The Future of Magnetic Resonance Imaging Contrast Agents
By Minton LE, Pandit R, Willoughby WR, Porter KK
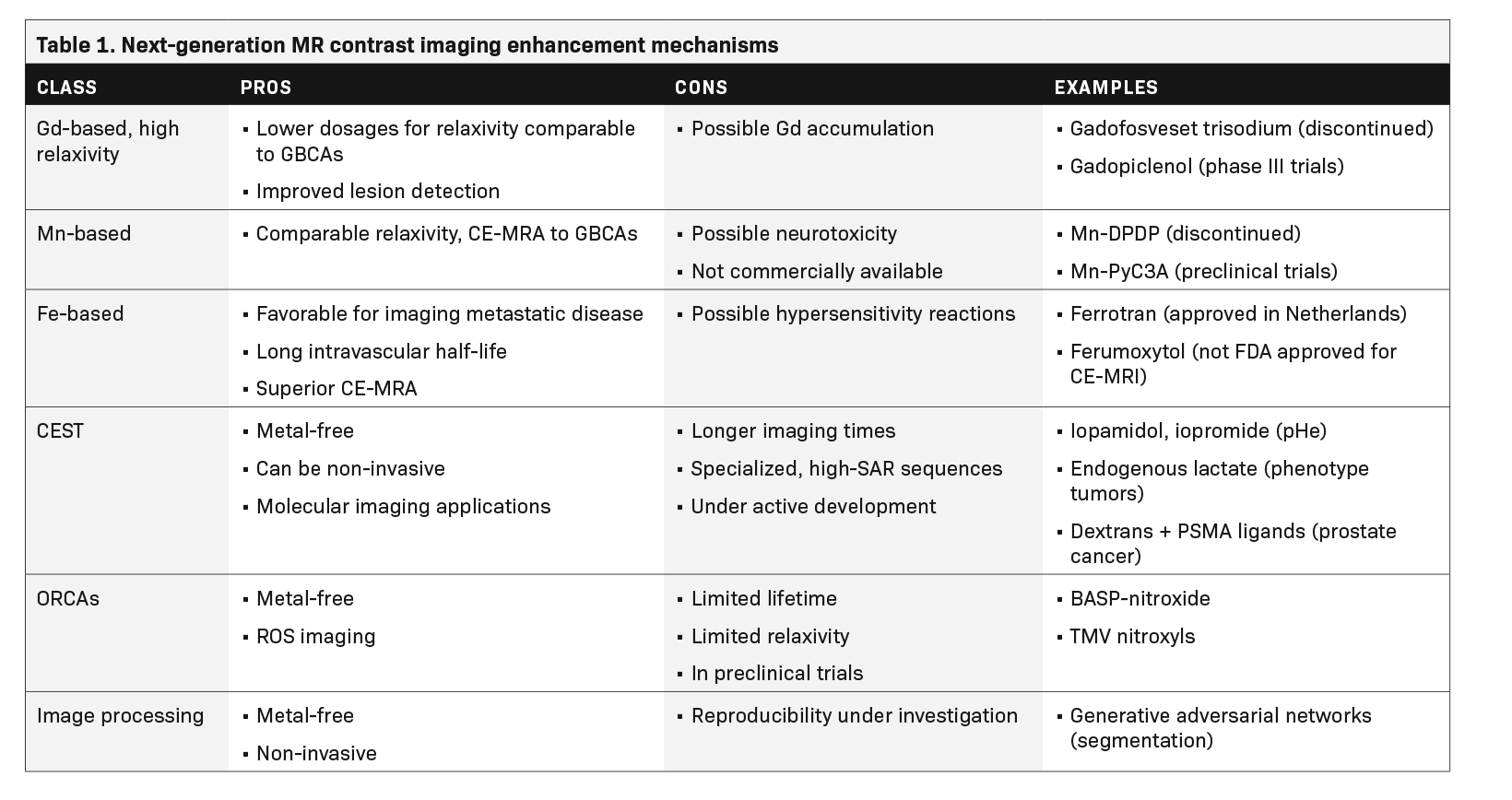
This article is accredited for one SA-CME credit and is available at appliedradiology.org/SAM2.
Gadolinium (Gd)-based contrast agents (GBCAs) dominate the current contrast-enhanced magnetic resonance imaging (CE-MRI) market and are proven to increase the efficacy of diagnostic magnetic resonance (MR) scans.1 Adverse side effects of GBCAs are uncommon,2 and the reported GBCA-associated incidence of nephrogenic systemic fibrosis (NSF) has declined due to pre-MR patient screening and replacement of linear GBCAs with macrocyclic compounds.3 Recently, however, there has been concern about Gd de-chelation from GBCAs and possible effects of bioaccumulation in the central nervous system.4-7
The future of MR contrast may see a replacement of Gd with alternative metals, such as manganese (Mn) or iron-based agents. Metal-based paramagnetic contrast agents are limited by the intrinsic toxicity of many metal ions, their persistence in tissue, and their ability to provide only one type of contrast.8 This has led researchers to pursue contrast mechanisms that do not require metals. A promising example is chemical exchange dependent saturation transfer (CEST) MRI, which can generate clinically useful contrasts using diamagnetic molecules with exchangeable protons in amine, amide and hydroxyl groups.9-15
Recent work has also been done to improve the relaxivity and in vivo lifetime of metal-free, nitroxide-based contrast agents.16 Alternatively, the development of sequences, arrays of coils, k-space strategies, stochastic imaging, and machine learning-based image analysis procedures17 have provided numerous opportunities to improve image contrast in MRI.18 MRI sequences and post-processing techniques may be developed to replace or decrease the use of contrast agents (for example 4D MRI instead of MRA and CEST imaging) or hybrid technologies such as PET/MR may rely on radiotracers in lieu of MR contrast agents.19
Higher-relaxivity Gadolinium agents
Given the history of NSF and Gd retention in the brain and other organs, the lowest GBCA dose should be used that provides enough enhancement for diagnosis in routine practice.20 Thus, the development of next-generation high-relaxivity GBCAs meets a real medical need. By revisiting the GBCA dose-response relationship, such agents would allow reduction of the injected Gd dose with the same efficacy or improvement in the rate of lesion detection and characterization with the usual dose.
An example of a high-relaxivity GBCA is the blood pool agent gadofosveset trisodium, which noncovalently binds to human serum albumin at the proportion of 80% to 90%.21 Gadofosveset trisodium was indicated for MRA; however, after poor sales, production was discontinued in 2017. Another high-relaxivity macrocyclic agent, Gadopiclenol, is currently in phase III clinical trials.22 With its higher relaxivity, Gadopiclenol may only require half of a standard Gd dose to reach the same efficacy compared to agents already available.23
Manganese-based agents
Manganese (Mn)-based products have been identified as a potential alternative to GBCAs.24 Two Mn-based agents, commercially sold as Teslascan (Mangafodipir) and LumenHance, have been available in the past,25 but were withdrawn from the US market due to toxicity concerns and poor sales. As of 2021, no Mn-based contrast agent is currently commercially available, and it is unknown whether Mn products will become a safer alternative to GBCAs as approved MR contrast agents.
Manganese dipyridoxildiphosphate (Mn-DPDP), marketed as Teslascan (Mangafodipir), was first used in humans as an intravenous, paramagnetic, hepatobiliary MR contrast agent for the detection and characterization of liver lesions.25 This water-soluble molecule was taken up selectively by hepatocytes; it dissociated in vivo into the metal and chelate. The Mn was absorbed intra-cellularly and excreted in bile, while the chelate was eliminated via renal filtration.25 More recently, Mn-PyC3A was shown in studies with baboons and mice to provide comparable relaxivity and contrast enhanced MR angiography to GBCAs.26,27 The PyC3A component serves as a chelator, which helps to prevent hepatic uptake and optimizes elimination.26
These studies demonstrate that Mn-PyC3A is excreted both renally and partially by the hepatobiliary system, with near complete elimination (>99.5% after 24 hours).28 This partial hepatobiliary elimination allows for delayed phase visualization of liver metastases in mouse models.28 PET-MRI studies in rat models with renal impairment showed that Mn-PyC3A was eliminated more completely from nephrectomy rats than gadoterate meglumine was, and that this was the result of increased hepatobiliary excretion.29 Although more studies are needed, these studies indicate that Mn-PyC3A has potential as a contrast agent in humans.
Studies of industrial workers exposed to Mn-containing fumes, suggest that Mn may induce neurotoxicity in human brains with behavioral consequences and cognitive deficits because of decreased globus pallidus and cerebellar volume.30 This is an important consideration, given that the Gd retention in the brain has, as of yet, not shown neurotoxicity. As such, it is uncertain whether the benefit-risk balance of Mn-based agents will ultimately reach or exceed that of GBCAs.
Iron-based agents
The identification of iron-based agents as a potential alternative to GBCAs has led to extensive preclinical and clinical research, although the only MR contrast agent commercially available is Ferrotran (formerly Combidex, ferumoxtran-10), an iron oxide nanoparticle approved in the Netherlands for detection of metastatic disease in lymph nodes.31 Iron oxide is a super-paramagnetic agent that leads to a disproportionate shortening of the T2 time, resulting in a strong decrease in the MR signal and thus producing a negative contrast in vivo. Additionally, when iron oxide particles are present at low concentration in the blood stream, they can also produce a signal enhancement on T1 sequences with very short echo times and produce positive contrast. After intravenous administration, the ultra-small formulations of superparamagnetic particles of iron oxide are removed from the blood by the reticuloendothelial system in the liver, spleen, and lymph nodes, a helpful distribution when considering imaging metastatic disease. These agents also have an extremely long intravascular half-life, making iron-based agents an attractive choice for MRA.32
Feraheme (ferumoxytol) has been approved for iron supplementation in patients with end-stage renal disease. While it has been used to visualize the vasculature in children and adults with MR, it is not FDA approved as an MRI contrast agent. Moreover, its labeling includes a boxed warning concerning the risk of fatal and serious hypersensitivity reactions as well as a contraindication for patients who have had an allergic reaction to any intravenous iron replacement product.33 In a 2015 study with 15 renal transplant participants, ferumoxytol was shown to deliver superior vessel sharpness with high-resolution steady state MRA and equivalent signal to noise ratio when compared to standard first-pass MRA. Because it is not Gd based, ferumoxytol can be used without concern for NSF or Gd retention.34
Chemical exchange saturation transfer (CEST) imaging
A relatively new class of contrast agents known as CEST agents have been studied and developed since 2000. In a process similar to magnetization transfer contrast (MTC), these agents provide contrast via selective radiofrequency (RF) saturation of the nuclear magnetization of protons on the agent molecules and subsequent chemical exchange of these saturated protons with water protons. After many successive saturation pulses and exchanges, a detectable decrease in the nearby water signal accumulates and generates contrast enhancement.
An appealing advantage of many of these contrast agents is the lack of paramagnetic metal that may lead to side effects or lasting health effects. Other promising features of the technique are its ability to provide molecularly specific information and its wide applicability. However, CEST imaging sequences are currently limited by long acquisition times, increased specific absorption of radiation (SAR), and strong dependence on experimental factors like field inhomogeneities.35
The CEST technique using contrast agents like iopamidol and iopromide has been successful in measuring extracellular pH (pHe) for the detection and characterization of tumors and even for the evaluation of tumor acidosis.9,10,13 Since the only requirement for a compound to be utilized for CEST contrast is having a proton that is exchangeable with water, the CEST technique can be used for clinically relevant molecular imaging with MRI.36
For example, extracellular lactate, a diamagnetic CEST (diaCEST) agent was successfully imaged using a paramagnetic shift reagent.37 This technique could eventually be used to detect not only the presence of tumors, but also their specific phenotypes. Diamagnetic dextran, a glucose polymer that is already clinically indicated as a plasma volume expander, has been modified with prostate-specific membrane antigen (PSMA) targeting ligands to provide MRI contrast specifically for prostate cancer without metals or radiotracers.38
The CEST technique also opens the door to theranostic applications without the use of radiotracers. Many pharmaceuticals cannot be imaged directly with MRI but contain exchangeable protons. This provides the possibility of image-guided drug delivery and pharmacokinetic studies without the risks associated with ionizing radiation.36 CEST contrast, however, requires specialized MR sequences that can increase SAR and prolong clinical examination times.
Organic radical contrast agents (ORCAs)
Another class of metal-free contrast agents uses the magnetic interaction of unpaired electrons on organic radical moieties and nearby water protons to modulate MR contrast. These organic radical contrast agents (ORCAs) provide another avenue to metal-free MR contrast enhancement, and since they are paramagnetic, do not require specialized pulse sequences with long, adiabatic RF pulses to provide contrast enhancement, like CEST agents. However, the stable organic radical nitroxide has only one unpaired electron, compared to the seven unpaired electrons on the Gd3+ ion or five unpaired electrons on the Fe3+ ion, which limits the relaxivity and contrast enhancement provided by the molecule.39
The appeal of metal-free organic contrast agents has led to broad interest in addressing the limitations of nitroxide-based MR contrast, which include the relatively low relaxivity and rapid metabolic reduction of nitroxide to molecules that do not enhance contrast.39 Rajca, et al, demonstrated that nitroxide radicals on polymeric scaffolds can increase the lifetime of ORCAs in vivo and provide proton relaxivity r1 close to that of GBCAs, about 5 mM-1 s-1. Nguyen, et al, showed that synthesizing nitroxide radicals into brush-arm star polymeric (BASP) structures substantially increases both the relaxivity due to a larger concentration of paramagnetic species and the half-life of ORCAs in vivo. MR contrast enhancement with r2 relaxivities greater than 1000 mM-1 s-1 in rodents was observed up to 24 hours post-injection of BASP-ORCAs.40
Nguyen, et al, also showed the usefulness of BASP-ORCAs as theranostic agents, where the relaxivity could be modulated with drug-activated triggers.41 Dharmawardana, et al, synthesized nitroxyl groups onto rigid tobacco mosaic virus (TMV) protein coats to achieve r1 relaxivity of 1.5 mM-1 s-1 and r2 relaxivity of 4.5 mM-1 s-1 at 1.5 T, which is on the order of relaxivities of GBCAs.42 TMV-based contrast agents could also be used to image superoxide production in cases of deep tissue injury due to their unique structure that prevents the quenching of fluorophores by nearby free radicals.42 None of these compounds have progressed further than preclinical testing, however, and further developments need to be made before ORCAs are approved for clinical use.
Contrast Without the Agents
Ongoing research makes contrast agents safer, more effective, and more biochemically specific for precision diagnoses. On the other hand, recent developments in the field of radiomics, machine learning, and image processing algorithms are taking steps toward lessening or even eliminating the need for intravenous injection of foreign materials into the body to improve the diagnostic efficacy of MRI. Advanced machine learning algorithms based on generative adversarial networks have been developed that, when trained, provide approximately the same segmentation information from non-CE MRI of liver lesions and cardiac cine imaging without the use of contrast agents compared to the goldstandard CE-MRI.17,43 These image analysis methods can provide radiologists with the information they need to make accurate clinical assessments without expensive, potentially unsafe use of GBCAs. However, more work needs to be done to evaluate the reproducibility of radiomic data provided by such algorithms across a broader population.
Conclusion
Despite significant efforts in preclinical, translational, and clinical research to identify and develop alternative Gd-free diagnostic agents, it is most probable that the next generation of MRI contrast agents will still include Gd, suggesting that true high relaxivity macrocyclic GBCAs may be at least in the near future. Looking forward, a personalized combination of contrast enhancement and image processing techniques is likely to be used to optimize diagnosis and treatment planning while minimizing risk and cost.
References
- Li H, Meade TJ. Molecular magnetic resonance imaging with Gd(III)-based contrast agents: challenges and key advances. J Am Chem Soc. 2019;141(43):17025-17041.
- Bruder O, Schneider S, Pilz G, et al. 2015 Update on acute adverse reactions to gadolinium based contrast agents in cardiovascular MR. Large multi-national and multiethnical population experience with 37788 patients from the EuroCMR registry. J Cardiovasc Magn Reson. 2015;17:58.
- Attari H, Cao Y, Elmholdt TR, Zhao Y, Prince MR. A systematic review of 639 patients with biopsy-confirmed nephrogenic systemic fibrosis. Radiology. 2019;292(2):376-386.
- Ohashi T, Naganawa S, Iwata S, Kuno K. Age-related changes in the distribution of intravenously administered gadolinium-based contrast agents leaked into the cerebrospinal fluid in patients with suspected endolymphatic hydrops. Jpn J Radiol. 2021;39(5):433-441.
- Rasschaert M, Weller RO, Schroeder JA, Brochhausen C, Idee JM. Retention of gadolinium in brain parenchyma: pathways for speciation, access, and distribution. A critical review. J Magn Reson Imaging. 2020;52(5):1293-1305.
- Richter H, Bucker P, Martin LF, et al. Gadolinium tissue distribution in a large-animal model after a single dose of gadolinium-based contrast agents. Radiology. 2021:210553.
- Runge VM. Critical Questions Regarding Gadolinium Deposition in the Brain and Body After Injections of the Gadolinium-Based Contrast Agents, Safety, and Clinical Recommendations in Consideration of the EMA’s Pharmacovigilance and Risk Assessment Committee Recommendation for Suspension of the Marketing Authorizations for 4 Linear Agents. Invest Radiol. 2017;52(6):317-323.
- Gu L, Cao X, Mukhtar A, Wu K. Fe/Mn multilayer nanowires as dual mode T1 -T2 magnetic resonance imaging contrast agents. J Biomed Mater Res B Appl Biomater. 2021;109(4):477-485.
- Anemone A, Consolino L, Conti L, et al. Tumour acidosis evaluated in vivo by MRI-CEST pH imaging reveals breast cancer metastatic potential. Br J Cancer. 2021;124(1):207-216.
- Chen LQ, Howison CM, Jeffery JJ, Robey IF, Kuo PH, Pagel MD. Evaluations of extracellular pH within in vivo tumors using acidoCEST MRI. Magn Reson Med. 2014;72(5):1408-1417.
- Guivel-Scharen V, Sinnwell T, Wolff SD, Balaban RS. Detection of proton chemical exchange between metabolites and water in biological tissues. J Magn Reson. 1998;133(1):36-45.
- Lindeman LR, Randtke EA, High RA, Jones KM, Howison CM, Pagel MD. A comparison of exogenous and endogenous CEST MRI methods for evaluating in vivo pH. Magn Reson Med. 2018;79(5):2766-2772.
- Longo DL, Dastru W, Digilio G, et al. Iopamidol as a responsive MRI-chemical exchange saturation transfer contrast agent for pH mapping of kidneys: In vivo studies in mice at 7 T. Magn Reson Med. 2011;65(1):202-211.
- Walker-Samuel S, Ramasawmy R, Torrealdea F, et al. In vivo imaging of glucose uptake and metabolism in tumors. Nat Med. 2013;19(8):1067-1072.
- Ward KM, Aletras AH, Balaban RS. A new class of contrast agents for MRI based on proton chemical exchange dependent saturation transfer (CEST). J Magn Reson. 2000;143(1):79-87.
- Guo S, Wang X, Dai Y, et al. Enhancing the efficacy of metal-free MRI contrast agents via conjugating nitroxides onto PEGylated cross-linked poly(carboxylate ester). Adv Sci (Weinh). 2020;7(14):2000467.
- Xu C, Howey J, Ohorodnyk P, Roth M, Zhang H, Li S. Segmentation and quantification of infarction without contrast agents via spatiotemporal generative adversarial learning. Med Image Anal. 2020;59:101568.
- de Figueiredo EH, Borgonovi AF, Doring TM. Basic concepts of MR imaging, diffusion MR imaging, and diffusion tensor imaging. Magn Reson Imaging Clin N Am. 2011;19(1):1-22.
- Jadvar H, Colletti PM. Competitive advantage of PET/MRI. Eur J Radiol. 2014;83(1):84-94.
- Davenport MS. Choosing the safest gadolinium-based contrast medium for MR imaging: not so simple after all. Radiology. 2018;286(2):483-485.
- Bane O, Lee DC, Benefield B, Markl M, Carr J, Carroll TJ. A pilot study of leakage and compartmentalization of the contrast agent Ablavar. Journal of Cardiovascular Magnetic Resonance. 2013;15(1):E7.
- Guerbet. Efficacy and Safety of Gadopiclenol for Body Magnetic Resonance Imaging (MRI) (PROMISE). clinicaltrials.gov. https://clinicaltrials.gov/ct2/show/NCT03986138. Published 2019. Accessed Jul 14 2021.
- Robert P, Vives V, Grindel AL, et al. Contrast-to-dose relationship of gadopiclenol, an MRI macrocyclic gadolinium-based contrast agent, compared with gadoterate, gadobenate, and gadobutrol in a rat brain tumor model. Radiology. 2020;294(1):117-126.
- Lancelot E, Raynaud JS, Desche P. Current and future MR contrast agents: Seeking a better chemical stability and relaxivity for optimal safety and efficacy. Invest Radiol. 2020;55(9):578-588.
- Pan D, Schmieder AH, Wickline SA, Lanza GM. Manganese-based MRI contrast agents: past, present and future. Tetrahedron. 2011;67(44):8431-8444.
- Gale EM, Atanasova IP, Blasi F, Ay I, Caravan P. A manganese alternative to gadolinium for MRI contrast. J Am Chem Soc. 2015;137(49):15548-15557.
- Gale EM, Wey HY, Ramsay I, Yen YF, Sosnovik DE, Caravan P. A manganese-based alternative to gadolinium: Contrast-enhanced MR angiography, excretion, pharmacokinetics, and metabolism. Radiology. 2018;286(3):865-872.
- Erstad DJ, Ramsay IA, Jordan VC, et al. Tumor contrast enhancement and whole-body elimination of the manganese-based magnetic resonance imaging contrast agent Mn-PyC3A. Invest Radiol. 2019;54(11):697-703.
- Zhou IY, Ramsay IA, Ay I, et al. Positron emission tomography-magnetic resonance imaging pharmacokinetics, In vivo biodistribution, and whole-body elimination of Mn-PyC3A. Invest Radiol. 2021;56(4):261-270.
- Chang Y, Jin SU, Kim Y, et al. Decreased brain volumes in manganese-exposed welders. Neurotoxicology. 2013;37:182-189.
- Fortuin AS, Brüggemann R, van der Linden J, et al. Ultra-small superparamagnetic iron oxides for metastatic lymph node detection: back on the block. Wiley Interdiscip Rev Nanomed Nanobiotechnol. 2018;10(1).
- Finn JP, Nguyen KL, Han F, et al. Cardiovascular MRI with ferumoxytol. Clin Radiol. 2016;71(8):796-806.
- Varallyay CG, Toth GB, Fu R, et al. What does the boxed warning Tell Us? Safe Practice of Using Ferumoxytol as an MRI Contrast Agent. AJNR Am J Neuroradiol. 2017;38(7):1297-1302.
- Corwin MT, Fananapazir G, Chaudhari AJ. MR Angiography of Renal Transplant Vasculature with Ferumoxytol: Comparison of High-Resolution Steady-State and FirstPass Acquisitions. Acad Radiol. 2016;23(3):368-373.
- Kim J, Wu Y, Guo Y, Zheng H, Sun PZ. A review of optimization and quantification techniques for chemical exchange saturation transfer MRI toward sensitive in vivo imaging. Contrast Media Mol Imaging. 2015;10(3):163-178.
- Chen Z, Han Z, Liu G. Repurposing Clinical Agents for Chemical Exchange Saturation Transfer Magnetic Resonance Imaging: Current Status and Future Perspectives. Pharmaceuticals (Basel). 2020;14(1).
- Zhang L, Martins AF, Mai Y, et al. Imaging Extracellular Lactate In Vitro and In Vivo Using CEST MRI and a Paramagnetic Shift Reagent. Chemistry. 2017;23(8):1752-1756.
- Liu G, Banerjee SR, Yang X, et al. A dextran-based probe for the targeted magnetic resonance imaging of tumours expressing prostate-specific membrane antigen. Nat Biomed Eng. 2017;1:977-982.
- Fu C, Yu Y, Xu X, et al. Functional polymers as metal-free magnetic resonance imaging contrast agents. Progress in Polymer Science. 2020;108:101286.
- Nguyen HV, Chen Q, Paletta JT, et al. Nitroxide-Based Macromolecular Contrast Agents with Unprecedented Transverse Relaxivity and Stability for Magnetic Resonance Imaging of Tumors. ACS Cent Sci. 2017;3(7):800-811.
- Nguyen HV, Detappe A, Harvey P, et al. Pro-organic radical contrast agents (“pro-ORCAs”) for real-time MRI of pro-drug activation in biological systems. Polym Chem. 2020;11(29):4768-4779.
- Dharmarwardana M, Martins AF, Chen Z, et al. Nitroxyl Modified Tobacco Mosaic Virus as a Metal-Free High-Relaxivity MRI and EPR Active Superoxide Sensor. Mol Pharm. 2018;15(8):2973-2983.
- Xiao X, Qiang Y, Zhao J, Yang X, Yang X. Segmentation of Liver Lesions Without Contrast Agents With Radiomics-Guided Densely UNet-Nested GAN. IEEE Access. 2020;PP:1-1.
References
- Li H, Meade TJ. Molecular magnetic resonance imaging with Gd(III)-based contrast agents: challenges and key advances. J Am Chem Soc. 2019;141(43):17025-17041.
- Bruder O, Schneider S, Pilz G, et al. 2015 Update on acute adverse reactions to gadolinium based contrast agents in cardiovascular MR. Large multi-national and multiethnical population experience with 37788 patients from the EuroCMR registry. J Cardiovasc Magn Reson. 2015;17:58.
- Attari H, Cao Y, Elmholdt TR, Zhao Y, Prince MR. A systematic review of 639 patients with biopsy-confirmed nephrogenic systemic fibrosis. Radiology. 2019;292(2):376-386.
- Ohashi T, Naganawa S, Iwata S, Kuno K. Age-related changes in the distribution of intravenously administered gadolinium-based contrast agents leaked into the cerebrospinal fluid in patients with suspected endolymphatic hydrops. Jpn J Radiol. 2021;39(5):433-441.
- Rasschaert M, Weller RO, Schroeder JA, Brochhausen C, Idee JM. Retention of gadolinium in brain parenchyma: pathways for speciation, access, and distribution. A critical review. J Magn Reson Imaging. 2020;52(5):1293-1305.
- Richter H, Bucker P, Martin LF, et al. Gadolinium tissue distribution in a large-animal model after a single dose of gadolinium-based contrast agents. Radiology. 2021:210553.
- Runge VM. Critical Questions Regarding Gadolinium Deposition in the Brain and Body After Injections of the Gadolinium-Based Contrast Agents, Safety, and Clinical Recommendations in Consideration of the EMA’s Pharmacovigilance and Risk Assessment Committee Recommendation for Suspension of the Marketing Authorizations for 4 Linear Agents. Invest Radiol. 2017;52(6):317-323.
- Gu L, Cao X, Mukhtar A, Wu K. Fe/Mn multilayer nanowires as dual mode T1 -T2 magnetic resonance imaging contrast agents. J Biomed Mater Res B Appl Biomater. 2021;109(4):477-485.
- Anemone A, Consolino L, Conti L, et al. Tumour acidosis evaluated in vivo by MRI-CEST pH imaging reveals breast cancer metastatic potential. Br J Cancer. 2021;124(1):207-216.
- Chen LQ, Howison CM, Jeffery JJ, Robey IF, Kuo PH, Pagel MD. Evaluations of extracellular pH within in vivo tumors using acidoCEST MRI. Magn Reson Med. 2014;72(5):1408-1417.
- Guivel-Scharen V, Sinnwell T, Wolff SD, Balaban RS. Detection of proton chemical exchange between metabolites and water in biological tissues. J Magn Reson. 1998;133(1):36-45.
- Lindeman LR, Randtke EA, High RA, Jones KM, Howison CM, Pagel MD. A comparison of exogenous and endogenous CEST MRI methods for evaluating in vivo pH. Magn Reson Med. 2018;79(5):2766-2772.
- Longo DL, Dastru W, Digilio G, et al. Iopamidol as a responsive MRI-chemical exchange saturation transfer contrast agent for pH mapping of kidneys: In vivo studies in mice at 7 T. Magn Reson Med. 2011;65(1):202-211.
- Walker-Samuel S, Ramasawmy R, Torrealdea F, et al. In vivo imaging of glucose uptake and metabolism in tumors. Nat Med. 2013;19(8):1067-1072.
- Ward KM, Aletras AH, Balaban RS. A new class of contrast agents for MRI based on proton chemical exchange dependent saturation transfer (CEST). J Magn Reson. 2000;143(1):79-87.
- Guo S, Wang X, Dai Y, et al. Enhancing the efficacy of metal-free MRI contrast agents via conjugating nitroxides onto PEGylated cross-linked poly(carboxylate ester). Adv Sci (Weinh). 2020;7(14):2000467.
- Xu C, Howey J, Ohorodnyk P, Roth M, Zhang H, Li S. Segmentation and quantification of infarction without contrast agents via spatiotemporal generative adversarial learning. Med Image Anal. 2020;59:101568.
- de Figueiredo EH, Borgonovi AF, Doring TM. Basic concepts of MR imaging, diffusion MR imaging, and diffusion tensor imaging. Magn Reson Imaging Clin N Am. 2011;19(1):1-22.
- Jadvar H, Colletti PM. Competitive advantage of PET/MRI. Eur J Radiol. 2014;83(1):84-94.
- Davenport MS. Choosing the safest gadolinium-based contrast medium for MR imaging: not so simple after all. Radiology. 2018;286(2):483-485.
- Bane O, Lee DC, Benefield B, Markl M, Carr J, Carroll TJ. A pilot study of leakage and compartmentalization of the contrast agent Ablavar. Journal of Cardiovascular Magnetic Resonance. 2013;15(1):E7.
- Guerbet. Efficacy and Safety of Gadopiclenol for Body Magnetic Resonance Imaging (MRI) (PROMISE). clinicaltrials.gov. https://clinicaltrials.gov/ct2/show/NCT03986138. Published 2019. Accessed Jul 14 2021.
- Robert P, Vives V, Grindel AL, et al. Contrast-to-dose relationship of gadopiclenol, an MRI macrocyclic gadolinium-based contrast agent, compared with gadoterate, gadobenate, and gadobutrol in a rat brain tumor model. Radiology. 2020;294(1):117-126.
- Lancelot E, Raynaud JS, Desche P. Current and future MR contrast agents: Seeking a better chemical stability and relaxivity for optimal safety and efficacy. Invest Radiol. 2020;55(9):578-588.
- Pan D, Schmieder AH, Wickline SA, Lanza GM. Manganese-based MRI contrast agents: past, present and future. Tetrahedron. 2011;67(44):8431-8444.
- Gale EM, Atanasova IP, Blasi F, Ay I, Caravan P. A manganese alternative to gadolinium for MRI contrast. J Am Chem Soc. 2015;137(49):15548-15557.
- Gale EM, Wey HY, Ramsay I, Yen YF, Sosnovik DE, Caravan P. A manganese-based alternative to gadolinium: Contrast-enhanced MR angiography, excretion, pharmacokinetics, and metabolism. Radiology. 2018;286(3):865-872.
- Erstad DJ, Ramsay IA, Jordan VC, et al. Tumor contrast enhancement and whole-body elimination of the manganese-based magnetic resonance imaging contrast agent Mn-PyC3A. Invest Radiol. 2019;54(11):697-703.
- Zhou IY, Ramsay IA, Ay I, et al. Positron emission tomography-magnetic resonance imaging pharmacokinetics, In vivo biodistribution, and whole-body elimination of Mn-PyC3A. Invest Radiol. 2021;56(4):261-270.
- Chang Y, Jin SU, Kim Y, et al. Decreased brain volumes in manganese-exposed welders. Neurotoxicology. 2013;37:182-189.
- Fortuin AS, Brüggemann R, van der Linden J, et al. Ultra-small superparamagnetic iron oxides for metastatic lymph node detection: back on the block. Wiley Interdiscip Rev Nanomed Nanobiotechnol. 2018;10(1).
- Finn JP, Nguyen KL, Han F, et al. Cardiovascular MRI with ferumoxytol. Clin Radiol. 2016;71(8):796-806.
- Varallyay CG, Toth GB, Fu R, et al. What does the boxed warning Tell Us? Safe Practice of Using Ferumoxytol as an MRI Contrast Agent. AJNR Am J Neuroradiol. 2017;38(7):1297-1302.
- Corwin MT, Fananapazir G, Chaudhari AJ. MR Angiography of Renal Transplant Vasculature with Ferumoxytol: Comparison of High-Resolution Steady-State and FirstPass Acquisitions. Acad Radiol. 2016;23(3):368-373.
- Kim J, Wu Y, Guo Y, Zheng H, Sun PZ. A review of optimization and quantification techniques for chemical exchange saturation transfer MRI toward sensitive in vivo imaging. Contrast Media Mol Imaging. 2015;10(3):163-178.
- Chen Z, Han Z, Liu G. Repurposing Clinical Agents for Chemical Exchange Saturation Transfer Magnetic Resonance Imaging: Current Status and Future Perspectives. Pharmaceuticals (Basel). 2020;14(1).
- Zhang L, Martins AF, Mai Y, et al. Imaging Extracellular Lactate In Vitro and In Vivo Using CEST MRI and a Paramagnetic Shift Reagent. Chemistry. 2017;23(8):1752-1756.
- Liu G, Banerjee SR, Yang X, et al. A dextran-based probe for the targeted magnetic resonance imaging of tumours expressing prostate-specific membrane antigen. Nat Biomed Eng. 2017;1:977-982.
- Fu C, Yu Y, Xu X, et al. Functional polymers as metal-free magnetic resonance imaging contrast agents. Progress in Polymer Science. 2020;108:101286.
- Nguyen HV, Chen Q, Paletta JT, et al. Nitroxide-Based Macromolecular Contrast Agents with Unprecedented Transverse Relaxivity and Stability for Magnetic Resonance Imaging of Tumors. ACS Cent Sci. 2017;3(7):800-811.
- Nguyen HV, Detappe A, Harvey P, et al. Pro-organic radical contrast agents (“pro-ORCAs”) for real-time MRI of pro-drug activation in biological systems. Polym Chem. 2020;11(29):4768-4779.
- Dharmarwardana M, Martins AF, Chen Z, et al. Nitroxyl Modified Tobacco Mosaic Virus as a Metal-Free High-Relaxivity MRI and EPR Active Superoxide Sensor. Mol Pharm. 2018;15(8):2973-2983.
- Xiao X, Qiang Y, Zhao J, Yang X, Yang X. Segmentation of Liver Lesions Without Contrast Agents With Radiomics-Guided Densely UNet-Nested GAN. IEEE Access. 2020;PP:1-1.
Affiliation: University of Alabama at Birmingham School of Medicine, Birmingham, Alabama. Disclosure: Dr Porter is a member of the Bracco Diagnostics Inc. COVID-19 Healthcare Professional Advisory Board (2020) and a stockholder of Pfizer Inc.