Musculoskeletal imaging in children: What the general radiologist should know
Images
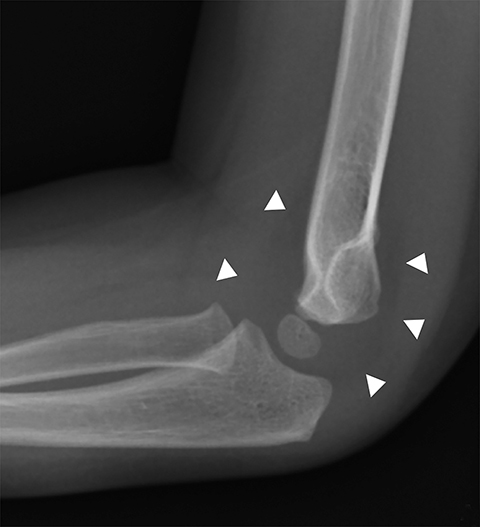
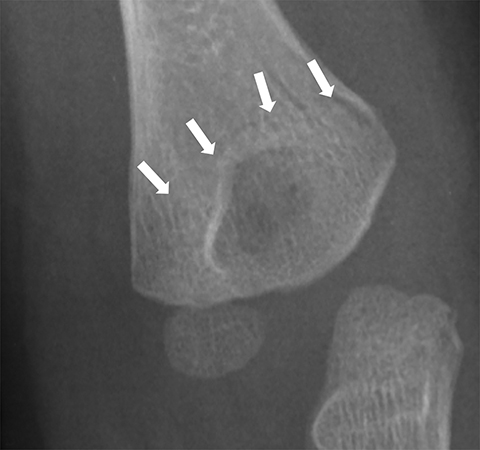
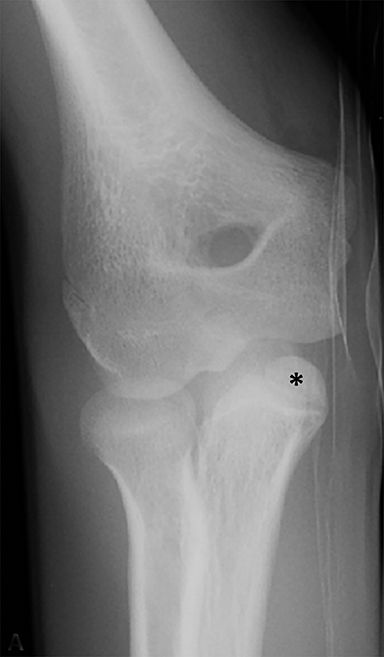
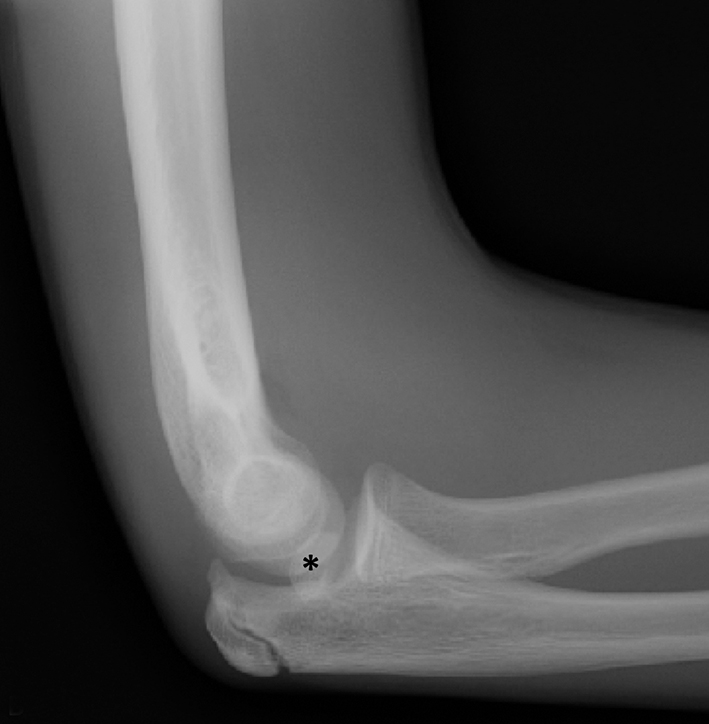
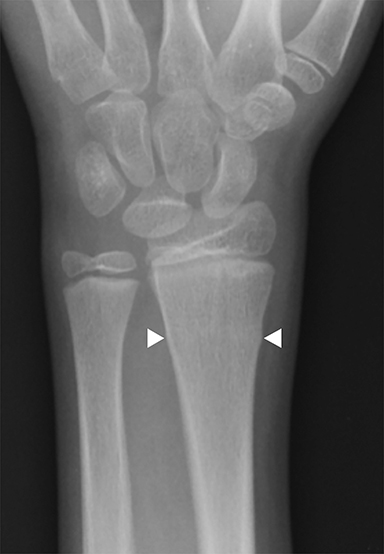
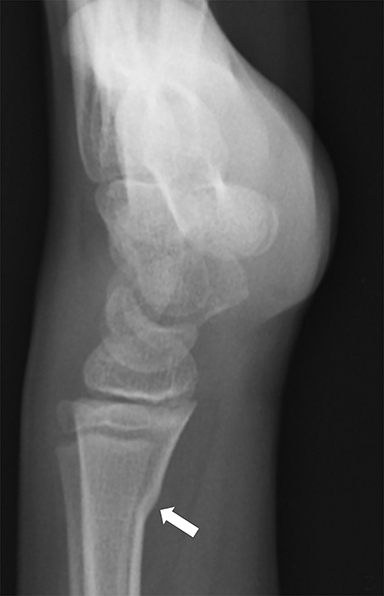
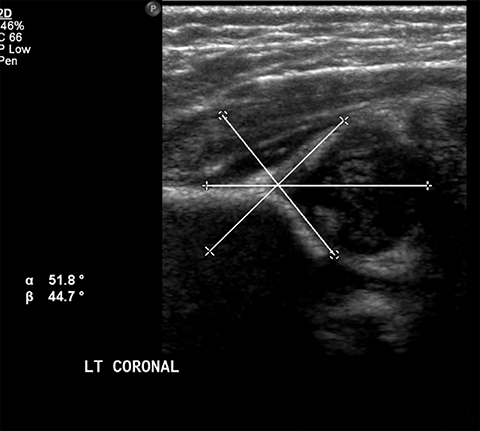
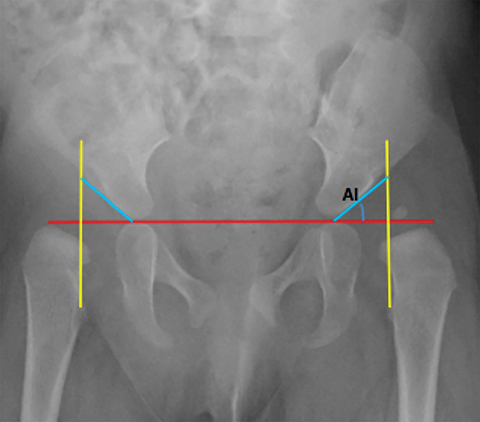
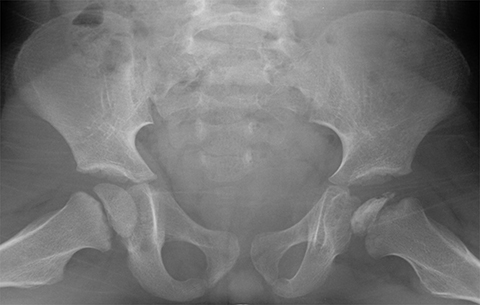
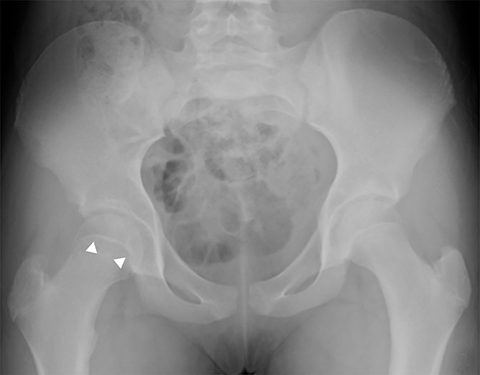
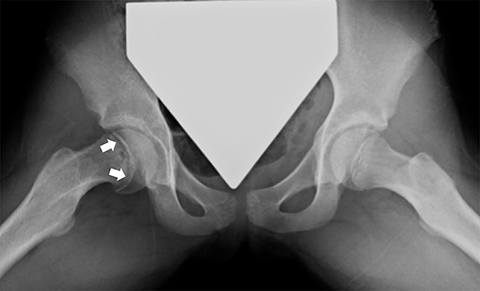
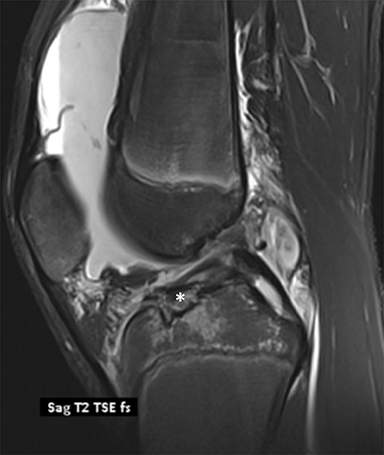
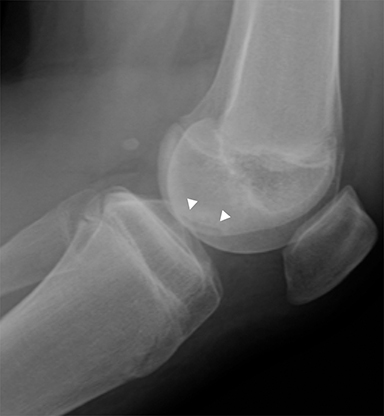
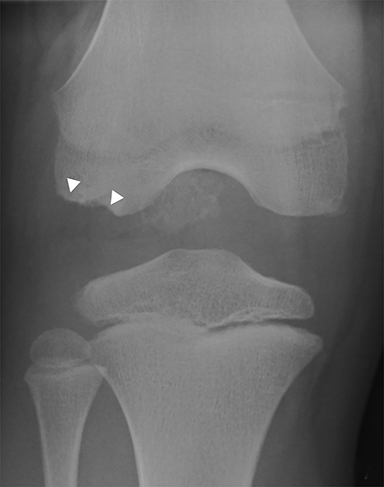
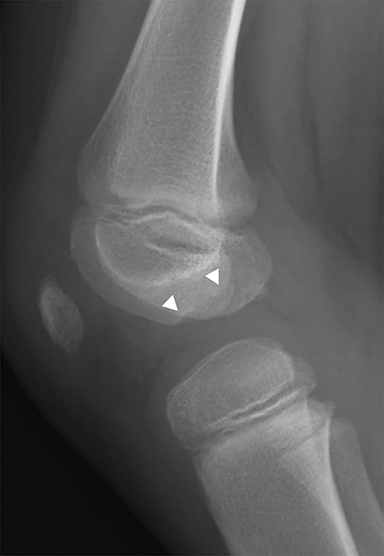
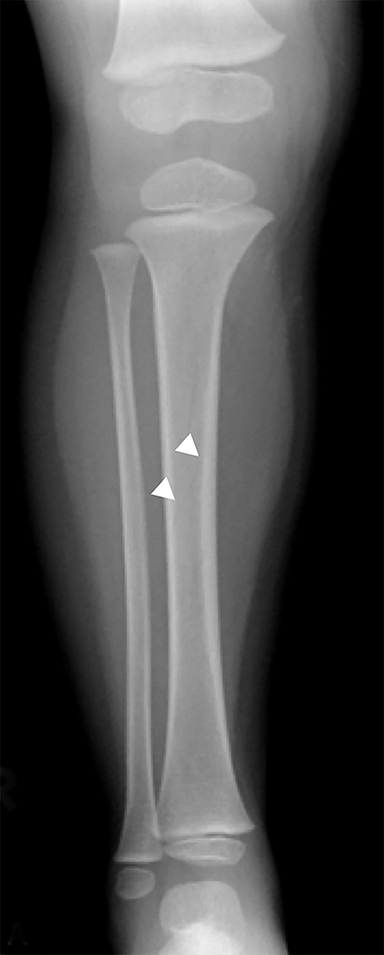
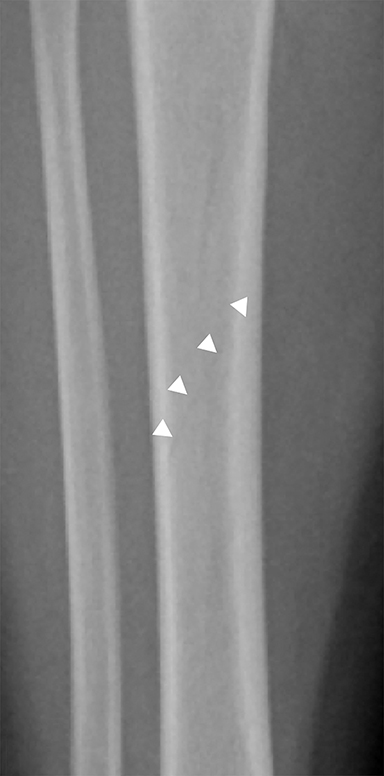
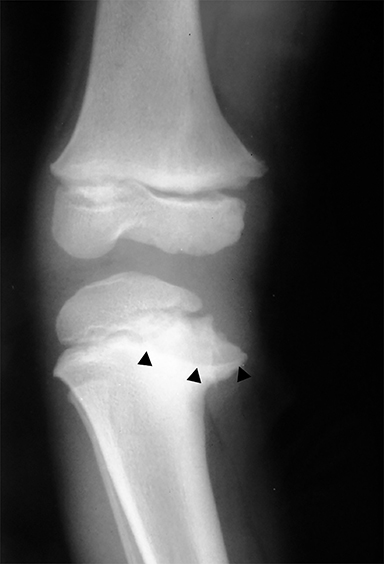
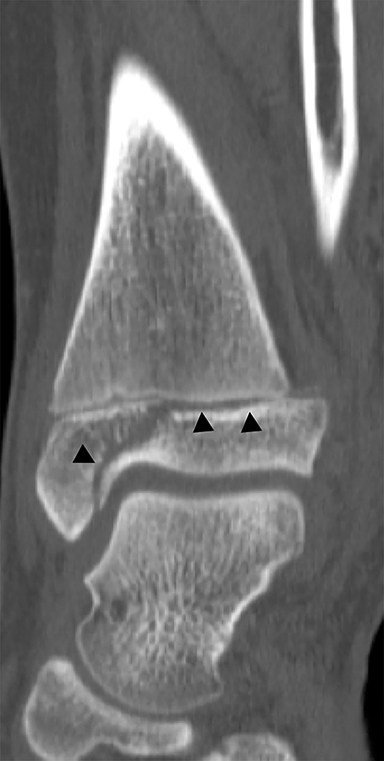
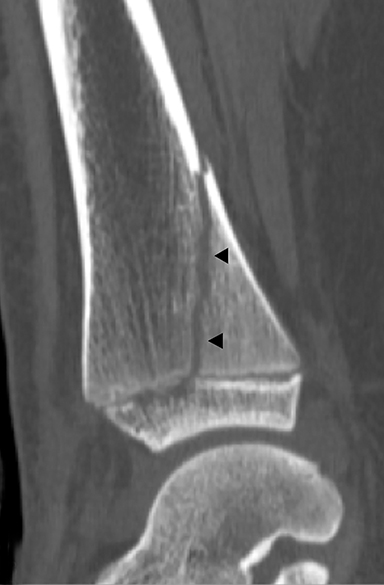
This article is accredited for one SA-CME credit. Visit appliedradiology.org/SAM2 for full SA-CME information.
Radiographs are the mainstay of pediatric musculoskeletal imaging, making up the majority of imaging exams due to their low cost and minimal risk with regard to radiation exposure.1,2 Many pediatric fractures, particularly toddlers’ fractures and buckle fractures, are only seen on one view, rendering orthogonal projections essential. Computed tomography (CT) is best used for detailed fracture analysis to help guide management. Magnetic resonance imaging (MRI) often provides complementary information for many different pathologies, but may require sedation, particularly in patients 6 months to 7 years of age. In this article, we review common pediatric musculoskeletal radiology abnormalities the radiologist may be tasked with assessing, using an anatomic approach.
Salter-Harris classification of pediatric fractures
In general, pediatric joint capsules and ligaments tend to be stronger than the physis, making physeal injuries incredibly common and the mechanical equivalent to ligamentous injuries in adults.3 Reviewing the Salter-Harris classification scheme, which is utilized to describe and classify fractures involving the physis, is useful. A Salter-Harris I fracture passes all the way through the physis without involving the metaphysis or epiphysis and is often initially occult on radiographs. Salter-Harris II fractures involve the physis and part of the metaphysis and are the most common type of physeal injury.4,5 Salter-Harris III fractures involve the physis and epiphysis. Salter-Harris IV fractures involve the physis, epiphysis and metaphysis and have more complications due to the intraarticular extension. Salter-Harris V fractures are rare crush injuries to the physis and are often occult on radiographs. Type I-III fractures tend to do well with conservative or surgical treatment, though prognosis is worse in the lower extremities. Type IV and V injuries tend to cause premature fusion across the growth plate (“physeal bar”) that can result in limb length discrepancy and angulation.3–5
Upper extremity
Elbow
In order to diagnose pediatric elbow fractures, it is essential to know the order in which the ossification centers about the elbow first appear. The mnemonic “CRITOE” (Capitellum, Radial head, Internal (medial) epicondyle, Trochlea, Olecranon and External (lateral) epicondyle) can help diagnose certain fractures, particularly those where an ossification center ‘appears’ out of the expected order. The ossification centers can look very irregular and fragmented as they are developing, particularly the olecranon and trochlea. It is important to note that the fusion of these ossification centers may not take place in this same order in which they appear.
The most common type of pediatric elbow fracture is the supracondylar fracture (Figure 1), accounting for 50-75% of all elbow fractures. It is typically seen in children 3-10 years old and occurs in FOOSH (fall on outstretched hand) injuries where the olecranon acts as the fulcrum. Approximately 10-20% of these fractures present with neurovascular compromise. The fracture line is not always evident, making alignment, as evaluated with the anterior humeral line and the radiocapitellar line, important. The anterior humeral line, drawn along the anterior humeral diaphyseal cortex, should normally bisect the middle third of the capitellum in children older than 2.5 years.6 The radiocapitellar line, a line through the radial head and neck, should normally bisect the capitellum on all views.6 The presence of an elbow effusion is also helpful in detecting radiographically occult fractures, and if clinical concern warrants, immobilization and repeat radiographs in 10-14 days may be useful.
Lateral condylar fractures are the second most common elbow fracture in children, accounting for 10-15% of fractures, typically occurring in children ages 6-10 years with a varus force on a supinated elbow. This fracture is intra-articular and displacement is important to recognize, specifically because it is often treated with open reduction and pinning. The mnemonic “CRITOE” is helpful in detecting this type of fracture, as the lateral epicondyle is the last ossification center to appear, and if seen before any of the other ossification centers, concern for a fracture is raised.
Medial epicondyle avulsion fractures account for approximately 10% of pediatric elbow fractures, usually ages 7-15 years.6 They are caused by valgus stress on the elbow and associated with elbow dislocations in one third to one half of cases. The mnemonic “CRITOE” is particularly helpful in diagnosing this type of elbow fracture, as the avulsed medial epicondyle may mimic the developing trochlea. However, based on “CRITOE”, one would expect appearance of the medial epicondyle (in normal position) prior to the appearance of the trochlear ossification center (Figure 2).
Forearm/wrist
Buckle (torus) fractures are incredibly common in children 2-12 years and are fractures in which one cortex is compressed while the opposite tension side of the cortex remains intact (Figure 3).7,8 There are two types of buckle fractures: (1) the classic buckle fracture where there is an outward cortical bulge and (2) the angled buckle fracture in which the cortex is angled but there is no outward bulge.9,10 Buckle fractures typically heal well with immobilization and account for 50% of pediatric fractures of the wrist.8,11
Plastic bending fractures occur because pediatric bones are soft, and an axial load will first cause the bone to bend. No fracture line is evident with a bending fracture. They most commonly occur in the forearm but can also affect the clavicle and the fibula.9 If the force is great enough, it can ultimately cause the cortex to break along the tension side of the fracture while the cortex on the compression side remains intact, which is termed the greenstick fracture.8,10 In contrast to buckle fractures, greenstick fractures tend to be unstable and can displace even after the first two weeks.8
Gymnast wrist is a pediatric entity demonstrating widening of the growth plate with irregularity and sclerosis of the metaphysis.10 It is caused by repetitive compressive loading and shearing forces that result in physeal microfractures, injury to the vascular supply, and ultimately to a chronic overuse Salter-Harris I fracture. A physeal bar may form that can lead to growth disturbance, positive ulnar variance, and ligamentous and carpal disturbances.8 Gymnast wrist is also known as “pseudo rickets” growth plate abnormality or distal radial epiphysitis and commonly affects 10-14 year olds involved in weight lifting as well.12
Lower extremity
Hip
Developmental dysplasia of the hip (DDH) encompasses a wide range of pediatric hip disorders and is characterized as clinical instability with or without anatomic dysplasia, subluxation or dislocation.13 Risk factors include female sex, breech positioning at ≥ 34 weeks gestation, family history of DDH and tight lower extremity swaddling.14 Ultrasound is the preferred imaging modality in infants because of a very high sensitivity, but it can lead to overdiagnosis.15 Ultrasound is usually only useful between 2-4 weeks and 4-6 months of age because of increased laxity in the setting of maternal hormones shortly after birth and progressive ossification of the femoral head around 6 months of age.13 It is generally performed at 4 to 6 weeks of age (adjusted for prematurity) for infants who were breech at ≥ 34 weeks gestation, have a family history of DDH or demonstrate clinical instability on physical exam.14 On ultrasound, the main imaging findings to look for in a normal hip include greater than 50% coverage of the femoral head by the acetabulum, an alpha angle greater than 60 degrees and absence of subluxation or dislocation with stress maneuvers (Figure 4). After the age of 6 months, radiographs are the best modality in evaluation of DDH, and can be used to evaluate the acetabular angle, or the angle between Hilgenreiner’s line (horizontal line connecting the triradiate cartilages) and a line drawn along the acetabular roof (Figure 4). The normal acetabular angle changes with age but should be less than 30 degrees in a newborn and approximately 20 degrees at age 2. Shenton’s line is another line that can be used for radiographic evaluation of DDH and is an arc along the inferior border of the superior pubic ramus and the inferomedial border of the femoral neck. This arc should be continuous and when disrupted, is suggestive of DDH.13
Legg-Calve-Perthes is idiopathic osteonecrosis of the immature femoral head and is a diagnosis of exclusion after considering other causes of pediatric avascular necrosis including sickle cell disease, steroids, and prior trauma. It commonly occurs in children 5-6 years old, is 5 times more common in boys and unilateral in 85-90% of cases.13 Early radiographic findings include hip effusion and lateral subluxation, fragmentation, sclerosis and flattening of the femoral epiphysis (Figure 5). This can progress to lateral extrusion of a portion of the femoral head and metaphyseal irregularity with underlying cystic change. Late radiographic findings include coxa plana, coxa magna and growth disturbances. Additional findings on MR include decreased or absent enhancement, low T1 signal of the subchondral bone, and thickening of the cartilage overlying the flattened femoral head. MR is also useful in defining the percentage of femoral head involvement, which is the basis of the Catterall classification system and one of the most important factors in predicting outcome.16,17 Approximately 60-70% of cases heal spontaneously without functional impairment, but it can lead to labral and cartilage damage as well as osteoarthritis.
Slipped Capital Femoral Epiphysis (SCFE) is a Salter-Harris type I fracture through the proximal femoral physis caused by shear stress. It most commonly affects overweight children, girls 11-12 years old and boys 13-14 years old. It is seen more commonly in males (2.5:1) and is bilateral in approximately one-third of cases.18 Radiographs are the mainstay of diagnosis, with the frog-lateral being more sensitive than AP (Figure 6). Findings include mild widening and irregularity of the physis in the most subtle of cases and slippage of the epiphysis posteromedially in later stages. Klein’s line, a line drawn parallel to the lateral femoral neck, can be helpful in diagnosing SCFE in that it should intersect the femoral epiphysis in a normal hip but often does not with SCFE. MR may be helpful in early cases, showing physeal widening and periphyseal marrow edema prior to the femoral head slipping. SCFE is treated surgically with in situ pinning due to the risk of increased displacement and avascular necrosis. Trying to realign the femoral head to a normal position prior to pinning increases the risk of avascular necrosis, so is usually reserved for severe cases.18 Other complications include femoral acetabular impingement and chondrolysis, for which MR is often useful in detecting.
Knee
Anterior cruciate ligament (ACL) avulsion fractures are seen in children prior to physeal closure and occur because the tibial spine is relatively weaker than the ligament.19 The actual anterior cruciate ligament is typically intact. These fractures can be difficult to recognize, but there is usually a small bone fragment in the intercondylar notch and irregularity of the tibial spine, suggestive of a donor site (Figure 7).20 The Meyers and McKeever classification system is used to describe the degree of displacement of the tibial spine and is important because it drives management. A type I injury describes minimal displacement of the tibial spine and is treated conservatively, while type II injuries demonstrate elevation of the anterior aspect of the tibial spine (hinge appearance) and usually require arthroscopy. Type III and IV injuries have complete separation from the tibial spine and are treated with internal fixation.20 Fortunately, surgical repair is usually straightforward and effective.19
Chondral and osteochondral lesions are incredibly common injuries, particularly in children with open physes.21 In the knee, the location of chondral injury varies depending on skeletal maturity. Before physeal closure, femoral chondral injury is most common, while patellar chondral injury is more common after physeal closure.21 The importance of these injuries is that it predisposes to early osteoarthritis and can result in loose fragments that can cause intermittent locking and pain.19 Osteochondritis dissecans is a term describing the dislodgement (partial or completely) from the articular surface due to ischemia. It is an acquired condition that may be caused by acute trauma, repetitive microtrauma, mechanical stress and/or avascular necrosis. It is most commonly seen in the weight bearing portion of the medial femoral condyle.19 Radiographically, it appears as a focal area of lucency involving the subchondral bone with a varying amount of surrounding sclerosis (Figure 8). The fragment of bone can be within the defect or can be displaced. MRI is useful in evaluating for stability of the osseous fragment. Imaging characteristics that suggest instability include T2 bright fluid surrounding the fragment, disruption of the subchondral bone and a 5mm or larger cyst adjacent to the lesion.19 An unstable or displaced fragment is managed surgically. Small, stable lesions and open physes are associated with better outcomes.22
One important entity to distinguish from osteochondritis dessicans is normal irregularity of the ossification centers. Irregular ossification typically affects the posterolateral femoral condyle and usually occurs in children less than 10 years old, although there is slight variation depending on sex.23 Radiographically, the normal variation in ossification appears as an irregular subchondral bone segment but lacks underlying bone marrow edema, overlying cartilage abnormalities, and intraarticular osteochondral free fragments (Figure9).19
Osgood-Schlatter disease is a chronic avulsion or overuse injury involving the patellar tendon insertion on the tibial tubercle, that results in inflammation, reactive bone formation, marrow edema, patellar tendon thickening and soft tissue swelling. Fragmentation of the tibial tubercle on pediatric knee radiographs is commonly seen with Osgood-Schlatter disease but may also be a normal variant. Only when there are clinical symptoms or additional radiographic findings such as patellar tendon thickening or soft tissue swelling is the diagnosis invoked.23 Osgood-Schlatter disease is bilateral in 25-50% of cases and typically affects young teenagers as the tubercle matures.19 Although it is a clinical diagnosis, MRI can be helpful in evaluating for marrow edema in very early cases.
Sinding-Larsen-Johansson syndrome is a conceptually similar entity to Osgood-Schlatter’s disease that affects a similar age group and is a result of chronic stress or overuse injury of the patellar tendon. The injury affects the patellar tendon at the attachment on the inferior pole of the patella rather than at the tibial tubercle. Radiographic findings are similar, with soft tissue swelling, inferior patellar fragmentation, patellar tendon thickening and possible tendon calcification/ossification. MRI shows all of these findings, along with edema within the inferior patella and in the patellar tendon.24
Patellar sleeve fractures are also unique to the pediatric population and are characterized by an avulsion of inferior patellar cartilage. The injury is most commonly seen in 8-12 year olds and occurs with vigorous contraction of the quadriceps. The fracture is frequently accompanied by an avulsed piece of bone. Radiographic findings include a small fragment of bone at the inferior pole of the patella, patella alta, soft tissue swelling and joint effusion. Radiographs often underestimate the degree of cartilage involvement, and for this reason, MRI is preferred.19,24
Tibia and fibula
Toddler’s fractures occur most commonly in the tibial diaphysis, and are typically hairline, nondisplaced, oblique fractures. They can be incredibly subtle and may only be seen on one view (Figure 10). These fractures can occur with relatively minor injuries, namely a twisting or torque force applied to the tibia during an accidental fall. Parents often do not report a history of trauma, and children (ages 9 months–3 years) present with a new onset limp or refusal to bear weight.25 It is important to note that this type of fracture is only seen in children who are ambulatory, and if a similar fracture is seen in a young, non-ambulatory infant, non-accidental trauma should be considered.26 Toddler’s fractures can also occur in the cuboid and the calcaneus, and are often radiographically occult on initial imaging.27,28
Blount disease, also known as tibia vara, is caused by abnormal endochondral ossification of the medial aspect of the proximal tibial physis. There are 3 different types: infantile (onset ≤ 4 years old), juvenile (4-10 years old), and late onset (≥ 10 years). The infantile variant is the most common and often can be treated with bracing. Juvenile and late onset variants may represent unrecognized or untreated infantile forms and are usually treated with valgus osteotomies.29 This difference in management makes early recognition and distinction from physiologic bowing critical. Blount disease is seen most commonly in early walkers, overweight children and African-Americans.29 The infantile variant is frequently bilateral.30–34 Radiographic findings include down sloping and irregularity of the medial tibial metaphysis, physeal widening, beaking of the medial metaphysis and internal rotation of the tibia (Figure 11). The metaphyseal-diaphyseal angle of the tibia, the angle between a line parallel to the top of the proximal tibial metaphysis and a line perpendicular to the tibial diaphysis, should measure greater than 11 degrees in Blount disease.29,30
Ankle
There are two unique ankle fracture types seen in children during an 18-month transitional period in which the physis is fusing: the juvenile Tillaux and triplane fractures. The physis typically first fuses centrally beginning at Kump’s bump, then anteromedially, posteromedially and finally laterally. The unfused portions of the physis are particularly prone to injury.3,35 Both of these fractures typically affect children ages 12-15 years.3,36
A juvenile Tillaux fracture is a type of Salter Harris III fracture in which there is an avulsion of the anterolateral distal tibial epiphysis at the insertion of the anterior tibiofibular ligament.37 The fracture is caused by a supination-eversion or external rotation injury. CT is helpful to characterize displacement of the epiphysis, as greater than 2 mm of displacement or 1 mm of translation requires open reduction and internal fixation due to increased risk of early degenerative changes. Patients with nondisplaced Tillaux fractures can be treated conservatively. Long term outcomes of treated Tillaux fractures are good, as there is minimal to no residual growth potential.3
The triplane fracture is a type of Salter-Harris IV injury that is caused by an external rotation force on a supinated foot. While there are many variants, the classic triplane fracture is characterized by a sagittal fracture through the epiphysis, an axial fracture through the physis and a coronal fracture through the distal tibial metaphysis (Figure 12). Similar to the Tillaux fracture, CT is helpful in guiding management. Fractures with less than 2 mm of displacement can be managed with immobilization. Fractures displaced by more than 2 mm are typically managed with open reduction and internal fixation because of higher rates of degenerative changes and discomfort without surgical intervention.3,36 Similar to the juvenile Tillaux fracture, triplane fractures rarely result in growth arrest.36
Conclusion
Pediatric patients manifest many unique skeletal injuries. Similar mechanisms of injury may present differently depending on skeletal maturity, with many injuries distinctly different than those seen in adults. Knowledge of these unique pathologies and anatomic variants will help the radiologist recognize these entities sooner, ultimately helping facilitate the most expeditious and appropriate care.
References
- World Health Organization. Communicating Radiation Risks in Paediatric Imaging: Information to Support Healthcare Discussions about Benefit and Risk.; 2016. http://apps.who.int/iris/bitstream/10665/205033/1/9789241510349_eng.pdf?ua=1.
- Radiological Society of North America (RSNA), American College of Radiology (ACR). Patient Safety - Radiation Dose in X-Ray and CT Exams. https://www.radiologyinfo.org/en/info.cfm?pg=safety-xray. Accessed February 27, 2018.
- Wuerz TH, Gurd DP. Pediatric physeal ankle fracture. J Am Acad Orthop Surg. 2013;21(4):234-244.
- Andrew Sonin DGB. Physeal Injury (Salter-Harris Fracture). STATdx. https://my.statdx.com/document/physeal-injury-salter-harris-fract-/be07596a-490d-41a3-980d-f428937443b2? searchTerm=Salter-Harris%20Fractures,%20Ankle. Accessed December 12, 2017.
- Rogers LF, Poznanski AK. Imaging of epiphyseal injuries. Radiology. 1994;191(2):297-308.
- Iyer RS, Thapa MM, Khanna PC, et al. Pediatric bone imaging: imaging elbow trauma in children--a review of acute and chronic injuries. AJR Am J Roentgenol. 2012;198(5):1053-1068.
- Primavesi R. Sticks and stones and broken bones: distal radius fractures in children. Can Fam Physician. 2011;57(1):45-46.
- Little JT, Klionsky NB, Chaturvedi A, et al. Pediatric distal forearm and wrist injury: an imaging review. Radiographics. 2014;34(2):472-490.
- Swischuk LE, Hernandez JA. Frequently missed fractures in children (value of comparative views). Emerg Radiol. 2004;11(1):22-28.
- Swischuk LE. What is different in children? Semin Musculoskelet Radiol. 2013;17(4):359-370.
- Ben-Yakov M, Boutis K. Buckle fractures of the distal radius in children. CMAJ. 2016;188(7):527.
- Paz DA, Chang GH, Yetto JM Jr, et al. Upper extremity overuse injuries in pediatric athletes: clinical presentation, imaging findings, and treatment. Clin Imaging. 2015;39(6):954-964.
- Zucker EJ, Lee EY, Restrepo R, et al. Hip disorders in children. AJR Am J Roentgenol. 2013;201(6):W776-W796.
- Rosenfeld SB. Developmental dysplasia of the hip: Clinical features and diagnosis. UpToDate. https://www.uptodate.com/contents/developmental-dysplasia-of-the-hip-clinical-features-and-diagnosis?sectionName=Plain%20radiographs&anchor=H16&source=see_link. Published July 13, 2017. Accessed January 9, 2018.
- Peled E, Eidelman M, Katzman A, et al. Neonatal incidence of hip dysplasia: ten years of experience. Clin Orthop Relat Res. 2008;466(4):771-775.
- Heesakkers N, van Kempen R, Feith R, et al. The long-term prognosis of Legg-Calvé-Perthes disease: a historical prospective study with a median follow-up of forty one years. Int Orthop. 2015;39(5):859-863.
- Manaster BJ. Legg-Calvé-Perthes Disease. STATdx. https://my.statdx.com/document/legg-calv-perthes-disease/c63e3da7-0d62-4cc9-bae8-42f715fc32a4?searchTerm=perthes. Accessed January 9, 2018.
- Manaster BJ. Slipped Capital Femoral Epiphysis. STATdx. https://my.statdx.com/document/slipped-capital-femoral-epiphysis/e62419c7-a839-4212-95a1-15ab44d308b6?searchTerm=scfe. Accessed January 9, 2018.
- Pai DR, Strouse PJ. MRI of the pediatric knee. AJR Am J Roentgenol. 2011;196(5):1019-1027.
- Gottsegen CJ, Eyer BA, White EA, et al. Avulsion fractures of the knee: imaging findings and clinical significance. Radiographics. 2008;28(6):1755-1770.
- Oeppen RS, Connolly SA, Bencardino JT, et al. Acute injury of the articular cartilage and subchondral bone: a common but unrecognized lesion in the immature knee. AJR Am J Roentgenol. 2004;182(1):111-117.
- De Smet AA, Ilahi OA, Graf BK. Untreated osteochondritis dissecans of the femoral condyles: prediction of patient outcome using radiographic and MR findings. Skeletal Radiol. 1997;26(8):463-467.
- Jans LBO, Jaremko JL, Ditchfield M, et al. Evolution of femoral condylar ossification at MR imaging: frequency and patient age distribution. Radiology. 2011;258(3):880-888.
- Dupuis CS, Westra SJ, Makris J, et al. Injuries and conditions of the extensor mechanism of the pediatric knee. Radiographics. 2009;29(3):877-886.
- John SD, Moorthy CS, Swischuk LE. Expanding the concept of the toddler’s fracture. Radiographics. 1997;17(2):367-376.
- Toddler’s fracture images, diagnosis, treatment options, answer review — Pediatric Radiology. https://www.pedsradiology.com/Historyanswer.aspx?qid=33&fid=1. Accessed January 31, 2018.
- Starshak RJ, Simons GW, Sty JR. Occult fracture of the calcaneus — another toddler’s fracture. Pediatr Radiol. 1984;14(1):37-40.
- Blumberg K, Patterson RJ. The toddler’s cuboid fracture. Radiology. 1991;179(1):93-94.
- Cheema JI, Grissom LE, Harcke HT. Radiographic characteristics of lower-extremity bowing in children. Radiographics. 2003;23(4):871-880.
- Coley BD. Caffey’s Pediatric Diagnostic Imaging. Philadelphia: Elsevier Saunders; 2013.
- Souder C. Infantile Blount’s Disease (tibia vara). Orthobullets. https://www.orthobullets.com/pediatrics/4050/infantile-blounts-disease-tibia-vara. Accessed February 27, 2018.
- Blount Disease: Background, Anatomy, Pathophysiology. https://emedicine.medscape.com/article/1250420-overview. Published October 30, 2017. Accessed February 27, 2018.
- Mooney JF, Murphy RF. Asynchronous onset of bilateral Blount’s Disease. Ped Health Res. 2017;2:1. Doi: 10.2176/2574-2817.100005
- Gill KG, Nemeth BA, Davis KW. Magnetic resonance imaging of the pediatric knee. Magn Reson Imaging Clin N Am. 2014;22(4):743-763.
- Crawford AH. Triplane and Tillaux fractures: is a 2 mm residual gap acceptable? J Pediatr Orthop. 2012;32 Suppl 1:S69-S73.
- Schnetzler KA, Hoernschemeyer D. The pediatric triplane ankle fracture. J Am Acad Orthop Surg. 2007;15(12):738-747.
- Tanaka M, Shibano K, Tagawa Y, et al. Juvenile Tillaux fracture with disrupted anteroinferior tibiofibular ligament: A case report. Knee Surg Sports Traumatol Arthrosc. 2009;17(10):1239-1242.
Citation
Ngo JS, Schooler GR. Musculoskeletal imaging in children: What the general radiologist should know. Appl Radiol. 2019;(2):7-14.
doi:https://www.appliedradiology.com/articles/musculoskeletal-imaging-in-children-what-the-general-radiologist-should-know
March 28, 2019