Enhancing Patient Safety in Today’s Healthcare Environment: Radiation Dose and Contrast Optimization in CT Imaging
Images
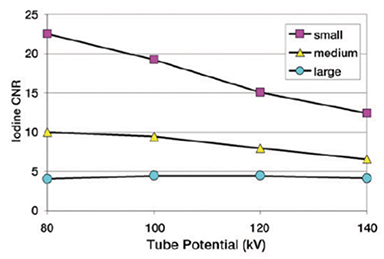
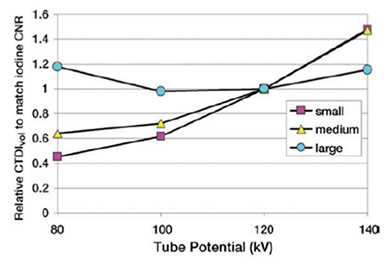
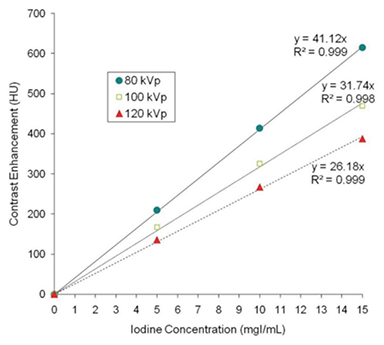
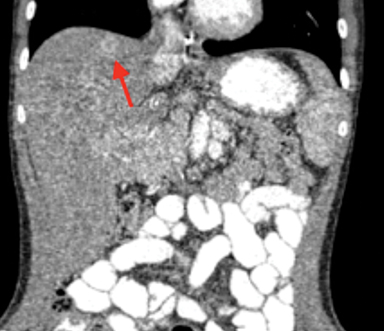
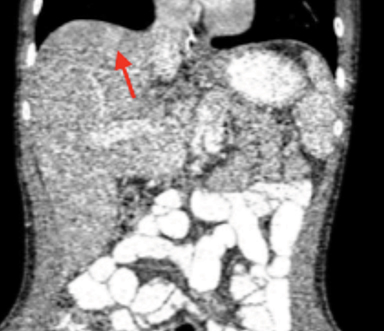
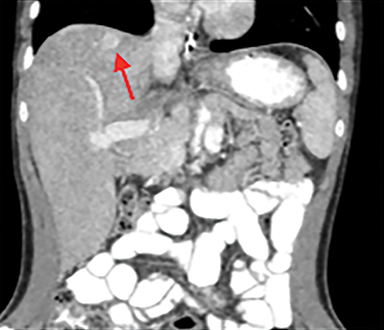
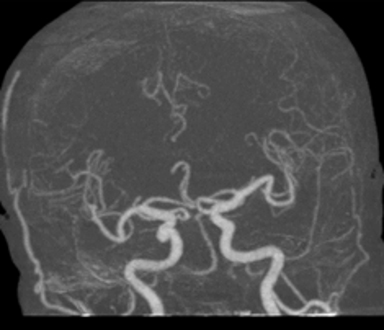
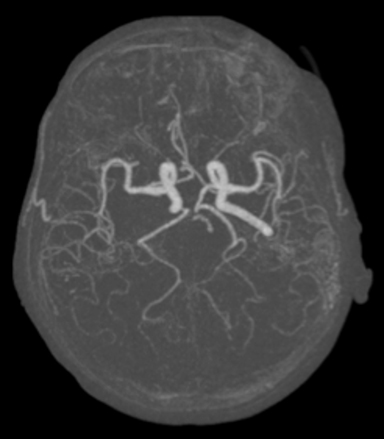
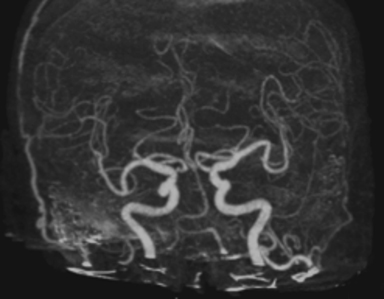
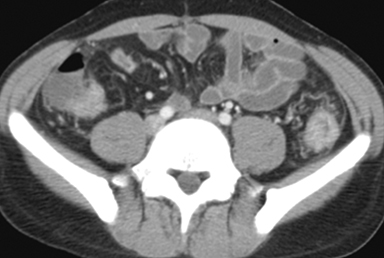
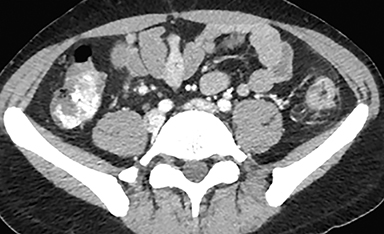
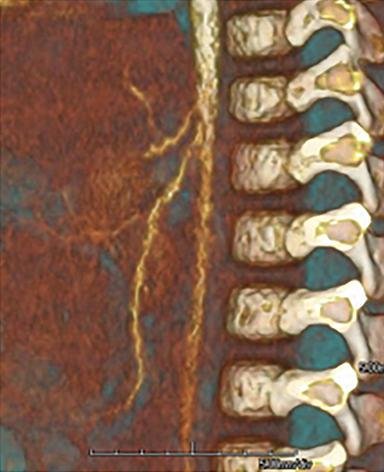
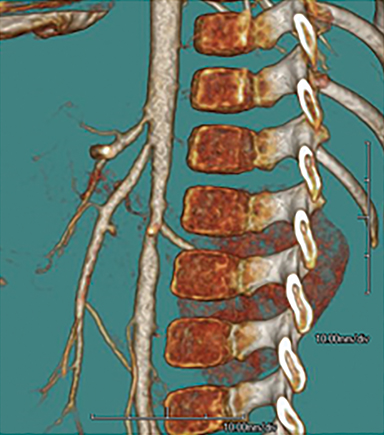
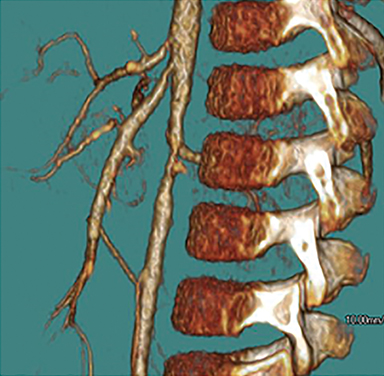
In the past 2 decades, the number of computed tomography (CT) exams performed in the United States has increased from approximately 20 million to more than 70 million per year, including 5 million pediatric CT exams. 1 In addition, a comparison of the sources of all radiation exposure to the US population between 1982 and 2006 showed an increase in the contribution of medical radiation from 15% to over 50% of the total. 2 This increase in radiation exposure from medical sources, particularly multidetector CT (MDCT), has fueled efforts by the radiology community and CT scanner manufacturers to create and implement both hardware and software solutions that reduce radiation exposure.
Keeping radiation dose ALARA — “As Low as Reasonably Achievable” — is the aim for those responsible for CT protocol design, keeping in mind that the potential risk must be balanced with the expected benefit of diagnostic information provided. In this sense, best clinical practice is to focus on dose optimization, rather than absolute radiation reduction. The clinical indication, imaging goals, and individual patient characteristics need to be taken into account when designing acquisition and contrast administration protocols, in order to obtain ideal diagnostic images at the appropriate radiation dose. Here we review general considerations for CT radiation dose optimization and, in addition, provide some detailed strategies specific to neuro, body, and cardiovascular CT (CVCT) imaging.
General CT Radiation Dose Optimization Strategies
CT Acquisition Parameters
The appropriate choice of CT acquisition parameters requires an understanding of the interaction of tube current (mA) and tube voltage (kV), and their effect on patient dose, image noise, and low-contrast resolution. Tube current (mA) relates to the intensity of the X-ray beam, and it varies linearly with radiation dose: if the mA is reduced by 30%, there will be a corresponding 30% reduction in radiation dose. Tube current is typically automatically adjusted during the examination to maintain operator-designated noise levels. These auto-exposure techniques (Table 1) modify the tube current along the xyz axis, increasing it where the patient diameter/density is higher and decreasing it where it is lower (tube current modulation).
Tube voltage (kV), or tube potential, relates to the energy of the X-ray beam. In contrast to what occurs with mA, radiation dose varies approximately as the square of tube voltage. As a result, small reductions in kV have a more substantial effect on radiation dose reduction. kV values remain constant throughout an exam phase, but can vary per pass on a multiphase exam. Interestingly, lower kV imaging is associated with higher image contrast, and this effect has been demonstrated to be greater in small- and medium-sized patients (Figure 1). Therefore, it is widely recommended that the standard 120 kV and higher 140 kV be reserved for larger patients, and that 100 kV, 80 kV, or even 70 kV, depending on the clinical indication and equipment available, be used for average and smaller-than-average patients. Software that automates kVp selection based on attenuation information from the topogram or scout, and the exam type, can lead to more frequent use of lower kVp values, and such lower kVp values contribute to achieving the optimal radiation dose while maintaining the expected contrast-to-noise ratio (Table 1).
For iodinated contrast-enhanced exams, it has been demonstrated that lower kVp values result in higher contrast enhancement, especially when employed with a high-iodine-concentration contrast agent (Figure 2). Therefore, the combination of low kV imaging and high-concentration contrast media results in an overall general lower radiation dose and higher image quality. Finally, an important and relatively simple way to minimize patient radiation dose is to ensure that the patient is accurately centered within the gantry; patient off-centering increases both radiation dose and image noise. A number of other acquisition/scanning parameters can impact radiation dose. For example, using thicker rather than thinner collimation can decrease the radiation dose required for low-contrast resolution purposes. In addition, as expected, limiting the scan coverage and/or number of phases acquired has a direct and proportional impact on radiation dose.
Iodinated Contrast Administration
For ideal diagnostic performance in CT, optimization of contrast media injection protocols is critical. Goals include maximum enhancement with the least amount of contrast media and synchronization of image acquisition with contrast media delivery (ie, a tight bolus that matches the acquisition). In addition, it is beneficial to maximize the iodine flux by increasing the injection volume or injection rate, or by using a high-iodine-concentration contrast media. Using computer simulations that vary the volume, rate, and iodine concentration of the contrast, Bae and colleagues demonstrated that of these options, only increasing the iodine concentration results in an increased peak in signal with preservation of bolus timing. 3 Patient safety may be further enhanced with additional strategies for individualization of contrast administration, including using weight-based contrast dosing, reducing contrast dosing in patients at risk for contrast-induced nephropathy, and using decreased contrast injection rates in patients with poor venous access.
Image Postprocessing
Traditional reconstruction of CT images is performed using filtered back projection (FBP). This technique is fast and works well on older computers, but makes many assumptions that lead to significant image noise. Traditionally, these high background noise levels require high radiation doses to overcome. Recently available iterative reconstruction (IR) techniques use the original FBP image base but compare and refine the data in an iterative fashion, resulting in a final image with significantly lower noise levels. Iterative reconstruction techniques, available from all vendors, thus enable the acquisition of high-quality CT data at substantially lower net radiation doses (Table 1). In addition, more advanced model-based IR techniques are even more effective, allowing even lower dose levels (Figure 3).
Radiation Dose Reduction Strategies in Neuro CT Imaging
Techniques used to reduce radiation dose in neuroradiology are similar to general radiation dose reduction strategies (ie, mA and kV reduction), with several unique considerations. The 2 main techniques used for radiation dose modulation in neuro CT/CT angiography (CTA) include xyz mA modulation, since the patient diameter changes drastically as the exam proceeds from the shoulders to the neck and then head and organ-protective dose modulation, in which the radiation is reduced when the tube is closest to radiosensitive organs (eg, lens and thyroid). In addition, using modern scanners, many neuro CTA exams can be performed at tube voltages as low as 70 kVp. In general, as kVp is reduced, noise levels must be maintained with a compensatory increase in mAs. When performing CTA, where iodine rather than parenchymal visualization is the goal, the usual noise levels are not required and thus, increases in mAs are not necessary. As mentioned above, the greater enhancement of high-iodine-concentration contrast media is more optimally leveraged at lower kVp. Low kVp (80-110) can also provide better gray/white matter differentiation.
An area of particular radiation dose concern in neuroimaging is CT perfusion — a high-radiation exam with several radiation-sensitive organs within the scan range. Efforts to reduce dose when performing CT perfusion include minimizing tube current values, using a shorter scanning window and a wider sampling interval and, as for CTA, using 70 kVp to better match the iodine k edge of a high-concentration iodine agent (Figure 4). 4,5
Iterative reconstruction is universally applicable for neuro CT scanning, optimizing low contrast resolution while maintaining high contrast detectability. In populations expected to undergo multiple, repeated examinations, eg, neuro intensive care, IR can be a critical component of an ultra-low–radiation dose approach for surveillance of an established diagnosis. 6
Radiation Dose Reduction Strategies in Body CT Imaging
The use of body CT is increasing, and with the increasing number of new applications and protocols, it is the responsibility of radiology practices to understand how to minimize radiation exposure while ensuring that diagnostic quality is not compromised. The acceptable image quality and noise level varies with the preference of the radiologist, but for many clinical situations, indication-appropriate image quality customization can be achieved while still reducing radiation dose. In specific instances, scan acquisitions can be limited and still provide all of the needed information; for example, for CT colonography, excluding the lung bases and limiting the scan to the colon can not only help reduce radiation dose, it can also reduce the number of incidental, extracolonic findings. If it is not possible to limit the range of the entire scan, the range of a particular phase (eg, delayed phase) can be limited, or an entire phase (eg, noncontrast phase) can be eliminated, while still obtaining all of the required information.
One approach to lowering radiation dose for body CT exams is to consider grouping exams into low-contrast, high-contrast, and screening/frequent follow-up exams. For low-contrast exams (eg, evaluation of masses or tissue characterization) such as routine portal venous phase abdomen exams, we would preferentially utilize a low mA technique with a weight-based kVp (ie, 80 or 100 kVp for lighter patients). For high-contrast exams (eg, CTA, CT urogram), the priority should be to optimize (lower) the kVp, resulting in higher contrast at a lower radiation dose, and then the mA would be optimized based on the kVp and body size. Finally, for screening studies or in those needing frequent follow-up exams (eg, CT colonography, follow-up to a recent procedure), greater dose reduction can be achieved by keeping both the mA and the kVp at a minimum.
Iterative reconstruction techniques can be applied in body CT to improve image quality at the same radiation dose, or to reduce noise, thereby preserving image quality at a lower radiation dose. The effectiveness of statistical IR in a patient with Crohn’s disease is shown in Figure 5: The initial exam was done with FBP and an estimated effective radiation dose of 11.6 mSv, while a follow-up exam was done using IR at a 50% dose reduction, with comparable image quality. Patients with Crohn’s disease are often younger and require multiple CT exams to monitor their clinical status; therefore, the use of IR in cases like these are particularly impactful.
For contrast-enhanced body CT and CTA, it is beneficial to maximize the iodine flux and, as mentioned above, iodine concentration increase results in an increased peak in signal with preservation of bolus timing and injection rate. 3 In an intraindividual comparison of 300 mgI/mL vs 370 mgI/mL contrast media in 20 patients with chronic liver disease, the higher-iodine-concentration contrast media injection was shown to improve enhancement of the liver parenchyma in the portal- and late-phase images, and to improve the image contrast, overall image quality, and diagnostic accuracy of the liver CT exam. 7 Similarly, for abdominal CTA in 80 renal transplant donors, 370 mgI/mL contrast media provided similar diagnostic accuracy but greater contrast enhancement and image quality compared to 300 mgI/mL. 8
Radiation Dose Reduction Strategies in Cardiovascular CT Imaging
As with neurologic and body applications, the goal in CVCT is to keep radiation dose low, particularly in pediatric patients. This is achieved by balancing radiation exposure and acceptable noise. Several advanced techniques allow the use of lower radiation doses while ensuring that the exam provides the required information, and does not need to be repeated or lead to additional diagnostic imaging.
For CVCT, Z-axis tube current modulation allows delivery of the optimum current for a patient’s specific body habitus. ECG-based tube pulsing can reduce the radiation dose during a retrospective EKG-cardiac CT angiogram by reducing the current during systole and reserving the higher values for the diastolic phases — when there is the least amount of cardiac motion. The availability of 70 kV imaging on newer scanners provides an attractive option for visualization of cardiovascular structures without sacrificing diagnostic quality. Automated kV software can help determine the appropriate use of such low-voltage acquisitions. Dual-energy imaging can reduce radiation dose by providing virtual noncontrast scans and low voltage acquisitions.
With the availability of faster scanners, there are reduced motion artifacts. This is a key advantage for pediatric CVCT and is leading to increased use of CTA for the evaluation of congenital heart disease in pediatric patients. In this patient population, it is particularly important to modify protocols to ensure minimal radiation exposure. The combination of lowering kV and using a high–iodine-concentration contrast media can provide significant radiation-dose reduction without compromising image quality (Figure 6).
Conclusions
When implemented appropriately, the core radiation-dose reduction strategies discussed above can greatly reduce patient exposure while maintaining image quality. Ensuring appropriate indications and scan coverage, as well as using the lowest possible mA and kV, should always be the cornerstones of a thoughtful and responsible approach to diagnostic CT. Techniques such as tube current modulation, low-kV imaging, and IR allow increased contrast enhancement at lower doses with acceptable noise levels. Ensuring high iodine flux with high-concentration contrast media contributes to improved diagnostic performance. Designing protocols for each indication, considering radiation and image quality, will allow overall doses to be contained while CT use continues to advance.
References
- Brenner DJ. Should we be concerned about the rapid increase in CT usage? Rev Environ Health. 2010;25:63–68.
- Mettler FA Jr, Bhargavan M, Faulkner K, et al. Radiologic and nuclear medicine studies in the United States and worldwide: frequency, radiation dose, and comparison with other radiation sources—1950-2007. Radiology. 2009;253:520–531.
- Bae KT. Intravenous contrast medium administration and scan timing at CT: considerations and approaches. Radiology. 2010;256:32–61.
- Corcuera-Solano I, McLellan AM, Doshi AH, Pawha P1, Tanenbaum LN. Whole-brain adaptive 70-kVp perfusion imaging with variable and extended sampling improves quality and consistency while reducing dose. Am J Neuroradiol. 2014;35:2045–2051.
- Li ZL, Li H, Zhang K, et al. Improvement of image quality and radiation dose of CT perfusion of the brain by means of low-tube voltage (70 KV). Eur Radiol. 2014;24:1906–1913.
- Corcuera-Solano I, Doshi AH, Noor A, Tanenbaum LN. Repeated head CT in the neurosurgical intensive care unit: feasibility of sinogram-affirmed iterative reconstruction-based ultra-low-dose CT for surveillance. Am J Neuroradiol. 2014;35:1281–1287.
- Furuta A, Ito K, Fujita T, Koike S, Shimizu A, Matsunaga N. Hepatic enhancement in multiphasic contrast-enhanced MDCT: comparison of high- and low-iodine-concentration contrast medium in same patients with chronic liver disease. Am J Roentgenol. 2004;183:157–162.
- Rau MM, Setty BN, Blake MA, Ouellette-Piazzo K, Hahn PF, Sahani DV. Evaluation of renal transplant donors with 16-section multidetector CT angiography: comparison of contrast media with low and high iodine concentrations. J Vasc Interv Radiol. 2007;18:603–609.
- McCollough CH, Chen GH, Kalender W, et al. Achieving routine submillisievert CT scanning: report from the summit on management of radiation dose in CT. Radiology. 2012;264:567–580.