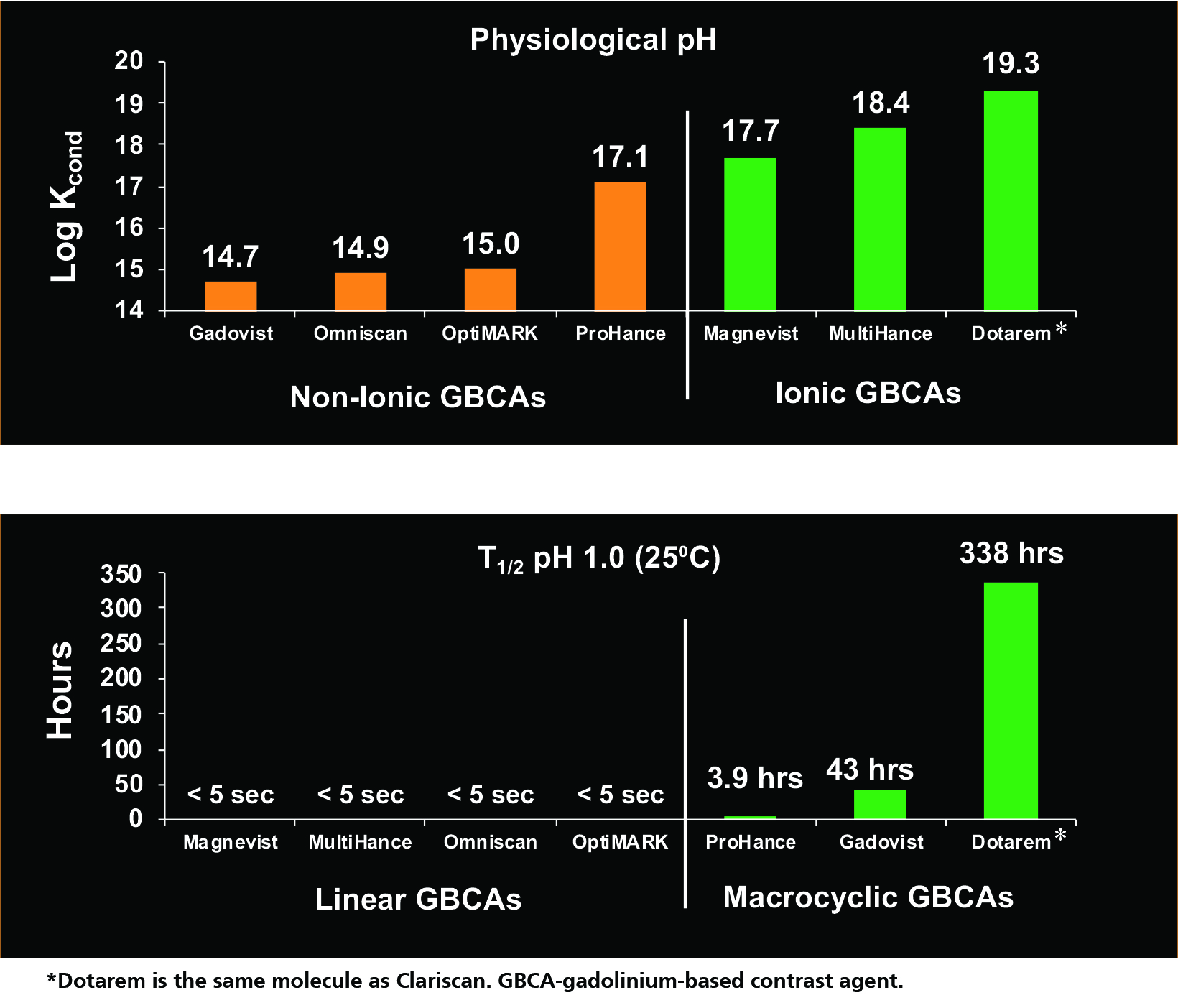
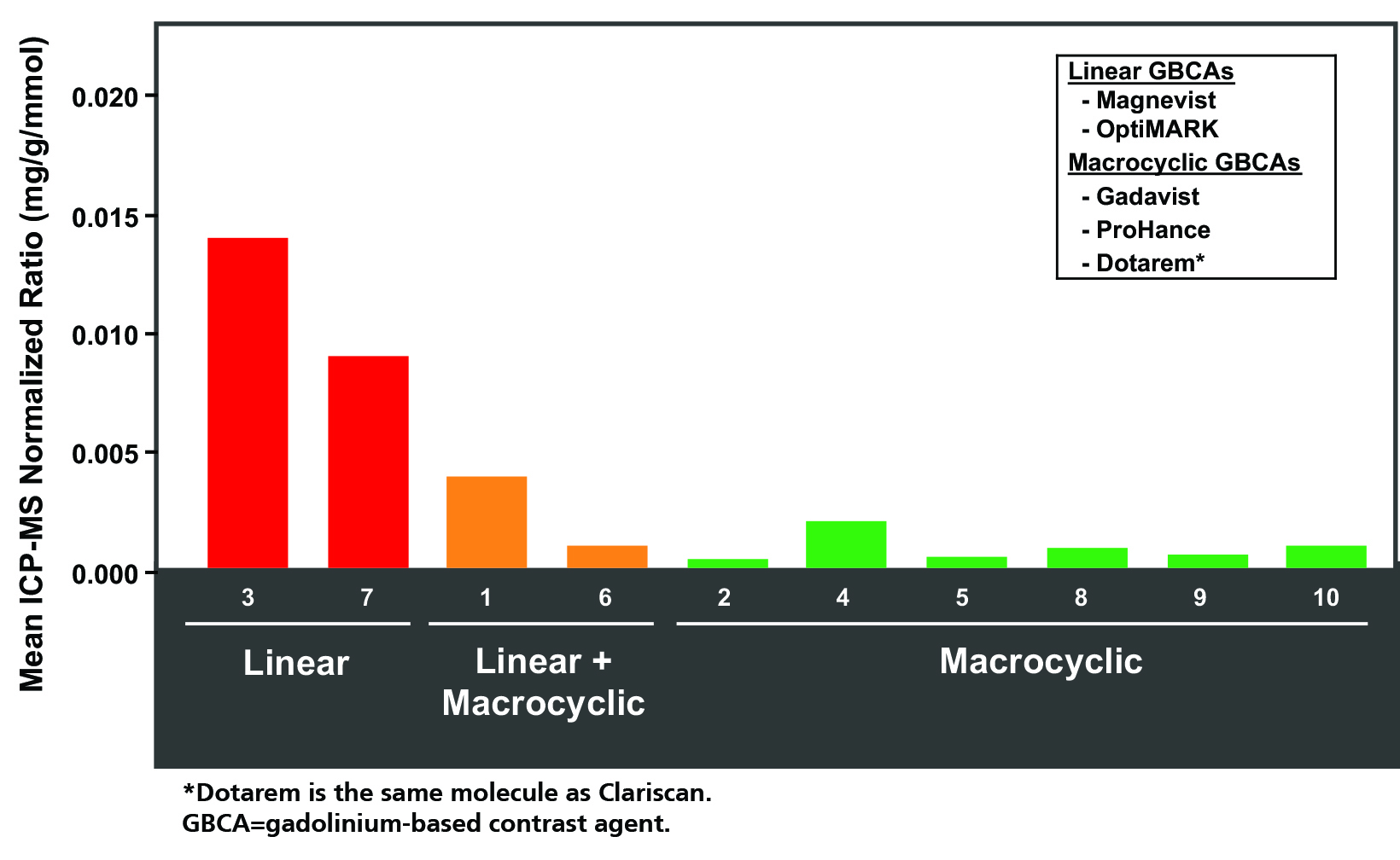
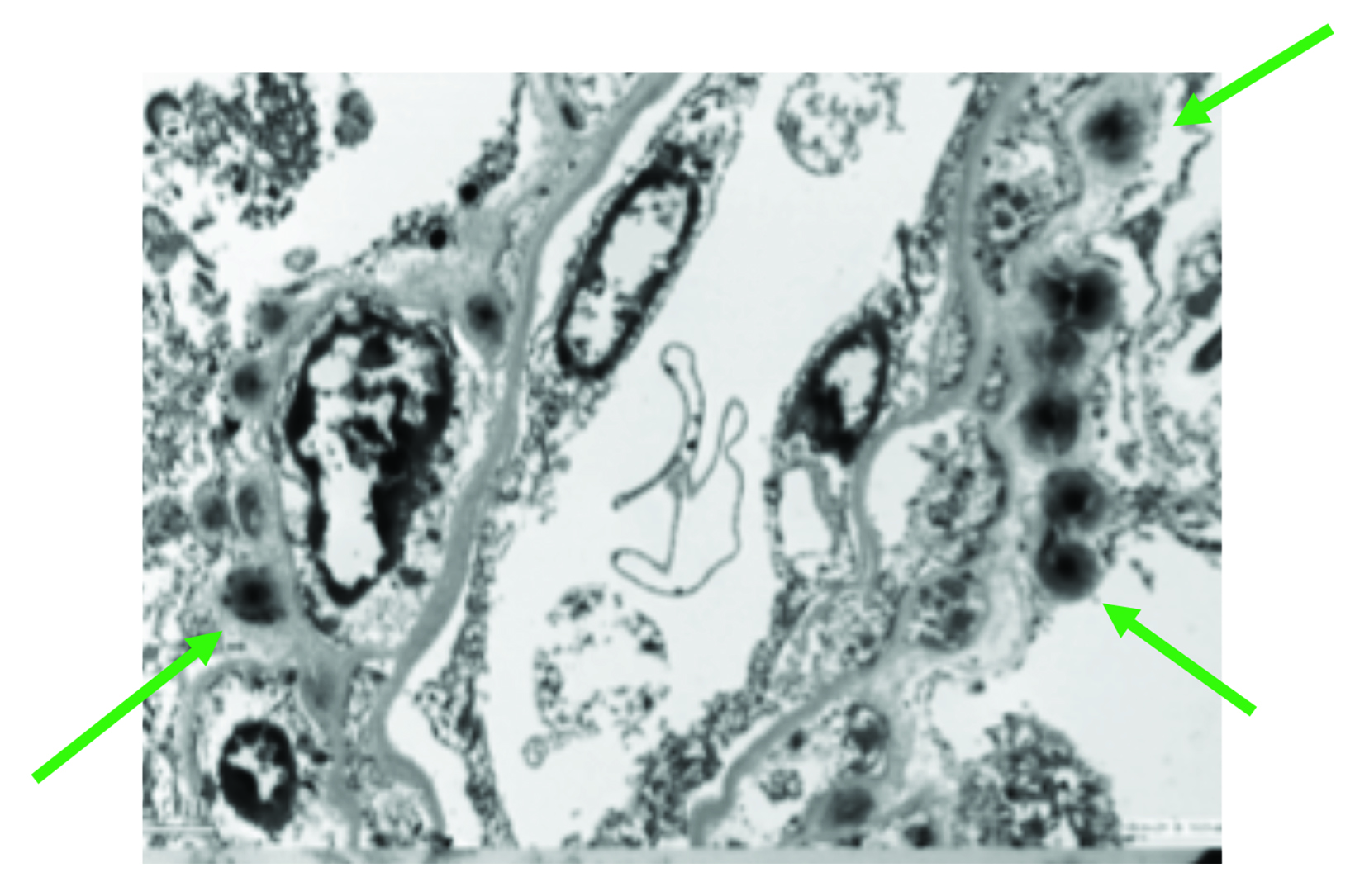
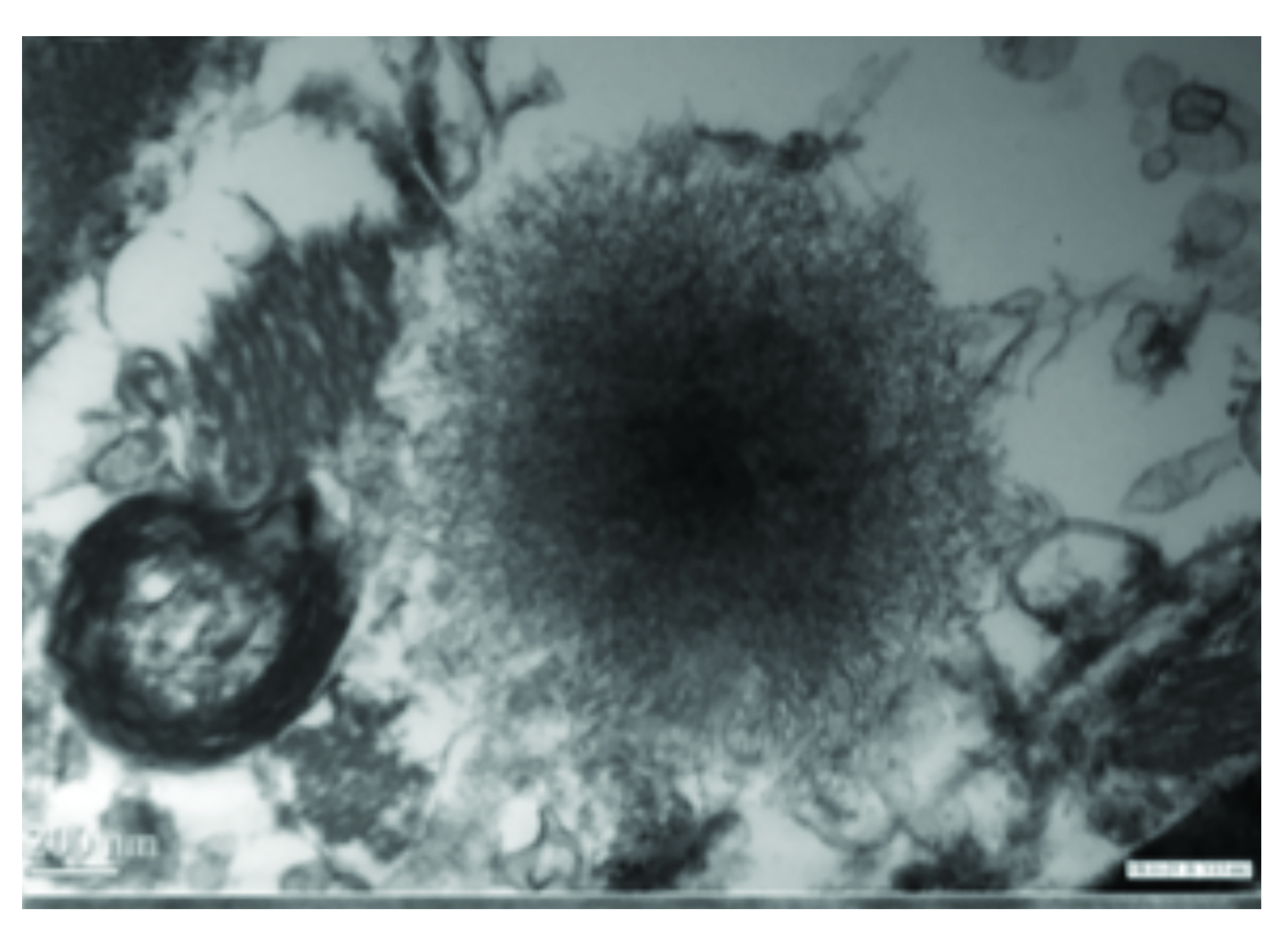
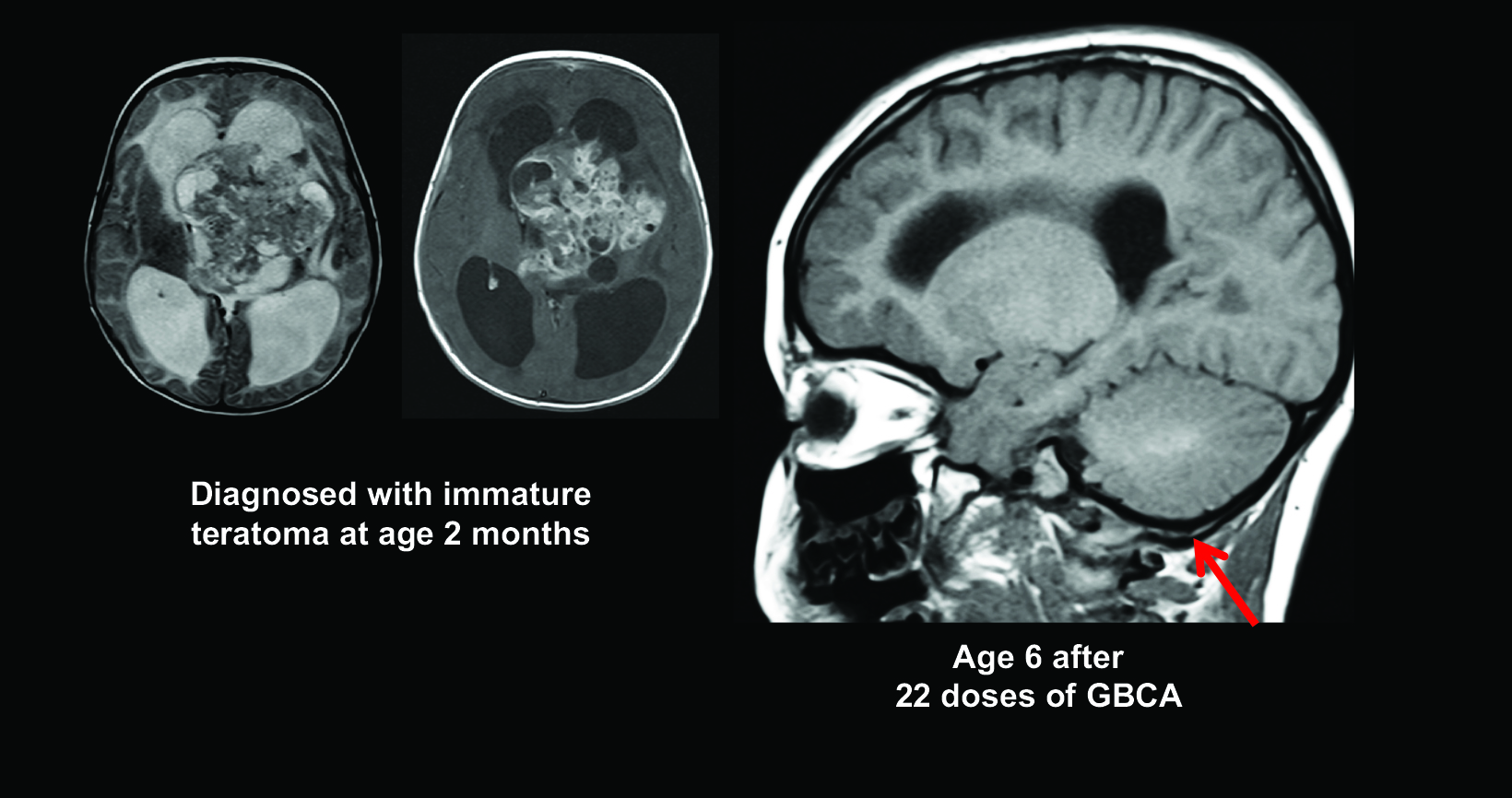
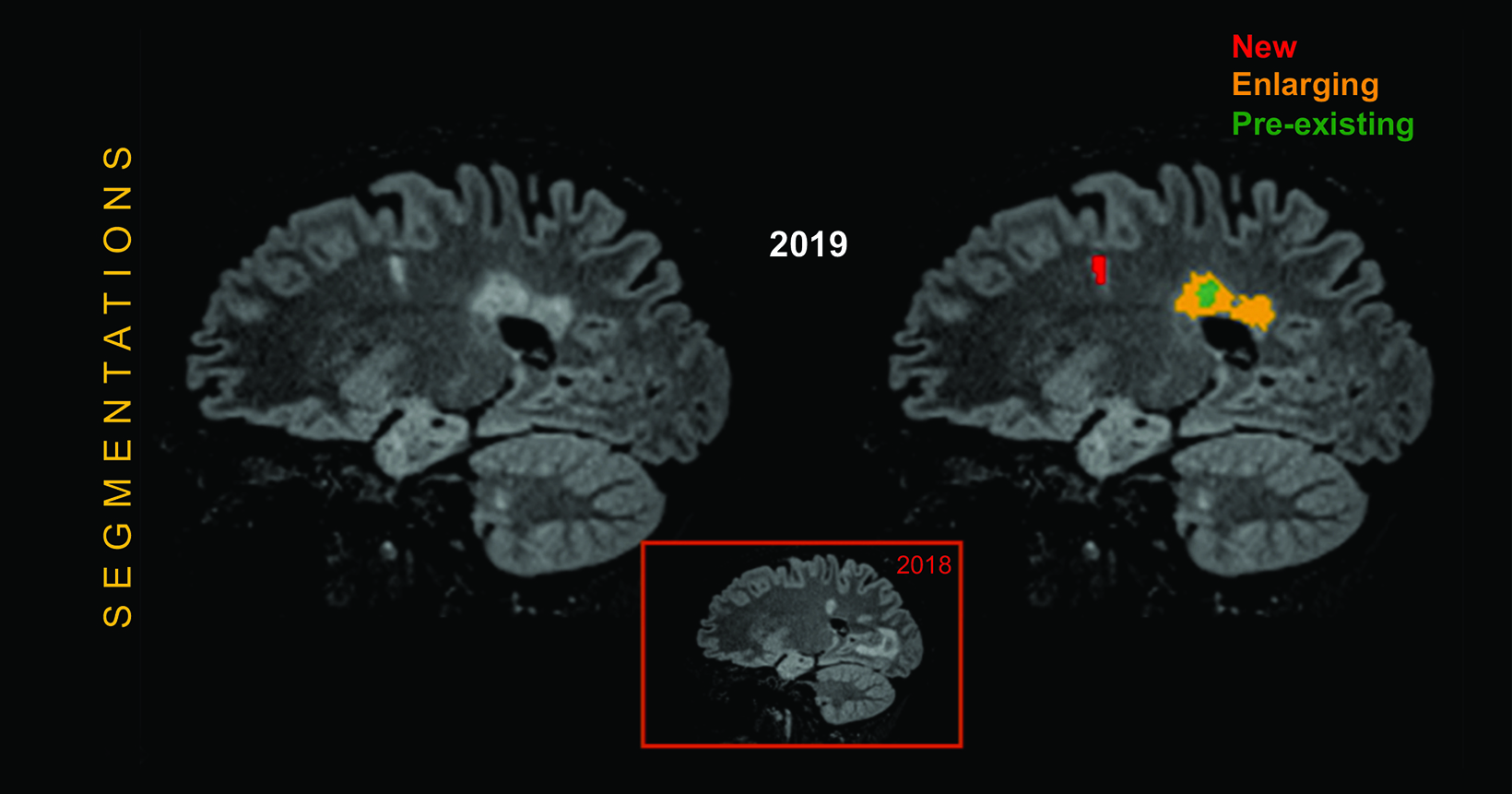
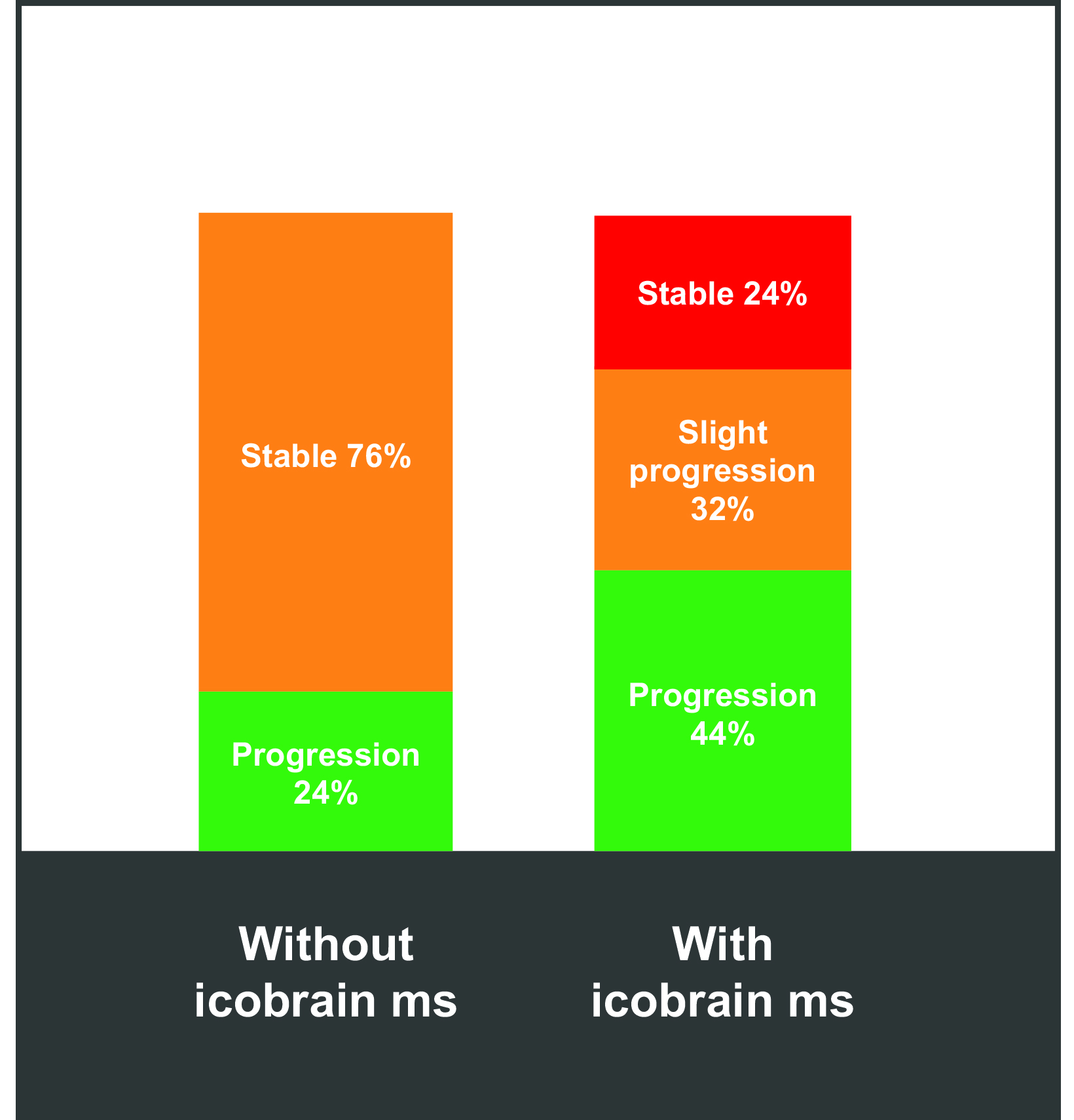
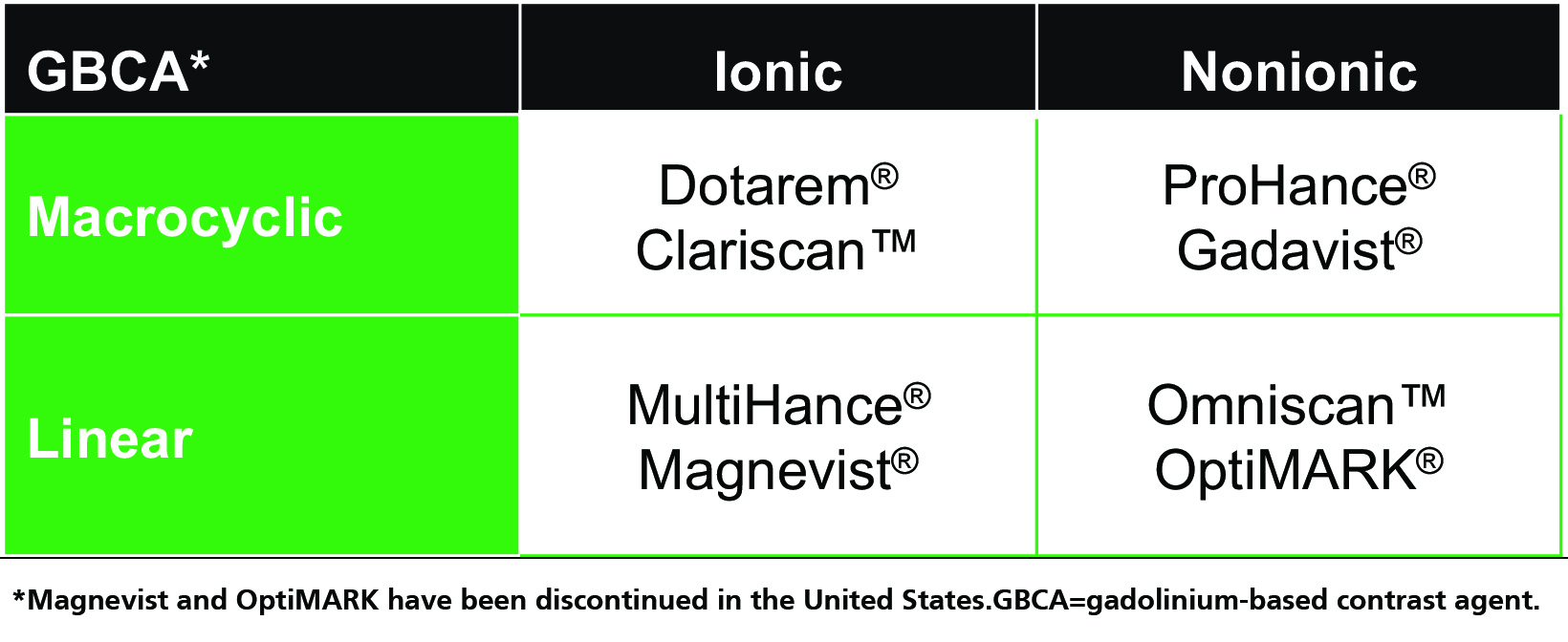
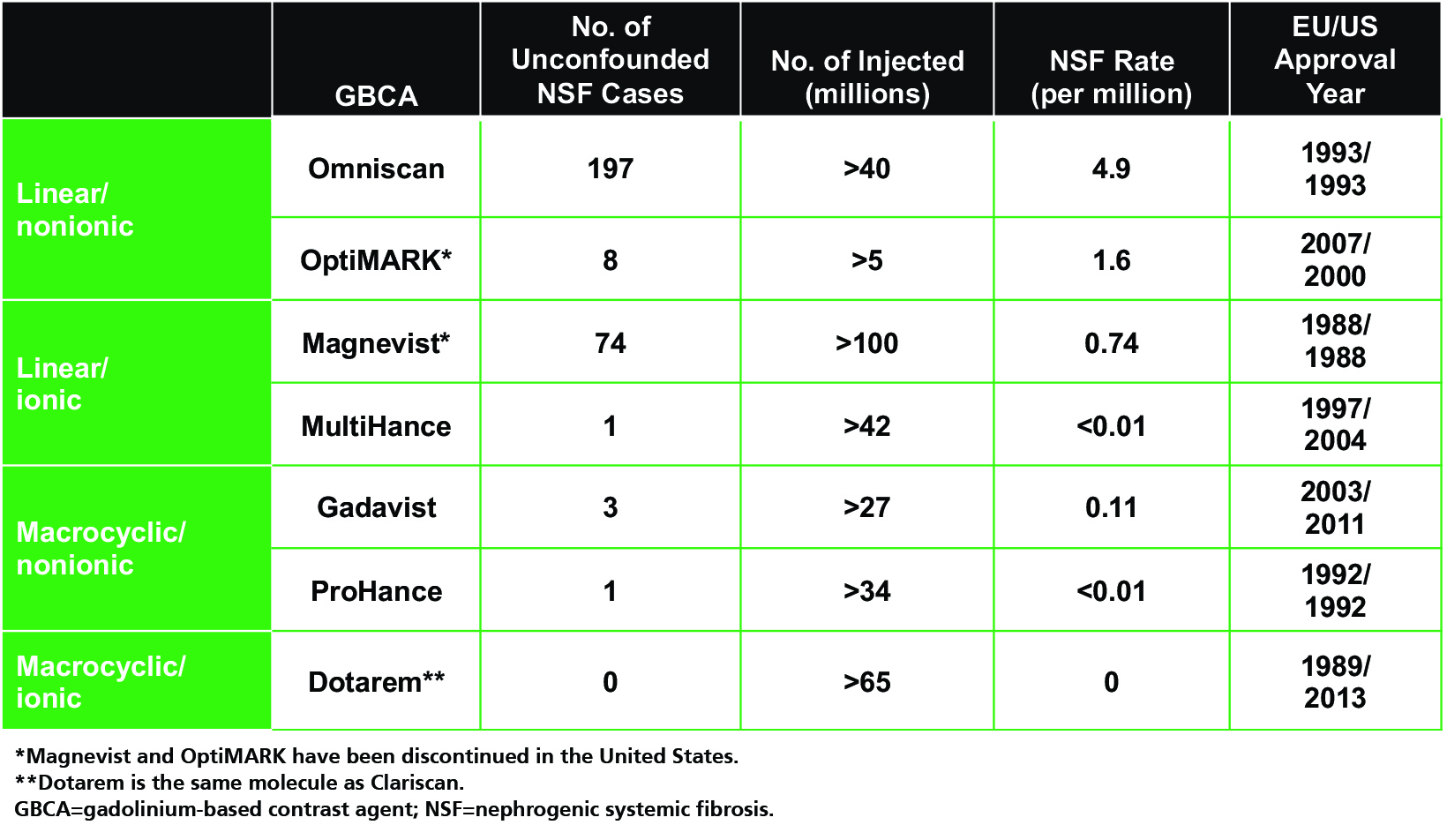
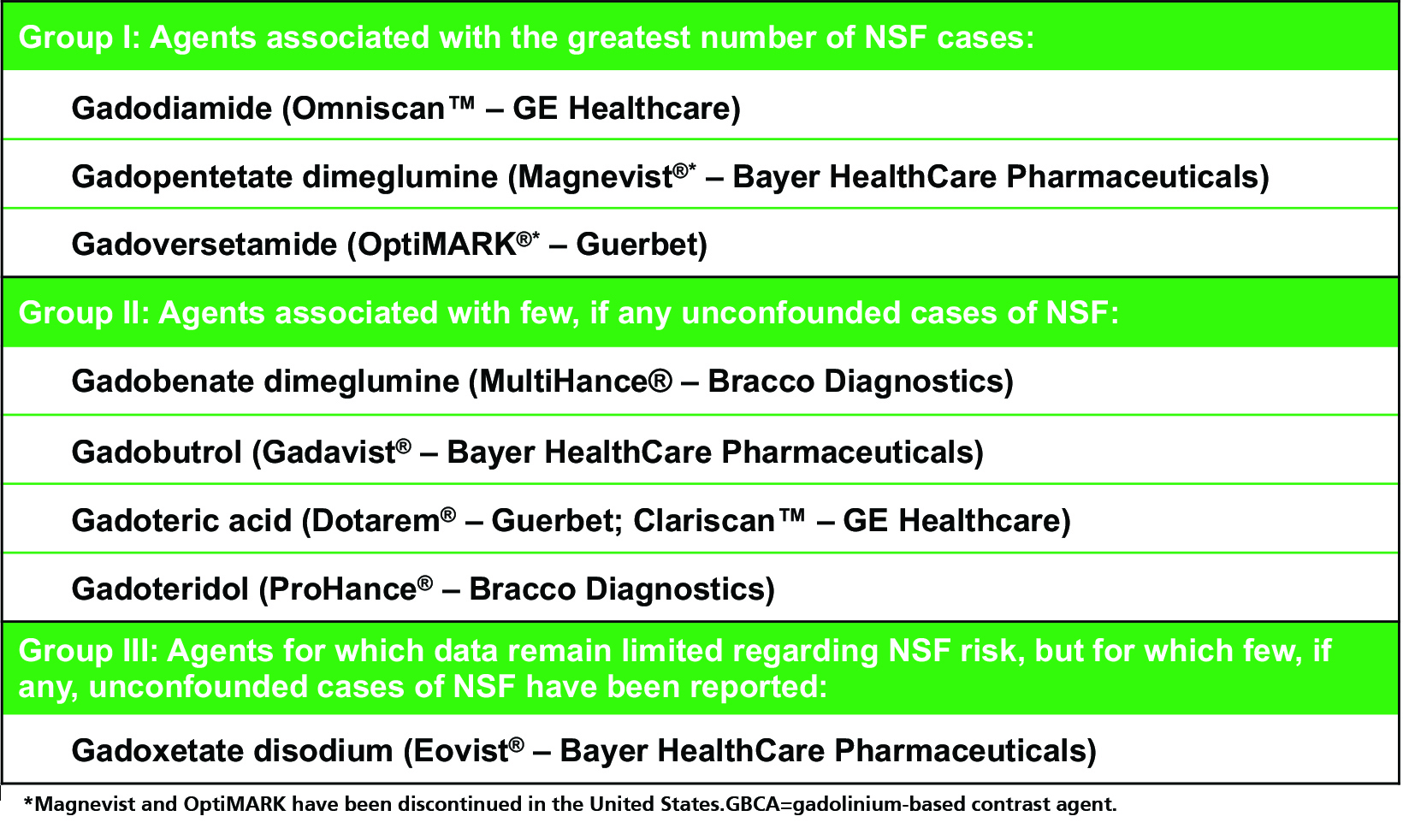
Gadolinium-based contrast agents (GBCAs) for magnetic resonance imaging (MRI) have been used for decades to increase soft-tissue contrast, characterize pathological structures, and detect vascularization and tissue perfusion.1 With over 450 million doses administered worldwide, GBCA safety and efficacy have been well established.2 Available GBCAs include: Dotarem® (gadoterate meglumine), Clariscan™ (gadoterate meglumine; a generic of Dotarem), Gadavist® (gadobutrol), ProHance® (gadoteridol), MultiHance® (gadobenate dimeglumine), and Omniscan™ (gadodiamide).3-8 Magnevist® (gadopentetate dimeglumine) and OptiMARK® (gadoversetamide) have been discontinued in the US.
All GBCA contrast agents are made up of a gadolinium (Gd) ion bound to an organic ligand to form a chelate, and these agents can be classified on the basis of ligand structure (linear or macrocyclic) and ionicity (ionic or nonionic).1,3-10 (Table 1) The specific combination of properties of each GBCA have long been known to affect the stability of the Gd-ligand chelate, resulting in differences in their tendency to dissociate and release free Gd ions, with important potential safety implications. Here we review the short- and long-term adverse effects associated with GBCAs, and strategies to optimize safe GBCA use in at-risk patient populations.
Short-term GBCA Tolerability
Most acute adverse reactions to GBCAs are mild and physiologic. They include coldness, warmth, pain at the injection site, nausea with or without vomiting, headache, paresthesia, and dizziness.11,12 In general, the rates of these reactions are low and comparable among the GBCAs; however, the largest, single-center study of acute reactions to date did show some minor differences in reaction rates among them.13 (Figure 1) Specifically, among the agents studied, the GBCA with the lowest rate of overall reactions was the macrocyclic agent Dotarem (the same molecule as Clariscan), at 12 per 10,000 injections, and the second lowest was the linear agent Omniscan, at 19 per 10,000. Severe reactions were found to be rare (1 per 50,000 injections); indeed, none were reported for Dotarem or Omniscan during the study time frame.
A main risk factor for acute reactions to GBCAs include a previous reaction to a GBCA; this can be an indication for corticosteroid prophylaxis, and it may also be prudent under such circumstances to use a different GBCA. Additionally, those with asthma and other allergies are at increased risk for an acute, allergic-like reaction, but here again the rate is low.12 Overall, GBCAs are very well tolerated; however, personnel trained in recognizing and managing reactions should be immediately available should one occur.12
Long-term GBCA Safety
The two main long-term safety concerns with GBCA administration are nephrogenic systemic fibrosis (NSF) and Gd retention in the organs and tissues in the body, as evidenced by T1 hyperintensity on noncontrast scans in patients having received a GBCA in the past. Both relate to GBCA chelate stability; ie, how tightly the Gd ion is bound to its ligand. Chelate stability is difficult to measure in vivo. Consequently, it is inferred from two stability constants that are measured in vitro: the conditional thermodynamic stability constant (Log Kcond) and the kinetic stability constant (T1/2).14 Conditional thermodynamic stability reflects how much of the Gd dissociates; it is denoted as “conditional” because it is measured at physiologic pH. Kinetic stability reflects how fast dissociation occurs. This measurement is taken under acidic conditions, which are required to observe any dissociation within a reasonable time period.
The effects of the thermodynamic and kinetic stabilities can be graphically depicted by a beaker of liquid with a hole in it, where the liquid represents free Gd that can leak out, or be released.15 (Figure 2) The height of the hole represents the thermodynamic stability, and the diameter of the hole represents the kinetic stability. The higher the hole on the side of the beaker (Figures 2A and 2C), the lower the overall volume of Gd released; the smaller the diameter of the hole (Figures 2A and 2B), the slower the speed of Gd release.15
The conditional thermodynamic and kinetic stability constants for various GBCAs are shown in Figure 3.14 Based on the conditional thermodynamic stability (how much Gd dissociates), ionic GBCAs are much more stable than nonionic GBCAs because the ionic bond is stronger than the nonionic bond. Note that this a logarithmic scale, so seemingly small differences are actually quite large.
Based on the kinetic stability (how quickly dissociation occurs), the linear GBCAs are much less stable, dissociating almost immediately (< 5 sec), while the macrocyclic agents dissociate much more slowly—on the order of hours and days.14 In the experiment shown in Figure 3, Dotarem did not exhibit dissociation after 338 hours, the timepoint at which the experiment was terminated. In summary, the nonionic linear GBCAs are the least stable, and the ionic macrocyclic GBCAs are the most stable, with the remainder falling in between.
Nephrogenic Systemic Fibrosis
Nephrogenic systemic fibrosis is a rare but serious disease observed in patients with end-stage renal disease receiving high and/or multiple doses of less stable GBCAs. GBCA clearance is known to be slower in these patients, presumably permitting a greater accumulation of free Gd not seen in patients with normal kidney function, particularly in those receiving less stable GBCAs.16 This free Gd is believed to be taken up by macrophages, triggering a systemic fibrotic process within the body that can be fulminant and, in rare cases, fatal.16
As one might expect, unconfounded NSF cases (ie, those occurring after administration of only one GBCA) are limited almost exclusively to the linear GBCAs (with the exception of the linear agent MultiHance), even when adjusting for the number of doses administered.3,17-26 (Table 2) The only agent for which no unconfounded NSF cases have been reported is Dotarem.3,26 Such observations led the American College of Radiology (ACR) to stratify GBCAs into three groups according to NSF risk: Group I (Omniscan, Magnevist, and OptiMARK) are associated with the greatest number of NSF cases; Group II (MultiHance, Gadavist, Dotarem, Clariscan and ProHance) are associated with few, if any, unconfounded NSF cases; and Group III (Eovist), for which data remain limited, but few cases have been reported.12 (Table 3) Since the implementation of guidelines limiting exposure of at-risk patients to Group II GBCAs, the incidence of NSF has dropped and today, new cases are exceedingly rare.
Gadolinium Retention
Retention of Gd in both bone and brain tissue was demonstrated some time ago.27,28 Moreover, it was recognized in these early studies that the amount of Gd retention was higher after administration of lower stability GBCAs. Interestingly, in 2011, a publication demonstrating hyperintensity on noncontrast brain scans of patients attributed their findings to the fact that the patients had received brain irradiation.29 Other studies attributed observed signal changes to a specific subtype of multiple sclerosis (MS).30 However, interest in Gd retention has been renewed by a large number of studies performed since 2014 showing hyperintensity on noncontrast brain scans of patients with normal renal function who had received GBCAs in the past.31-36
Taken together, these publications suggest that cumulative GBCA dosing is associated with T1 hyperintensity in patients with normal renal function who have been exposed to linear GBCAs. In addition, the presence of Gd has been confirmed in tissue using inductively coupled plasma mass spectrometry (ICP-MS) and, using ICP-MS, Gd retention has been shown to occur with all GBCAs.36,37 To date, whether the Gd seen using ICP-MS is free or chelated and, if chelated, whether it is chelated to its ligand or another macromolecule, is not known.2 Notably, although Gd retention has been well established by many investigators, no associated clinical sequalae have been definitively found to date.
GBCA Use in At-risk Populations
Pediatric Patients
The benefits of contrast-enhanced MRI in children are well recognized. For indicated pathologies, contrast improves the sensitivity and specificity of MRI, while avoiding the use of radiation. In addition, in children with CNS tumors, use of MRI often means the ability to avoid invasive procedures that might be needed to make a diagnosis, such as CSF sampling to exclude metastatic disease. New advances, such as artificial intelligence (AI) and others, are being successfully used to address the many challenges inherent to pediatric imaging, including the small size of anatomic structures, limited breath-holding capability, and motion artifacts.
Unfortunately, many children with brain tumors and recurrent disease require periodic surveillance. Often, these children are asymptomatic, and recurrence is diagnosed solely by MRI.38-41 Multiple contrast-enhanced MRI scans can quickly add up to significant cumulative doses of Gd. While the risks in children are similar to those in adults, given the potential for large exposures over a lifetime and the vulnerability of these patients, it is important to assess the risks associated with GBCAs, including hypersensitive reactions, NSF, and Gd retention.
Acute allergic-like reactions are even more rare in children than they are in adults. In 2 large studies, the frequency of pediatric acute reactions ranged from 0.04% to 0.10%.42,43 Moreover, NSF is even more rare in children: there have been only 23 reported cases, the youngest being 6 years of age.12,44 Although there are no published reports of NSF in neonates, in theory they may be at risk for NSF if given low-stability Gd agents. In a prospective, multicenter, and observational study to assess the overall safety and efficacy of Dotarem (the same molecule as Clariscan) in 1,631 pediatric patients, only a single adverse event was recorded (vomiting), and no suspicions of NSF were reported at 3-month follow-up.45
As for adults, studies in children have raised concerns for Gd retention in sensitive tissues of the body, including the brain, after repeat exposures.46 Evaluations of hyperintensity within the brains of children after repeated Gd exposure show a pattern that is similar to that seen in adults.47-62 There is generally a stronger signal intensity with increasing doses of linear agents that is not seen with the more stable macrocyclic agents. Also, as in adults, studies in children have shown the corresponding presence of Gd within the brain at autopsy.63-66 Specifically, the average concentration of Gd was higher with exposure to the linear agents compared to the macrocyclic agents, but all agents that were tested demonstrated at least some Gd in the brain.66 (Figure 4) At transmission electron microscopy, Gd can be seen as spherical, electron-dense deposits surrounding blood vessels.65 (Figure 5)
Figure 6 shows images from a child diagnosed with a tumor at two months of age, and by age six, the patient had already received 22 doses of Gd contrast, with visible dentate hyperintensity.
As mentioned, no study thus far has found evidence of any clinical consequence of Gd deposits in the brain; however, it is important to remember that the pediatric brain is known to be sensitive to very small amounts of metals and other man-made chemicals; eg, lead.67 Therefore, the fetus and developing child arguably bear the highest risk from the effects of Gd exposure.
Importantly, comparisons of retained Gd in the brain and bone tissues at autopsy have found that bone levels were 23 times higher, suggesting that the hyperintensity in the brain seen using less-stable agents could be a marker for even higher Gd levels elsewhere in the body.37 Also this may be important, in that pediatric patients are being exposed to large cumulative doses of Gd at a time when they are actively undergoing bone formation.
In light of all of these findings, practices are changing how they approach contrast administration in children. In a 2011 survey of pediatric radiology chairs in the US and Canada, most respondents reported using contrast agents considered by the ACR to be Class I agents; ie, those with a higher association with NSF.68 By 2016, a survey of pediatric physicians with 690 respondents, showed a shift to the more stable Class II agents.69 In fact, over half of respondents reported recently switching to solely using macrocyclic agents. The stated reason was concern about Gd deposition within the brain. Of those respondents who said they were still considering a switch, most indicated they were most likely to switch to the higher stability macrocyclic agent gadoterate meglumine.
Another recent shift, likely driven by increased awareness of the large cumulative doses administered to pediatric patients, has been to question whether Gd is necessary for surveillance MRI. Several studies have evaluated whether contrast informed patient management decisions in children with optic pathway gliomas70 and low-grade gliomas.71,72 In short, the authors concluded that Gd use, particularly for surveillance, should be individualized to the patient and disease. All these studies were retrospective, so prospective studies are needed to confirm when Gd is necessary for patient surveillance. Note that for tumor follow-up, as of 2020, the Response Assessment in Pediatric Neuro-Oncology (RAPNO) working group continues to recommend postcontrast imaging for many tumor types.73-75
Given the clinical benefits of MRI in children, addressing the concerns of family members around the issue of Gd contrast administration is important. Many patients and their caregivers believe the patient is receiving a “dye;” they are unaware that GBCAs are Food and Drug Administration (FDA)-approved drugs that have been used for decades. In a 2018 communication, the FDA made the following recommendation:
“All MRI centers should provide a Medication Guide the first time an outpatient receives a GBCA injection or when the information is substantially changed. In general, hospital inpatients are not required to receive a Medication Guide unless the patient or caregiver requests it.”76
Health care professionals and patients can access the patient Medication Guides, according to the GBCA drug name, on the Medication Guides webpage.77 In a recent publication, a group from the Seattle Children’s Hospital provided excellent guidance on how to discuss concerns about contrast usage with families of patients.78 The authors suggest reassuring patients and their families by letting them know that GBCAs have been in use safely for decades, and over 450 million intravenous doses of contrast agents have been administered. Also, patients and their families may not realize that several GBCAs are available, and it can be reassuring to know their doctor has selected the one most appropriate for the specific examination and patient. Parents are understandably concerned and often a thoughtful and transparent conversation is all that is needed.
In summary, for pediatric patients, it is important to 1) carefully consider whether contrast is needed; 2) consider the retention characteristics when choosing a GBCA, particularly for patients expected to undergo repeated dosing; 3) minimize repeated GBCA imaging studies, particularly closely-spaced MRI studies, whenever possible; and 4) accurately record the agent, the dose, and the cumulative dose. Finally, it is critical that no clinically necessary GBCA MRI scan be avoided or deferred without considering the clinical befits in relation to the potential risks of a contrast agent.
Patients Requiring Repeat MRI Exams
Repeat MRI exams are often required for specific patient populations under certain circumstances. These typically include screening for diseases such as cancer in high-risk patients, and for surveillance following diagnosis and/or treatment for tumors, multiple sclerosis, and inflammatory bowel disease.
Contrast-enhanced MRI is essential for tumor imaging, particularly for obtaining information on the location, classification, and grade of lesions; assisting in biopsy; aiding in treatment planning; and enabling clinicians to monitor response to therapy.79 Since the discovery of NSF and the observation that Gd can accumulate in bodily tissues, regulatory and clinical guidelines have begun to emerge on contrast-enhanced imaging. Currently there are no specific guidelines limiting the dose or frequency of Gd administration; the FDA recommends only minimizing repeat GBCA studies when possible, particularly closely-spaced studies.26 That said, the FDA also recommends that no contrast-enhanced MRI exam deemed to be necessary should be avoided or deferred. Many radiologists reiterate this point and reserve GBCA use for cases in which it has the potential to impact outcomes.
With the recognition that GBCAs should be used judiciously, a new appreciation has emerged for alternative, noncontrast techniques. A main reason to use a GBCA is to increase sensitivity to a lesion or pathology, since a missed disease can lead to mistreatment or undertreatment.88 Therefore, Gd can often be given for initial diagnosis and characterization of tumors, but then less routinely for surveillance. It may be prudent to evaluate whether follow-up MRI exams truly require Gd to effectively monitor lesions; ie, whether they provide additional information that will impact treatment. Examples of cases where Gd may not be needed for surveillance include macroadenoma, vestibular schwannoma, and meningioma. Additionally, alternative sequences and technical advances have made lower-contrast and noncontrast imaging a viable option for many patients. For example, artificial intelligence-based tools may reduce the dose of or even eliminate the need for contrast agents.81
Patients with MS are typically imaged on a regular basis and also when they experience flares. These patients often receive significant cumulative doses of Gd. According the Consortium of MS Centers (CMSC), GBCAs are “necessary for the accurate initial diagnosis of patients experiencing a first clinical attack of symptoms consistent with MS and for following patients with highly active disease or sudden, unexpected declines.”82 However, “GBCAs are optional, although helpful, in many other clinical scenarios, especially when noncontrast MRI can provide answers.”82 This is particularly the case in patients with stable, quiescent MS disease — contrast may not provide additional useful information.
Contrast is often deemed necessary in MS patients with active disease; changes over time are important to determine whether treatment is effective or if the disease is progressing. However, obtaining an accurate count or characterization of lesions is challenging, even with contrast. Side-by-side visual assessment of scans is time-intensive and subjective. In addition, detecting and quantifying subtle changes in lesion volume and number, as well as brain volume loss, can be challenging.
Artificial intelligence tools can help to better segment white matter lesions in patients with MS; better segmentation reveals changes in lesion volume faster and more accurately than can the human eye, providing accurate and quantitative information on T1 hyperintensities and FLAIR hyperintensities. One example is icobrain ms, a software tool that helps radiologists assess subclinical MRI metrics and predict/monitor disability progression and treatment response.83 (Figure 7) Artificial intelligence has also been shown to standardize and improve reporting speed and sensitivity, as well as to decrease intra- and inter-reader variability. 84-89 (Figure 8) Such standardization has been shown to positively impact the quality of care and efficiency of workflows.
Renally Impaired/Elderly Patients
In patients with renal failure, Gd elimination is slowed, resulting in a prolonged circulation time. Specifically, in renally insufficient patients, the elimination half-life of Gd in plasma increases substantially from hours to days, depending on renal function.90 Therefore, with respect to NSF and Gd retention, renally impaired and elderly patients require special care when contrast-enhanced MRI is deemed necessary. The elderly in particular are vulnerable, as their renal dysfunction may be asymptomatic.
Since NSF was observed in patients with end-stage renal disease, many of whom were elderly, care has been taken to avoid Gd contrast whenever possible and to use only those GBCAs deemed to be lowest risk; ie, the ACR Group II agents. This has all but eliminated new cases of NSF. Indeed, the risk of NSF is considered so low with Group II agents that, per the ACR, kidney function screening in potentially at-risk patients is considered optional, even in the elderly.12
With regard to Gd retention, studies have demonstrated that hyperintensity develops earlier and at lower cumulative doses in renally impaired or older patients with poor renal function.91,92 Therefore, recommendations to use the lowest effective dose of a GBCA at the least appropriate frequency would apply in these cases. However, with no known adverse effects associated with Gd retention, it remains important to consider the benefits that may be provided by contrast. More than likely, there is less concern for long-term clinical sequalae from Gd retention in the elderly than in pediatric patients.
Conclusions
Recognizing the significant benefit derived from contrast-enhanced MRI, guidelines nonetheless recommend using such imaging only when it appears more likely than noncontrast imaging to contribute to diagnosis and/or patient management. Moreover, a GBCA that offers the best combination of safety and efficacy should be selected, based on the need for repeat imaging and the patient’s age and level of renal function. Small amounts of Gd are likely to be retained in the body regardless of the GBCA selected. Therefore, ensuring that the benefit of a contrast-enhanced MRI outweighs any potential risk of adverse effects is critical.
References
- Bäuerle T, Saake M, Uder M. Gadolinium-based contrast agents: What we learned from acute adverse events, nephrogenic systemic fibrosis and brain retention. Rofo. 2021;193:1010-1018.
- McDonald RJ, Levine D, Weinreb J, et al. Gadolinium Retention: A Research Roadmap from the 2018 NIH/ACR/RSNA Workshop on Gadolinium Chelates. 2018;289:517-534.
- Dotarem® (gadoteric acid) [prescribing information]. Bloomington, IN: Guerbet LLC; September, 2017.
- Clariscan™ (gadoterate meglumine) [prescribing information]. Marlborough, MA: GE Healthcare Inc.; November, 2019.
- Gadavist® (gadobutrol) injection [prescribing information]. Wayne, NJ; Bayer HealthCare Pharmaceuticals; April 2016.
- ProHance® (Gadoteridol) Injection, [prescribing information]. Princeton, NJ: Bracco Diagnostics Inc.; November 2013.
- MultiHance® (gadobenate dimeglumine) injection [prescribing information]. Princeton, NJ; Bracco Diagnostics Inc.; July 2013.
- Omniscan™ (gadodiamide) injection [prescribing information]. Princeton, NJ: GE Healthcare; August 2013.
- Magnevist® (gadopentetate dimeglumine) injection [prescribing information]. Wayne, NJ: Bayer HealthCare Pharmaceuticals; June 2014.
- OptiMARK™ (gadoversetamide) injection [prescribing information]. Bloomington, IN: Guerbet LLC; August 2016.
- Gulani V, Calamante F, Shellock FG, Kanal E, Reeder SB; International Society for Magnetic Resonance in Medicine. Gadolinium deposition in the brain: summary of evidence and recommendations. Lancet Neurol. 2017;16:564-570.
- American College of Radiology (ACR) Committee on Drugs and Contrast Media. ACR Manual on Contrast Media, 2022. Available at: https://www.acr.org/-/media/ACR/Files/Clinical-Resources/Contrast_Media.pdf. Accessed August 2, 2022.
- McDonald JS, Hunt CH, Kolbe AB, et al. Acute Adverse Events Following Gadolinium-based Contrast Agent Administration: A Single-Center Retrospective Study of 281 945 Injections. Radiology. 2019;292:620-627.
- Port M, Idée JM, Medina C, Robic C, Sabatou M, Corot C. Efficiency, thermodynamic and kinetic stability of marketed gadolinium chelates and their possible clinical consequences: a critical review. Biometals. 2008;21:469-490.
- Idée JM, Port M, Robic C, Medina C, Sabatou M, Corot C. Role of thermodynamic and kinetic parameters in gadolinium chelate stability. J Magn Reson Imaging. 2009;30:1249-1258.
- Perazella MA. Nephrogenic systemic fibrosis, kidney disease, and gadolinium: is there a link? Clin J Am Soc Nephrol. 2007;2:200-202.
- Edwards BJ, Laumann AE, Nardone B, et al. Advancing pharmacovigilance through academic-legal collaboration: the case of gadolinium-based contrast agents and nephrogenic systemic fibrosis-a Research on Adverse Drug Events and Reports (RADAR) report. Br J Radiol. 2014;87:20140307.
- Lohani S, Golenbiewski J, Swami A, Halalau A. A unique case of nephrogenic systemic fibrosis from gadolinium exposure in a patient with normal eGFR. BMJ Case Rep. 2017;2017:bcr2017221016.
- Endrikat J, Schwenke C, Vogtlaender K, Dohannish S, Breuer J. Safety profile of gadoxetate disodium in elderly patients (≥65 years). Acta Radiol. 2018;59:81-88.
- Bracco Diagnostics Inc. Website. MultiHance. Available at: https://imaging.bracco.com/us-en/products/magnetic-resonance-imaging/multihance. Accessed June 11, 2022.
- Bracco Diagnostics Inc. Website. ProHance. Available at: https://imaging.bracco.com/us-en/products/magnetic-resonance-imaging/prohance. Accessed June 11, 2022.
- Diagnostic Imaging Website. Bracco reports case of NSF with gadolinium contrast agent ProHance. Available at: https://www.diagnosticimaging.com/view/bracco-reports-case-nsf-gadolinium-contrast-agent-prohance. Accessed June 11, 2022.
- GE Healthcare Website. Omniscan™. Available at: https://www.gehealthcare.com/products/contrast-media/omniscan. Accessed June 11, 2022.
- Prince MR, Zhang H, Zou Z, Staron RB, Brill PW. Incidence of immediate gadolinium contrast media reactions. AJR Am J Roentgenol. 2011;196:W138-W143.
- Matsumura T, Hayakawa M, Shimada F, et al. Safety of gadopentetate dimeglumine after 120 million administrations over 25 years of clinical use. Magn Reson Med Sci. 2013;12:297-304.
- US Food and Drug Administration Website. Advisory Committee Briefing Document. September 8, 2017. Available at: https://www.fda.gov/media/107506/ download. Accessed June 11, 2022.
- White GW, Gibby WA, Tweedle MF. Comparison of Gd (DTPA-BMA) (Omniscan) versus Gd(HP-DO3A) (ProHance) relative to gadolinium retention in human bone tissue by inductively coupled plasma mass spectroscopy. Invest Radiol. 2006;41:272-278.
- Xia D, Davis RL, Crawford JA, Abraham JL. Gadolinium released from MR contrast agents is deposited in brain tumors: in situ demonstration using scanning electron microscopy with energy dispersive X-ray spectroscopy. Acta Radiol. 2010;51:1126-1136.
- Kasahara S, Miki Y, Kanagaki M, et al. Hyperintense dentate nucleus on unenhanced T1-weighted MR images is associated with a history of brain irradiation. Radiology. 2011;258:222-228.
- Roccatagliata L, Vuolo L, Bonzano L, Pichiecchio A, Mancardi GL. Multiple sclerosis: hyperintense dentate nucleus on unenhanced T1-weighted MR images is associated with the secondary progressive subtype. Radiology. 2009;251:503-510.
- Kanda T, Ishii K, Kawaguchi H, Kitajima K, Takenaka D. High signal intensity in the dentate nucleus and globus pallidus on unenhanced T1-weighted MR images: relationship with increasing cumulative dose of a gadolinium-based contrast material. Radiology. 2014;270:834-841.
- Errante Y, Cirimele V, Mallio CA, Di Lazzaro V, Zobel BB, Quattrocchi CC. Progressive increase of T1 signal intensity of the dentate nucleus on unenhanced magnetic resonance images is associated with cumulative doses of intravenously administered gadodiamide in patients with normal renal function, suggesting dechelation. Invest Radiol. 2014;49:685-690.
- Radbruch A, Weberling LD, Kieslich PJ, et al. Gadolinium retention in the dentate nucleus and globus pallidus is dependent on the class of contrast agent. 2015;275:783-791.
- Quattrocchi CC, Mallio CA, Errante Y, et al. Gadodiamide and dentate nucleus T1 hyperintensity in patients with meningioma evaluated by multiple follow-up contrast-enhanced magnetic resonance examinations with no systemic interval therapy. Invest Radiol. 2015;50:470-472.
- Weberling LD, Kieslich PJ, Kickingereder P, et al. Increased signal intensity in the dentate nucleus on unenhanced T1-weighted images after gadobenate dimeglumine administration. Invest Radiol. 2015;50:743-748.
- McDonald RJ, McDonald JS, Kallmes DF, et al. Intracranial gadolinium deposition after contrast-enhanced MR imaging. Radiology. 2015;275:772-782.
- Murata N, Gonzalez-Cuyar LF, Murata K, et al. Macrocyclic and other non-group 1 gadolinium contrast agents deposit low levels of gadolinium in brain and bone tissue: preliminary results from 9 patients with normal renal function. Invest Radiol. 2016;51:447-453.
- Korones DN, Butterfield R, Meyers SP, Constine LS. The role of surveillance magnetic resonance imaging (MRI) scanning in detecting recurrent brain tumors in asymptomatic children. J Neurooncol. 2001;53:33-38.
- Minn AY, Pollock BH, Garzarella L, et al. Surveillance neuroimaging to detect relapse in childhood brain tumors: a Pediatric Oncology Group study. J Clin Oncol. 2001;19:4135-4140.
- Udaka YT, Yeh-Nayre LA, Amene CS, VandenBerg SR, Levy ML, Crawford JR. Recurrent pediatric central nervous system low-grade gliomas: the role of surveillance neuroimaging in asymptomatic children. J Neurosurg Pediatr. 2013;11:119-26.
- Weiser DA, Kaste SC, Siegel MJ, Adamson PC. Imaging in childhood cancer: a Society for Pediatric Radiology and Children’s Oncology Group Joint Task Force report. Pediatr Blood Cancer. 2013;60:1253-1260.
- Dillman JR, Ellis JH, Cohan RH, Strouse PJ, Jan SC. Frequency and severity of acute allergic-like reactions to gadolinium-containing i.v. contrast media in children and adults. AJR Am J Roentgenol. 2007;189:1533-1538.
- McDonald JS, Larson NB, Kolbe AB, et al. Acute Reactions to Gadolinium-Based Contrast Agents in a Pediatric Cohort: A Retrospective Study of 16,237 Injec- tions. AJR Am J Roentgenol. 2021;216:1363-1369.
- Jan F, Segal JM, Dyer J, LeBoit P, Siegfried E, Frieden IJ. Nephrogenic fibrosing dermopathy: two pediatric cases. J Pediatr. 2003;143:678-681.
- Chang DH, Pracros JP. Safety of gadoterate meglumine in over 1600 children included in the prospective observational SECURE study. Acta Radiol. 2019;60):1450-1456.
- Roberts DR, Holden KR. Progressive increase of T1 signal intensity in the dentate nucleus and globus pallidus on unenhanced T1-weighted MR images in the pediatric brain exposed to multiple doses of gadolinium contrast. Brain Dev. 2016;38:331-336.
- Hu HH, Pokorney A, Towbin RB, Miller JH. Increased signal intensities in the dentate nucleus and globus pallidus on unenhanced T1-weighted images: evi- dence in children undergoing multiple gadolinium MRI exams. Pediatr Radiol. 2016;46:1590-1598.
- Roberts DR, Chatterjee AR, Yazdani M, et al. Pediatric Patients Demonstrate Progressive T1-Weighted Hyperintensity in the Dentate Nucleus following Multiple Doses of Gadolinium-Based Contrast Agent. AJNR Am J Neuroradiol. 2016;37:2340-2347.
- Flood TF, Stence NV, Maloney JA, Mirsky DM. Pediatric Brain: Repeated Exposure to Linear Gadolinium-based Contrast Material Is Associated with Increased Signal Intensity at Unenhanced T1-weighted MR Imaging. Radiology. 2017;282:222-228.
- Schneider GK, Stroeder J, Roditi G, et al. T1 Signal Measurements in Pediatric Brain: Findings after Multiple Exposures to Gadobenate Dimeglumine for Imaging of Nonneurologic Disease. AJNR Am J Neuroradiol. 2017;38:1799-1806
- Radbruch A, Haase R, Kickingereder P, et al. Pediatric Brain: No Increased Signal Intensity in the Dentate Nucleus on Unenhanced T1-weighted MR Images after Consecutive Exposure to a Macrocyclic Gadolinium-based Contrast Agent. Radiology. 2017;283:828-836.
- Tibussek D, Rademacher C, Caspers J, et al. Gadolinium Brain Deposition after Macrocyclic Gadolinium Administration: A Pediatric Case-Control Study.Radiology. 2017;285:223-230.
- Rossi Espagnet MC, Bernardi B, Pasquini L, Figà-Talamanca L, Tomà P, Napolitano A. Signal intensity at unenhanced T1-weighted magnetic resonance in the globus pallidus and dentate nucleus after serial administrations of a macrocyclic gadolinium-based contrast agent in children. Pediatr Radiol. 2017;47:1345-1352.
- Renz DM, Kümpel S, Böttcher J, et al. Comparison of Unenhanced T1-Weighted Signal Intensities Within the Dentate Nucleus and the Globus Pallidus After Serial Applications of Gadopentetate Dimeglumine Versus Gadobutrol in a Pediatric Population. Invest Radiol. 2018;53:119-127.
- Ryu YJ, Choi YH, Cheon JE, et al. Pediatric Brain: Gadolinium Deposition in Dentate Nucleus and Globus Pallidus on Unenhanced T1-Weighted Images Is Dependent on the Type of Contrast Agent. Invest Radiol. 2018;53:246-255.
- Kasper E, Schemuth HP, Horry S, Kinner S. Changes in signal intensity in the dentate nucleus at unenhanced T1-weighted magnetic resonance imaging depending on class of previously used gadolinium-based contrast agent. Pediatr Radiol. 2018;48:686-693.
- Young JR, Pope WB, Bobinski M. Gadolinium Deposition within the Pediatric Brain: No Increased Intrinsic T1-Weighted Signal Intensity within the Dentate Nucleus following the Administration of a Minimum of 4 Doses of the Macrocyclic Agent Gadoteridol. AJNR Am J Neuroradiol. 2018;39:1604-1608.
- Bhargava R, Persad ARL, Bhargava NK, Hawkes M. Multiple Administrations of Gadobutrol in the Pediatric Brain: No Change in T1 Signal at MRI. Radiology. 2018;289:204-209.
- Pozeg P, Forget J, Meuli RA, Maeder P. Age, But Not Repeated Exposure to Gadoterate Meglumine, Is Associated With T1- and T2-Weighted Signal Intensity Changes in the Deep Brain Nuclei of Pediatric Patients. Invest Radiol. 2019;54:537-548.
- Topcuoglu ED, Topcuoglu OM, Semiz Oysu A, Bukte Y. Does Gadoterate Meglumine Cause Gadolinium Retention in the Brain of Children? A Case-Control Study. J Magn Reson Imaging. 2020;51:1471-1477.
- Towbin AJ, Zhang B, Dillman JR. Evaluation of the effect of multiple administrations of gadopentetate dimeglumine or gadoterate meglumine on brain T1- weighted hyperintensity in pediatric patients. Pediatr Radiol. 2021;51:2568-2580.
- Ozturk K, Nascene D. Effect of at Least 10 Serial Gadobutrol Administrations on Brain Signal Intensity Ratios on T1-Weighted MRI in Children: A Matched Case-Control Study. AJR Am J Roentgenol. 2021;217:753-760.
- Roberts DR, Welsh CA, LeBel DP 2nd, Davis WC. Distribution map of gadolinium deposition within the cerebellum following GBCA administration. Neurology. 2017;88:1206-1208.
- McDonald JS, McDonald RJ, Jentoft ME, et al. Intracranial Gadolinium Deposition Following Gadodiamide-Enhanced Magnetic Resonance Imaging in Pediat- ric Patients: A Case-Control Study. JAMA Pediatr. 2017;171:705-707.
- Zhang RR, Matkovic E, Fournelle JH, Richard B, Rowley HA, Salamat S. Histological features of gadolinium deposition in the brain, a case report. Acta Neuropathol. 2020;140:241-244.
- Stanescu AL, Shaw DW, Murata N, et al. Brain tissue gadolinium retention in pediatric patients after contrast-enhanced magnetic resonance exams: pathological confirmation. Pediatr Radiol. 2020;50:388-396.
- Stein J, Schettler T, Wallinga D, Valenti M. In harm’s way: toxic threats to child development. J Dev Behav Pediatr. 2002;23(1 Suppl):S13-S22.
- Trout AT, Dillman JR, Ellis JH, Cohan RH, Strouse PJ. Patterns of intravenous contrast material use and corticosteroid premedication in children—a survey of Society of Chairs of Radiology in Children’s Hospitals (SCORCH) member institutions. Pediatr Radiol. 2011;41:1272-1283.
- Mithal LB, Patel PS, Mithal D, Palac HL, Rozenfeld MN. Use of gadolinium-based magnetic resonance imaging contrast agents and awareness of brain gadolinium deposition among pediatric providers in North America. Pediatr Radiol. 2017;47:657-664.
- Maloney E, Stanescu AL, Perez FA, et al. Surveillance magnetic resonance imaging for isolated optic pathway gliomas: is gadolinium necessary? Pediatr Radiol. 2018;48:1472-1484.
- Campion T, Quirk B, Cooper J, et al. Surveillance imaging of grade 1 astrocytomas in children: can duration and frequency of follow-up imaging and the use of contrast agents be reduced? Neuroradiology. 2021;63:953-958.
- Malbari F, Chintagumpala MM, Wood AC, et al. Gadolinium is not necessary for surveillance MR imaging in children with chiasmatic-hypothalamic low-grade glioma. Pediatr Blood Cancer. 2021;68:e29178.
- Fangusaro J, Witt O, Hernáiz Driever P, et al. Response assessment in paediatric low-grade glioma: recommendations from the Response Assessment in Pediatric Neuro-Oncology (RAPNO) working group. Lancet Oncol. 2020;21:e305-e316.
- Erker C, Tamrazi B, Poussaint TY, et al. Response assessment in paediatric high-grade glioma: recommendations from the Response Assessment in Pediatric Neuro-Oncology (RAPNO) working group. Lancet Oncol. 2020;21:e317-e329.
- Cooney TM, Cohen KJ, Guimaraes CV, et al. Response assessment in diffuse intrinsic pontine glioma: recommendations from the Response Assessment in Pediatric Neuro-Oncology (RAPNO) working group. Lancet Oncol. 2020;21:e330-e336.
- US Food and Drug Administration Website. FDA Drug Safety Communication. May 16, 2018 Update. Available at: https://www.fda.gov/drugs/drug-safety-and-availability/fda-drug-safety-communication-fda-warns-gadolinium-based-contrast-agents-gbcas-are-retained-body#:~:text=05%2D16%2D2018%20 Update&text=All%20MRI%20centers%20should%20provide,patient%20or%20caregiver%20requests%20it. Accessed June 11, 2022.
- US Food and Drug Administration Website. Medication Guides. Available at: https://www.fda.gov/drugs/drug-safety-and-availability/medication-guides. Accessed June 11, 2022.
- Noda SM, Oztek MA, Stanescu AL, Maloney E, Shaw DWW, Iyer RS. Gadolinium retention: should pediatric radiologists be concerned, and how to frame conversations with families. Pediatr Radiol. 2022;52:345-353.
- Lohrke J, Frenzel T, Endrikat J, et al. 25 Years of Contrast-Enhanced MRI: Developments, Current Challenges and Future Perspectives. Adv Ther. 2016;33:1-28.
- Falk Delgado A, Van Westen D, Nilsson M, et al. Diagnostic value of alternative techniques to gadolinium-based contrast agents in MR neuroimaging—a comprehensive overview. Insights Imaging. 2019;10:84.
- Gong E, Pauly JM, Wintermark M, Zaharchuk G. Deep learning enables reduced gadolinium dose for contrast-enhanced brain MRI. J Magn Reson Imaging. 2018;48:330-340.
- Wattjes MP, Ciccarelli O, Reich DS, et al.; Magnetic Resonance Imaging in Multiple Sclerosis study group; Consortium of Multiple Sclerosis Centres; North American Imaging in Multiple Sclerosis Cooperative MRI guidelines working group. 2021 MAGNIMS-CMSC-NAIMS consensus recommendations on the use of MRI in patients with multiple sclerosis. Lancet Neurol. 2021;20:653-670.
- GE Healthcare Website. icobrain ms. Available at: https://apps.gehealthcare.com/app-products/icobrain-ms. Accessed May 26, 2022.
- Van Hecke W, Costers L, Descamps A, et al. A Novel Digital Care Management Platform to Monitor Clinical and Subclinical Disease Activity in Multiple Sclerosis. Brain Sci. 2021;11:1171.
- Dickerson E, Davenport MS, Syed F, et al.; Michigan Radiology Quality Collaborative. Effect of Template Reporting of Brain MRIs for Multiple Sclerosis on Report Thoroughness and Neurologist-Rated Quality: Results of a Prospective Quality Improvement Project. J Am Coll Radiol. 2017;14:371-379.e1.
- Alessandrino F, Bastianello S. Re: “Computer-Aided Detection Can Bridge the Skill Gap in Multiple Sclerosis Monitoring.” J Am Coll Radiol. 2018;15(1 Pt A):7-8.
- van Heerden J, Rawlinson D, Zhang AM, et al. Improving Multiple Sclerosis Plaque Detection Using a Semiautomated Assistive Approach. AJNR Am J Neuroradiol. 2015;36:1465-1471.
- Beadnall HN, Wang C, Van Hecke W, Ribbens A, Billiet T, Barnett MH. Comparing longitudinal brain atrophy measurement techniques in a real-world multiple sclerosis clinical practice cohort: towards clinical integration? Ther Adv Neurol Disord. 2019;12:1756286418823462.
- Struyfs H, Sima DM, Wittens M, et al. Automated MRI volumetry as a diagnostic tool for Alzheimer’s disease: Validation of icobrain dm. Neuroimage Clin. 2020;26:102243.
- Aime S, Caravan P. Biodistribution of gadolinium-based contrast agents, including gadolinium deposition. J Magn Reson Imaging. 2009;30:1259-1267.
- Kartamihardja AA, Nakajima T, Kameo S, Koyama H, Tsushima Y. Impact of Impaired Renal Function on Gadolinium Retention After Administration of Gadolinium-Based Contrast Agents in a Mouse Model. Invest Radiol. 2016;51:655-660.
- Barbieri S, Schroeder C, Froehlich JM, Pasch A, Thoeny HC. High signal intensity in dentate nucleus and globus pallidus on unenhanced T1-weighted MR images in three patients with impaired renal function and vascular calcification. Contrast Media Mol Imaging. 2016 May;11:245-250
References
Citation
LN T, D R,. The Use of Contrast-enhanced MRI in At-risk Patient Populations. Appl Radiol. 2021;(5):supplement.
September 1, 2022