Clinical Applications of Gadopiclenol in Pediatric MRI
By Vasanawala S
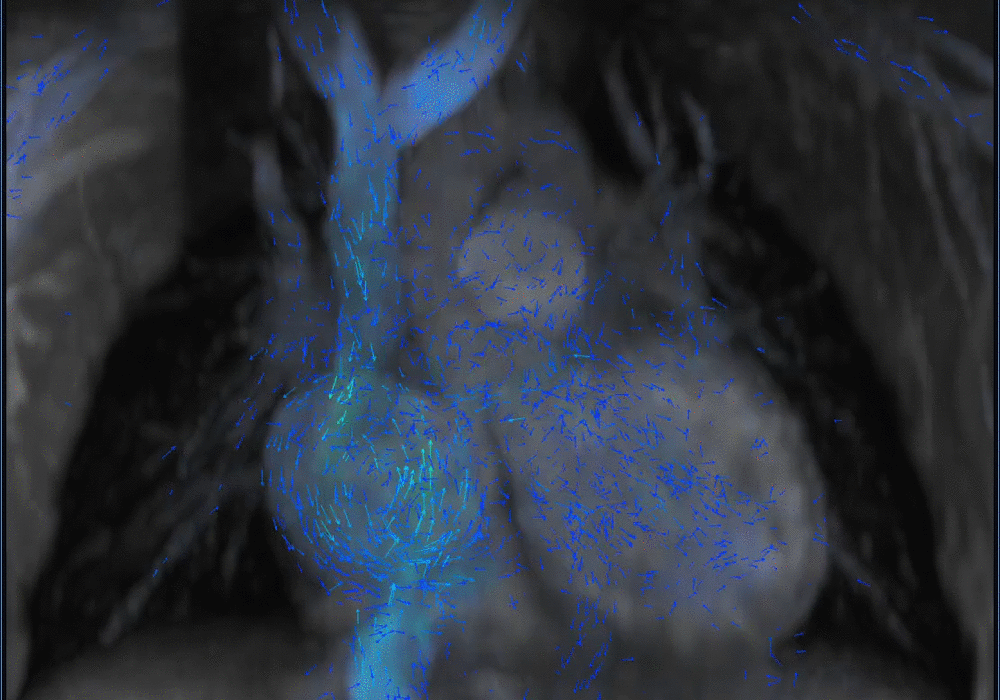
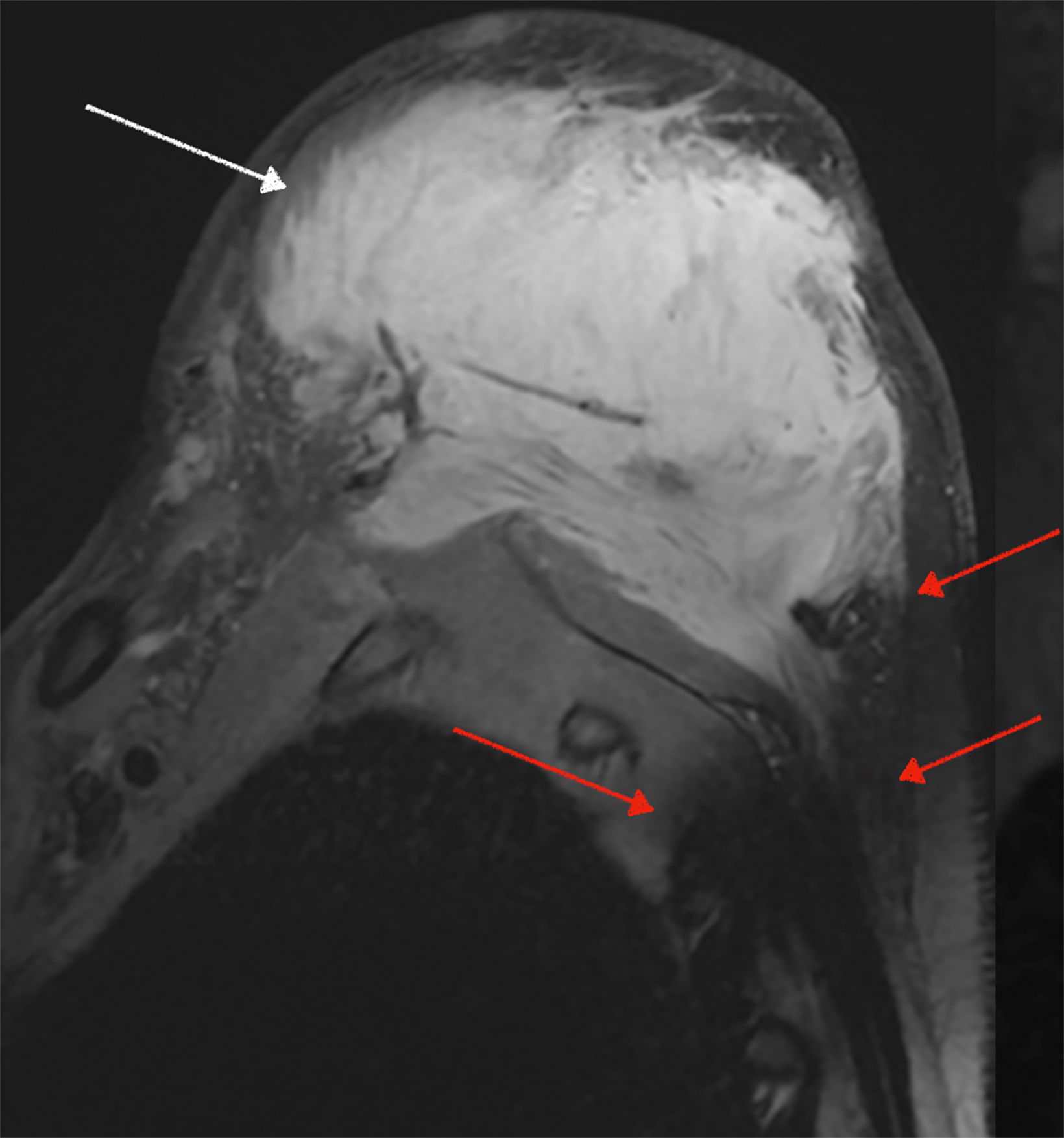
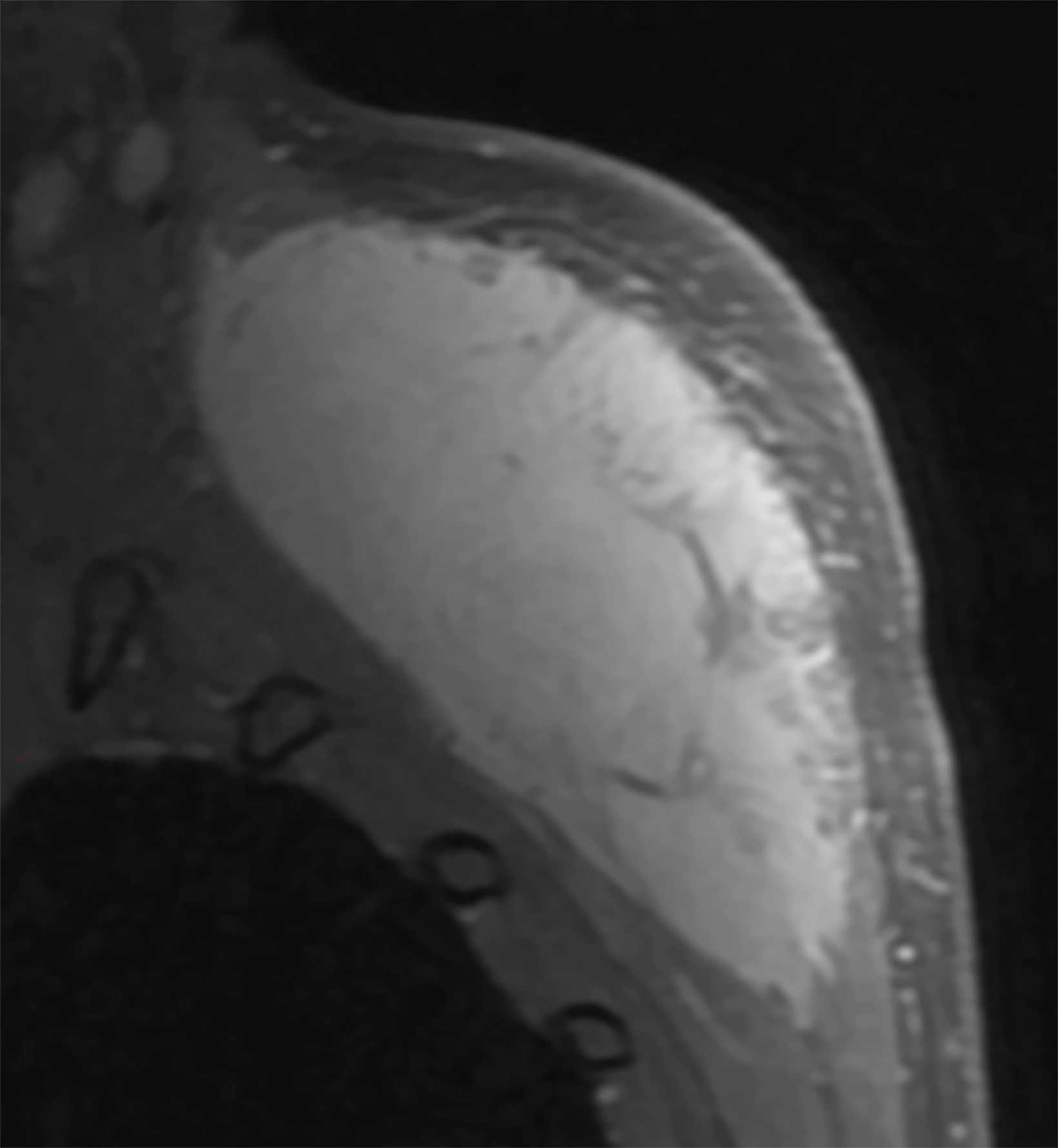
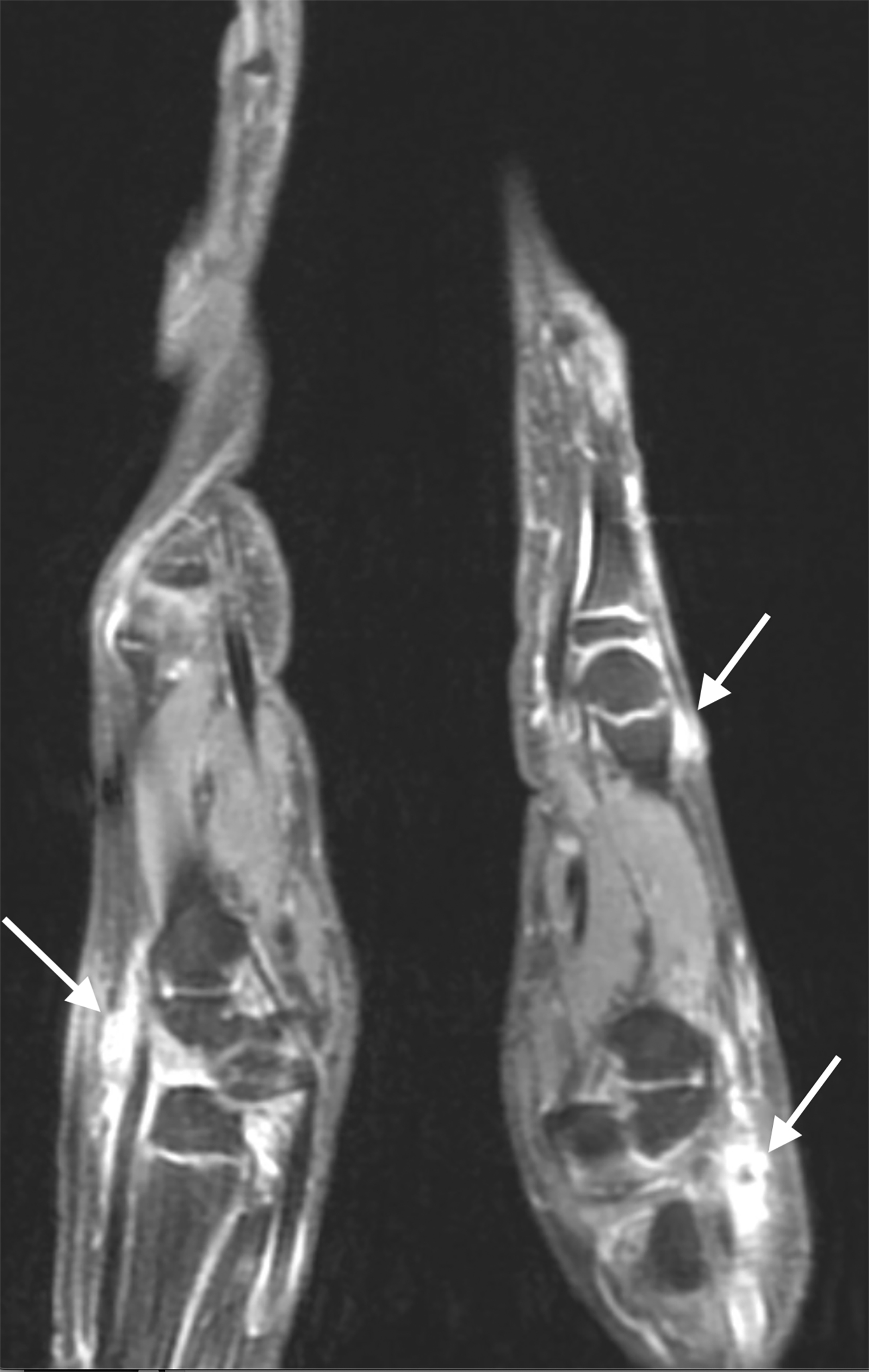
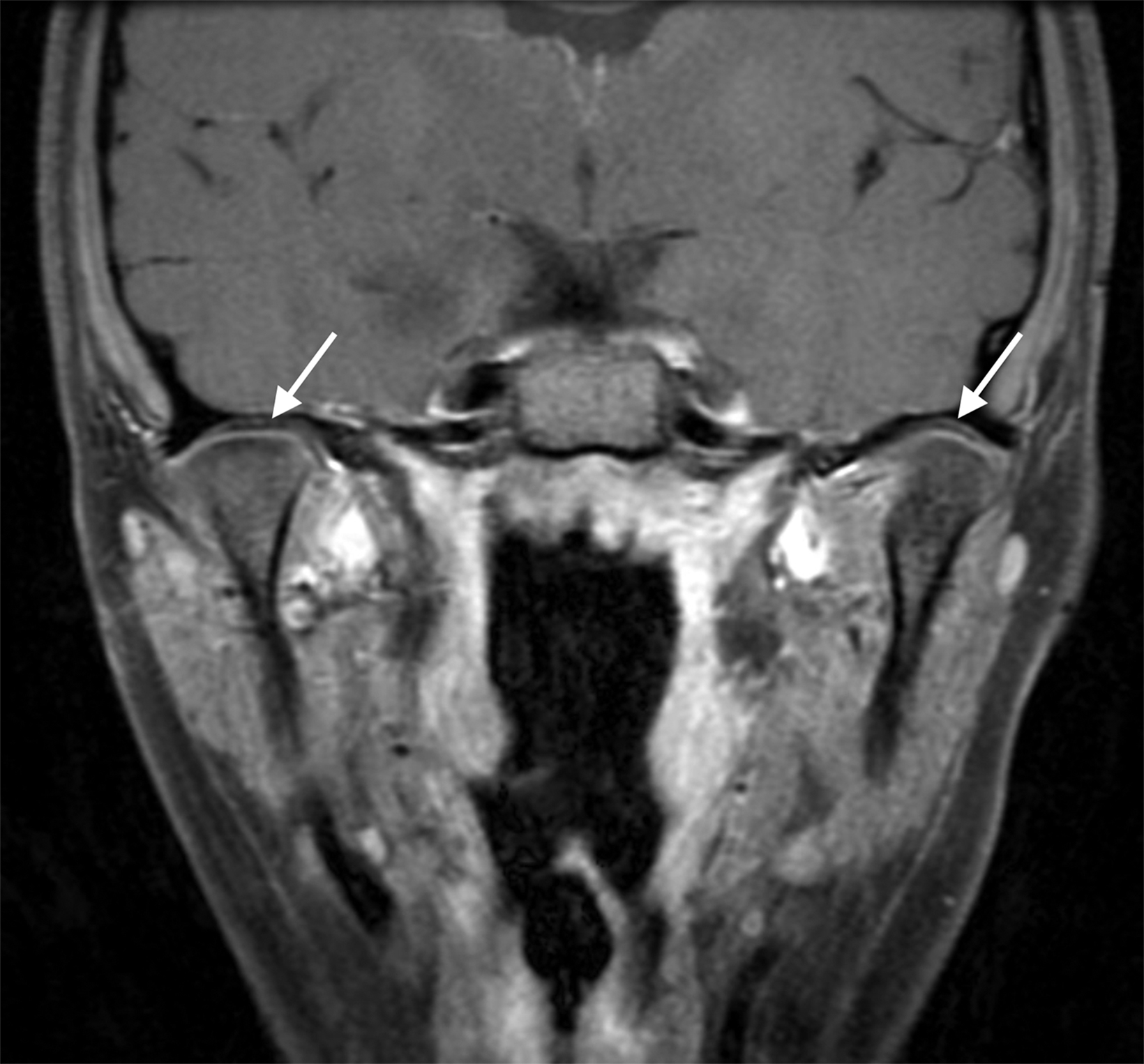
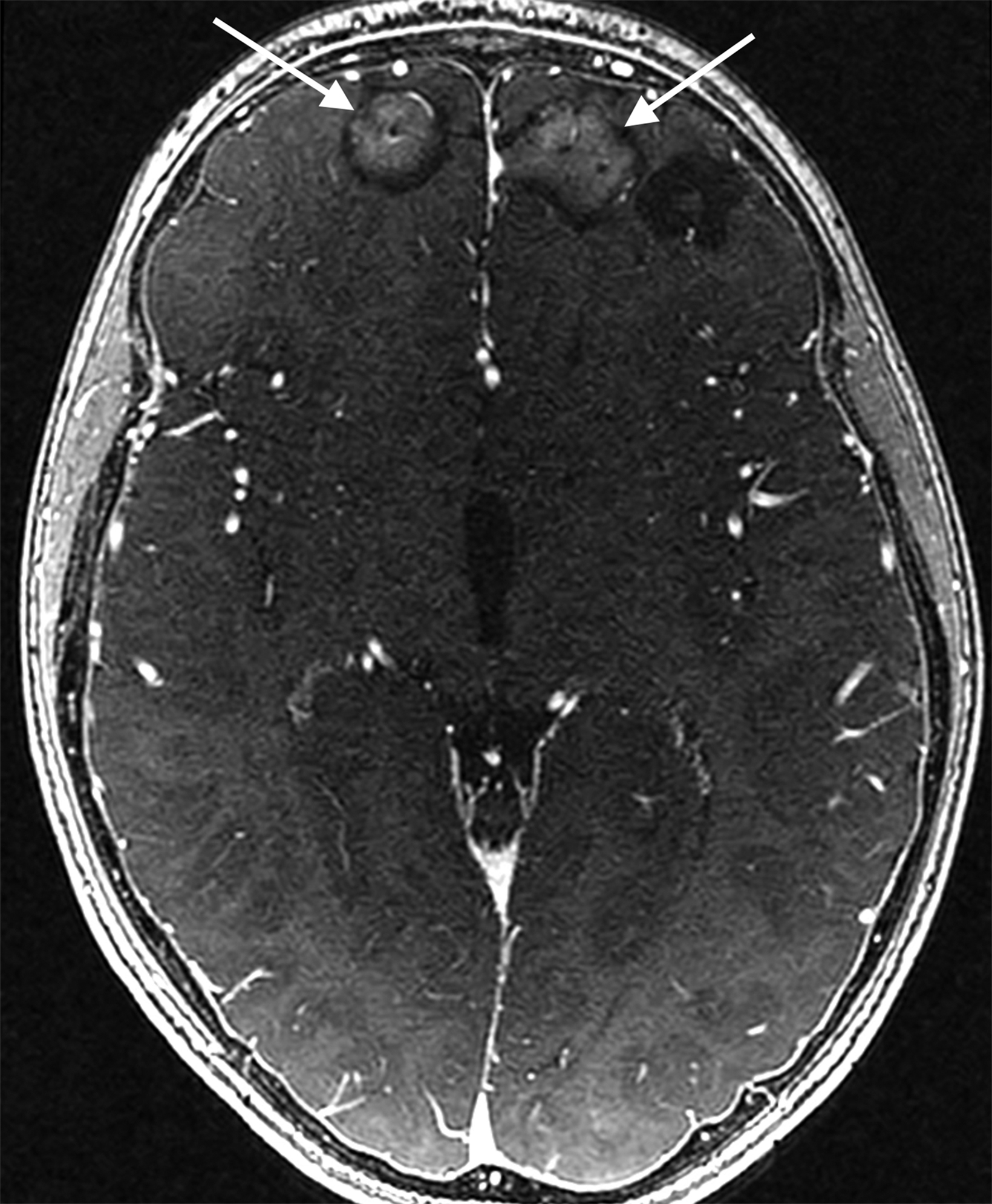
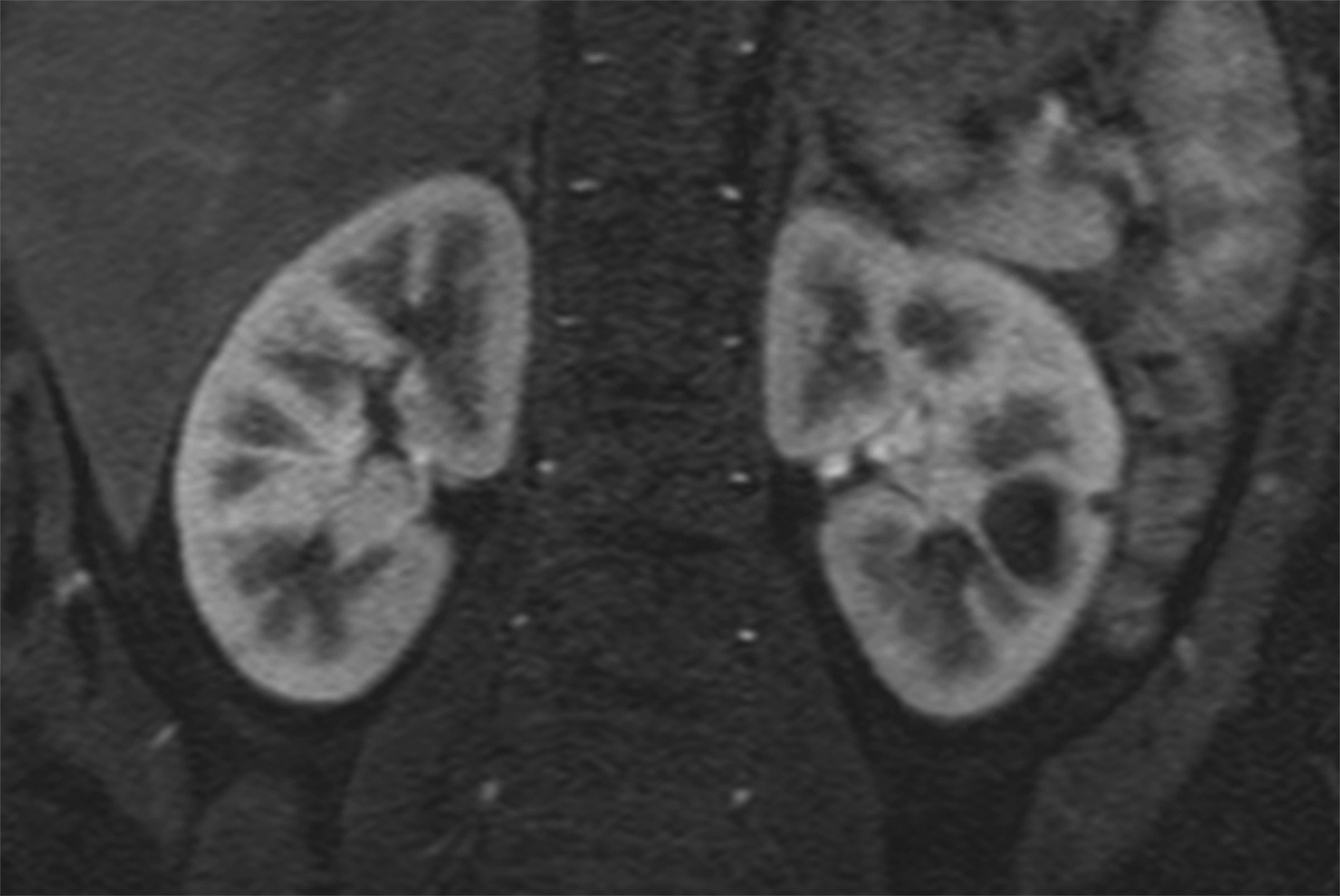
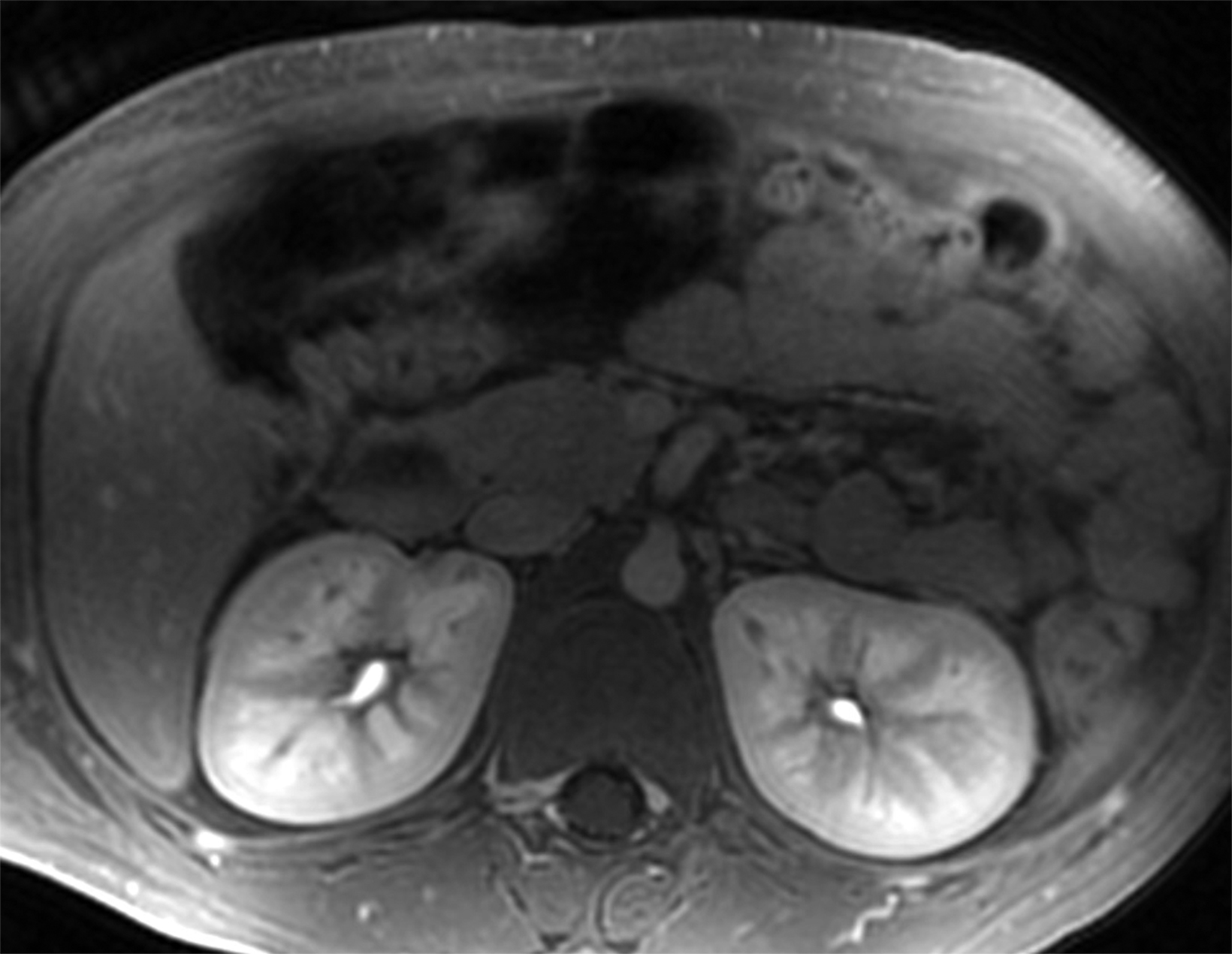
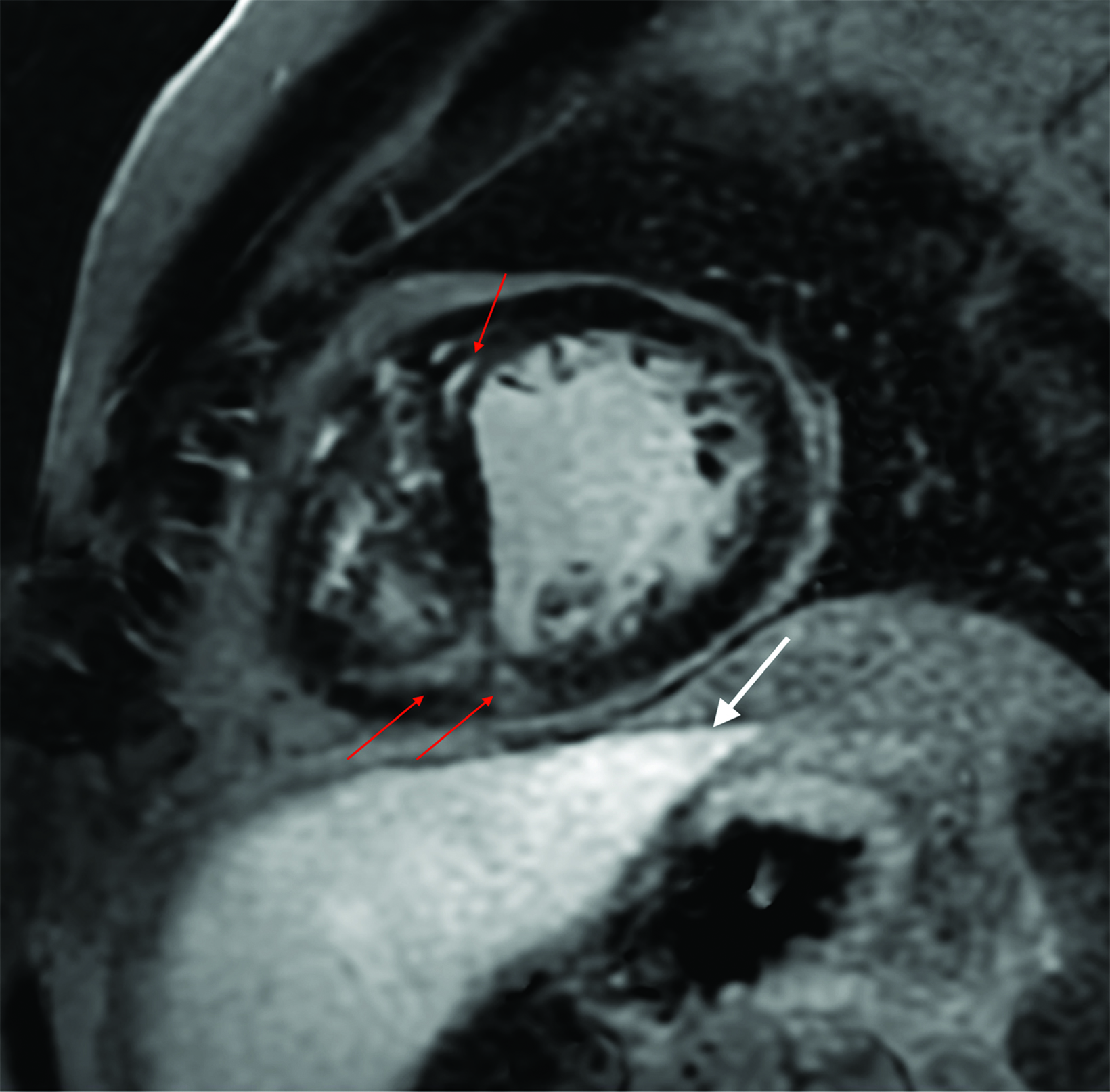
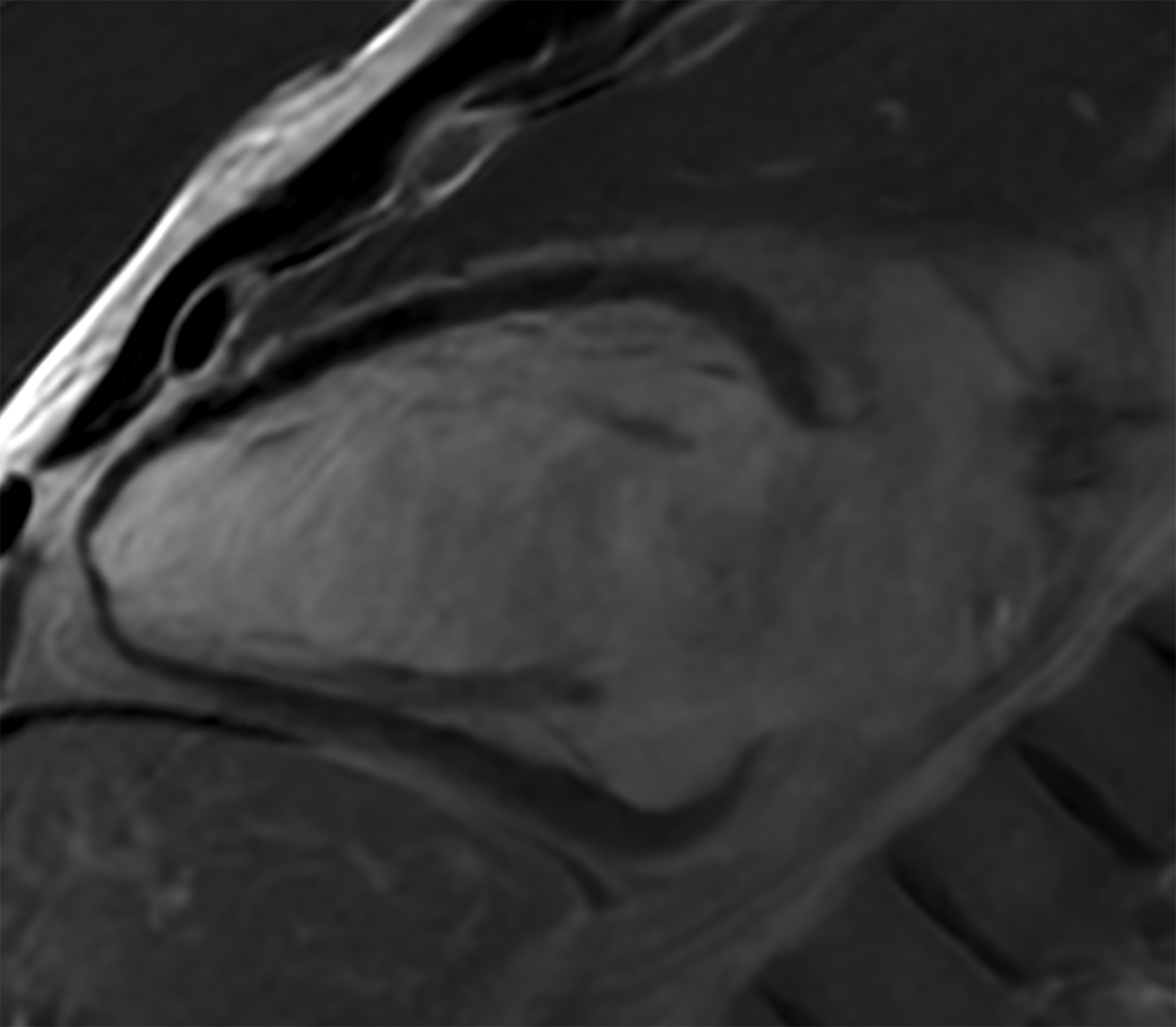
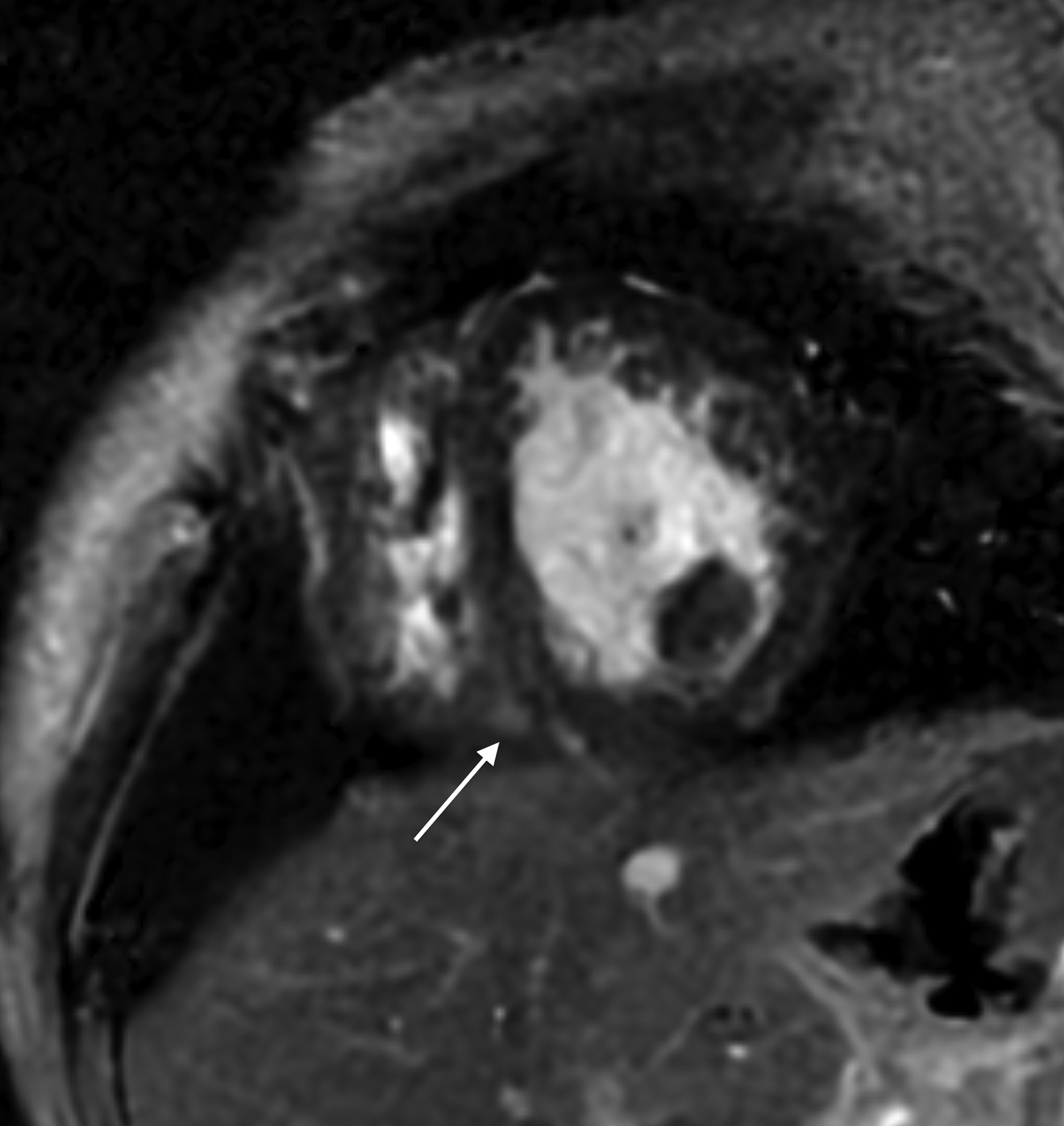
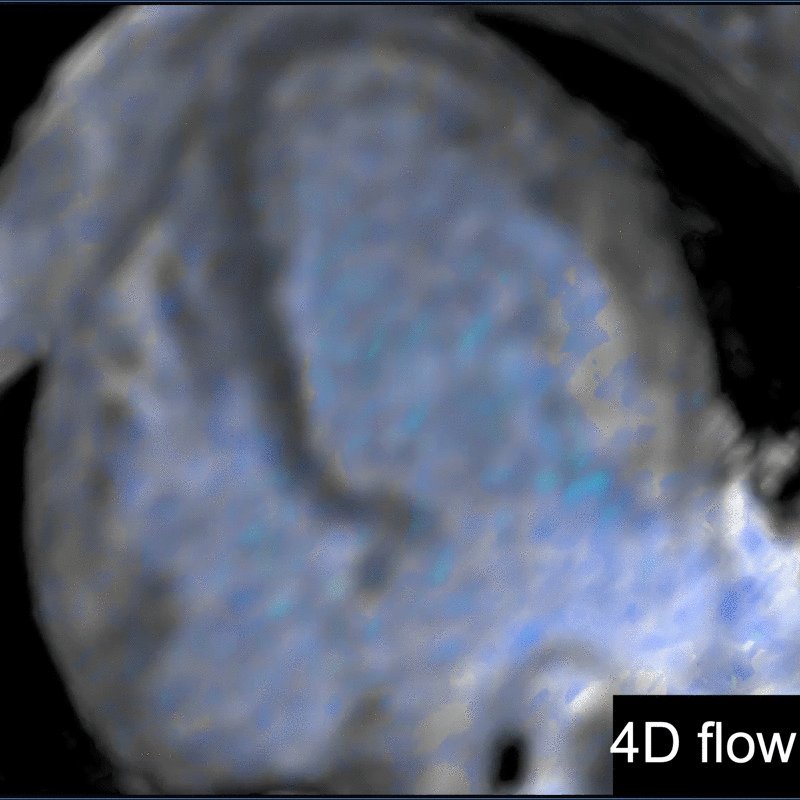
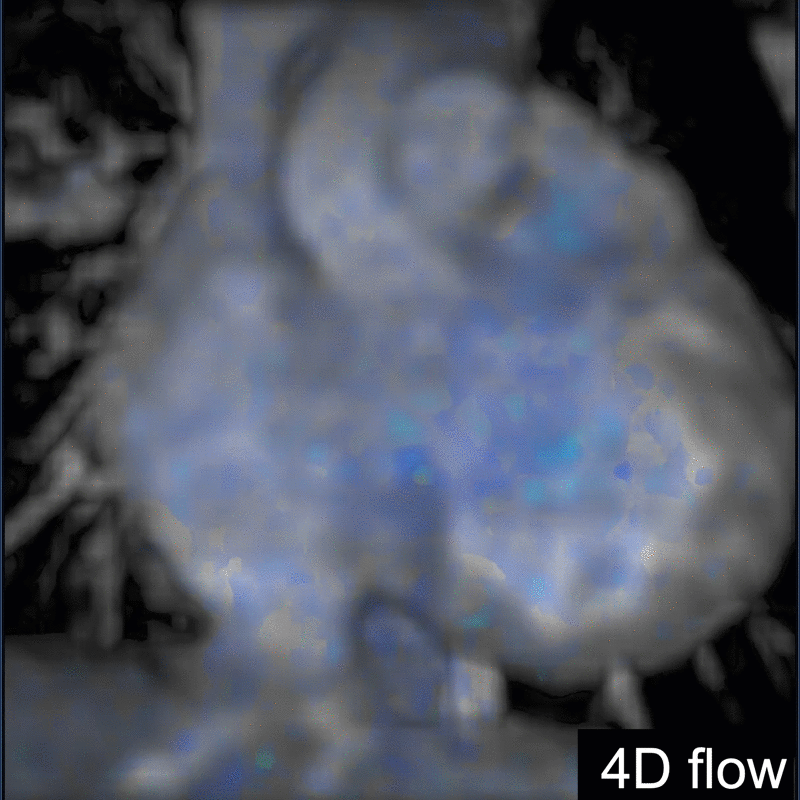
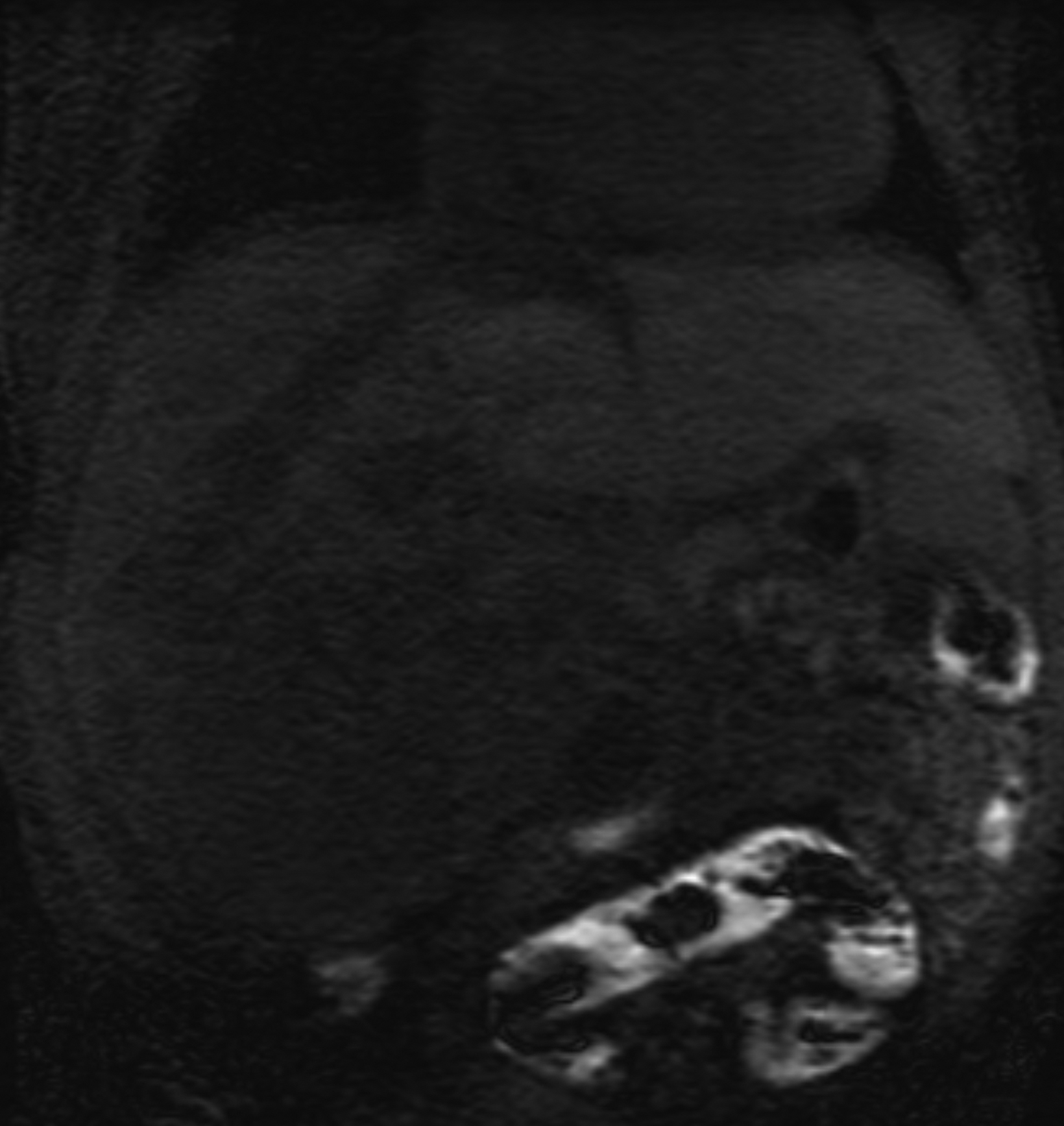
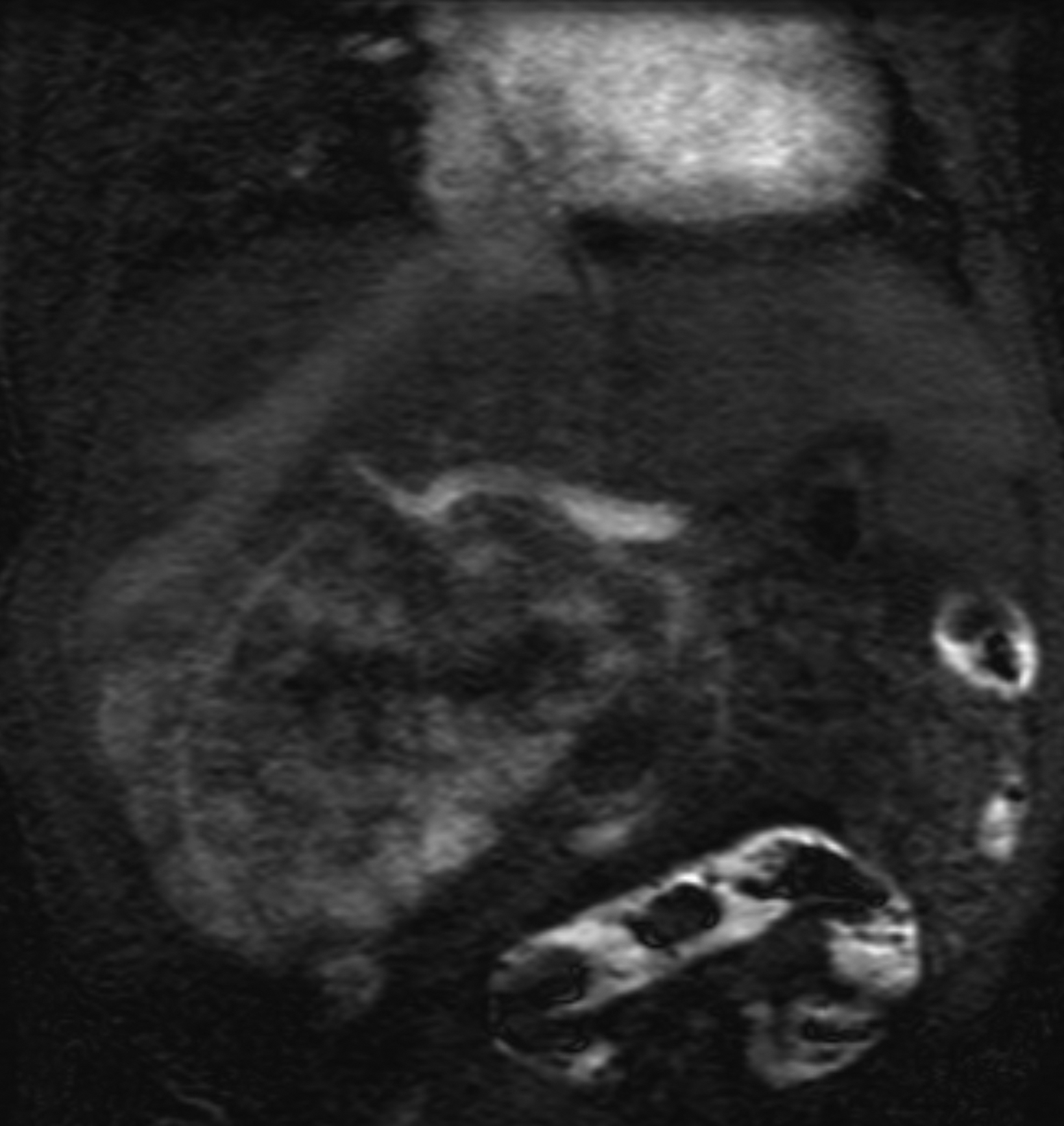
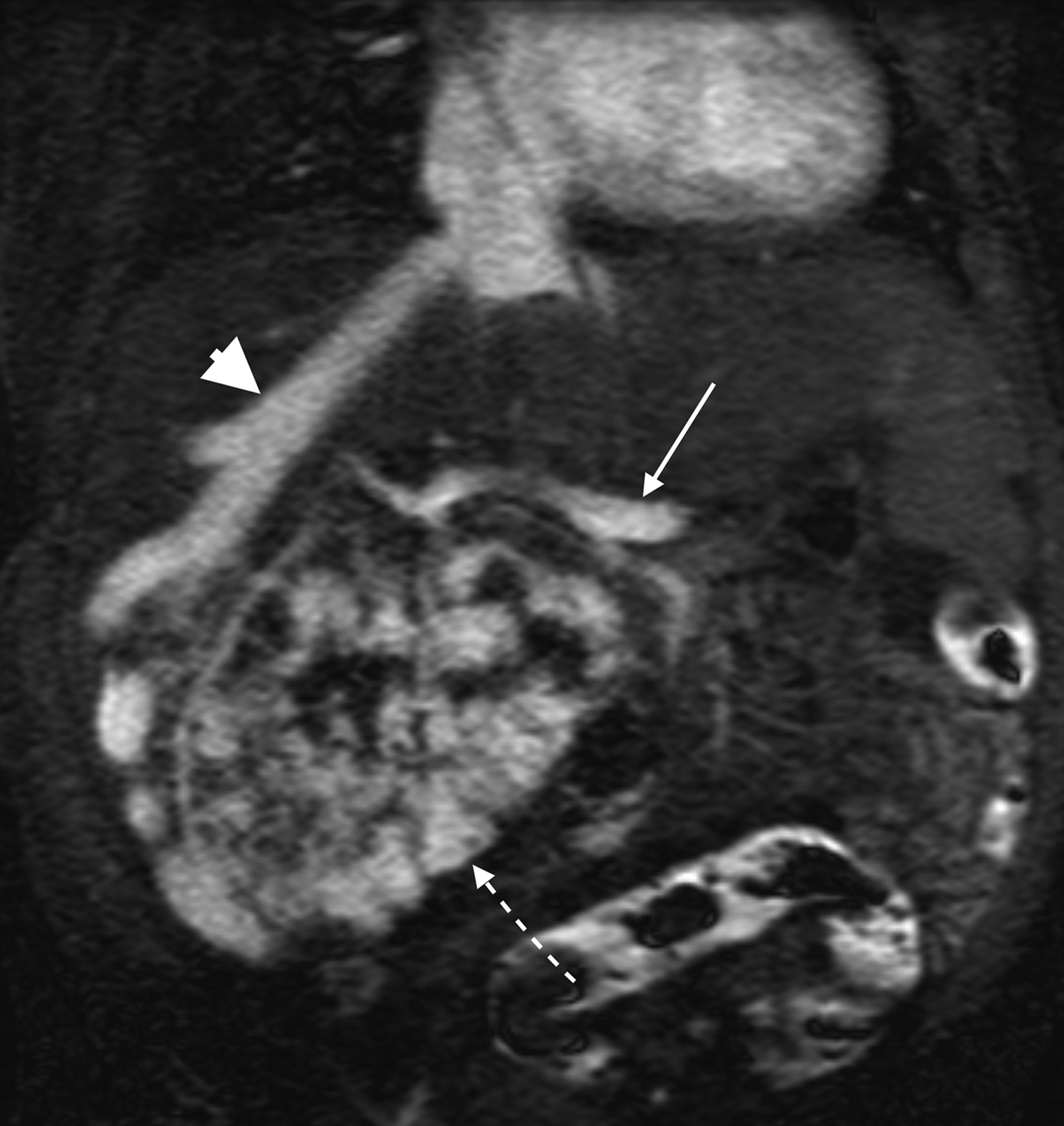
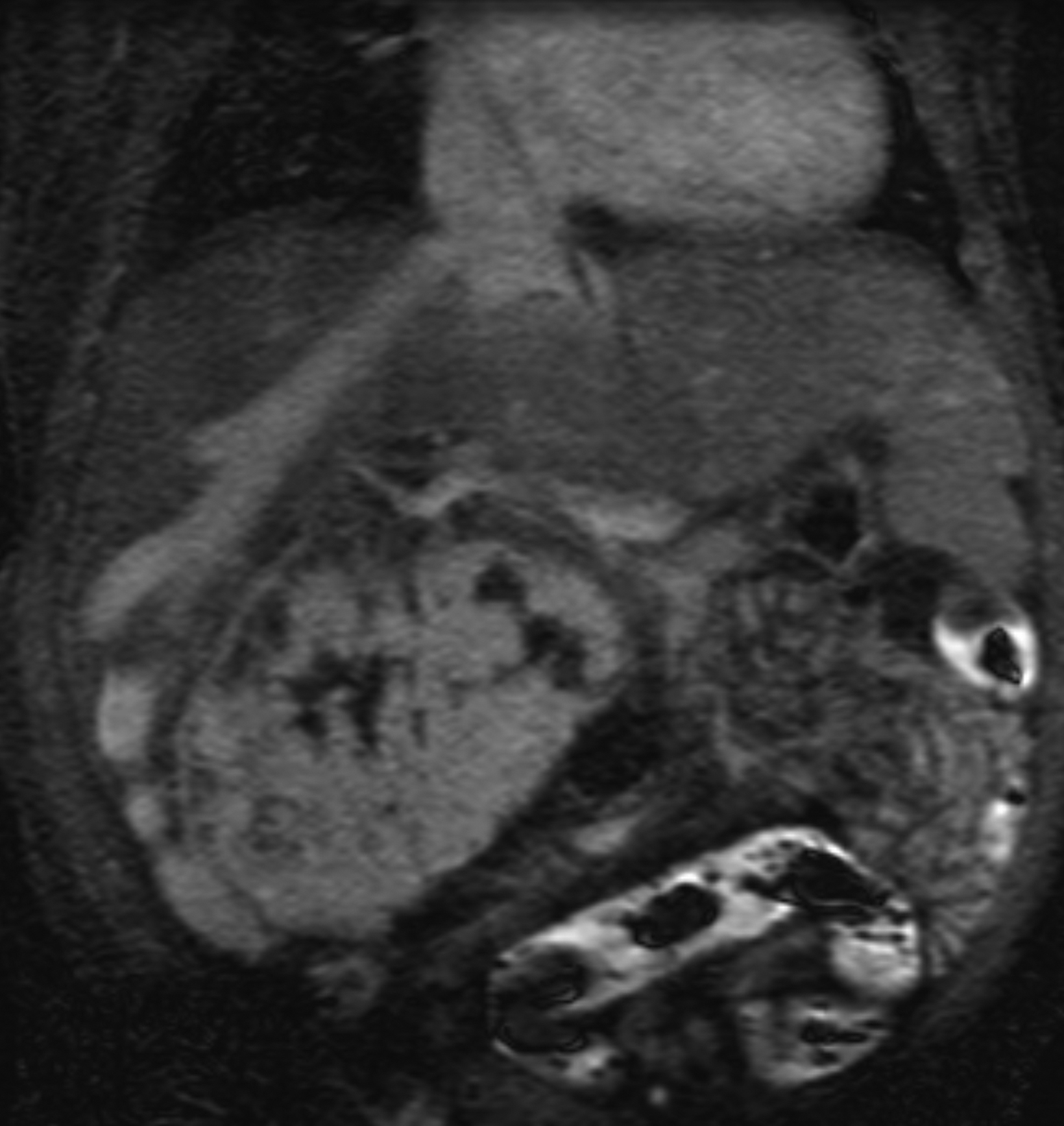
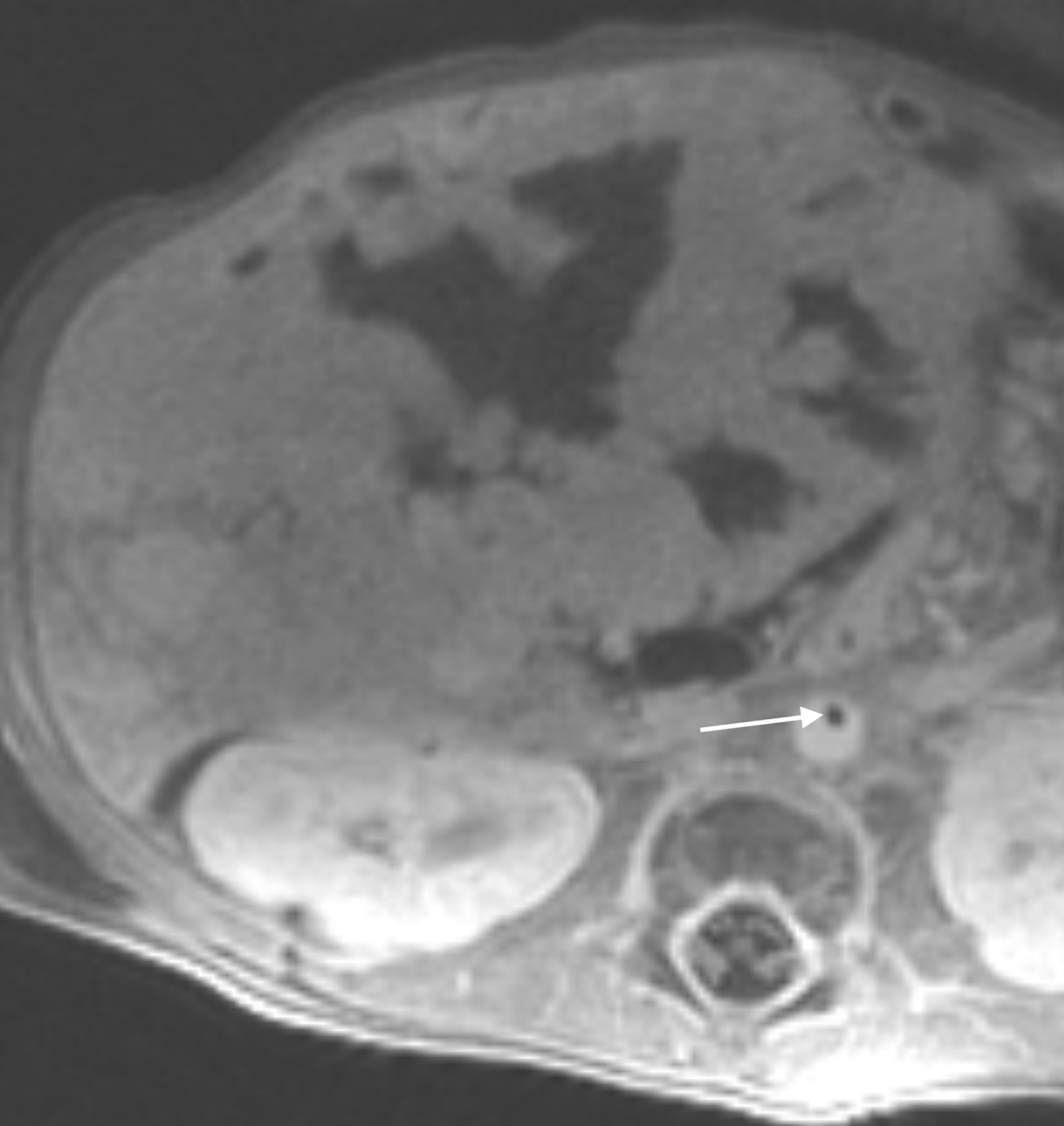
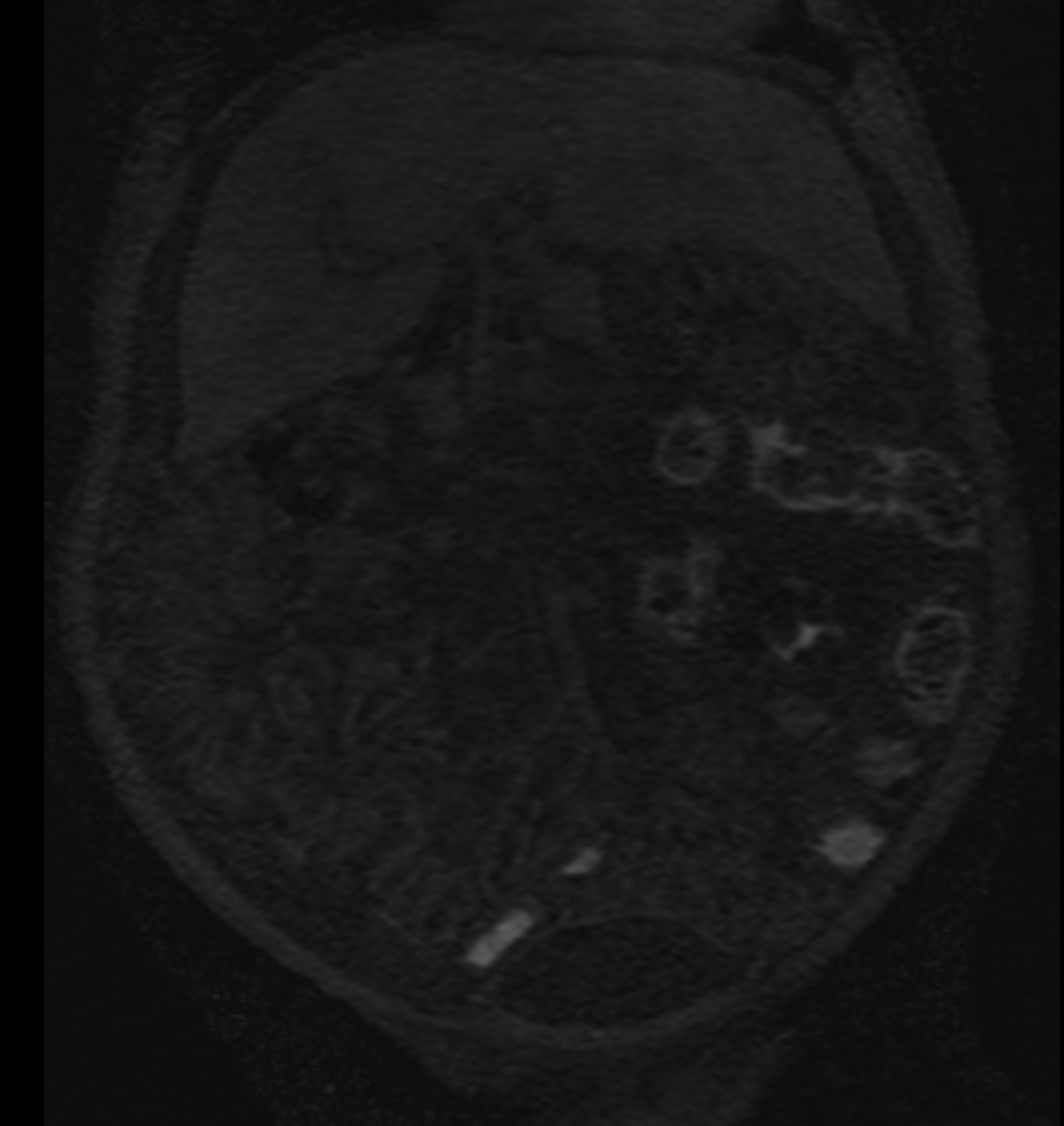
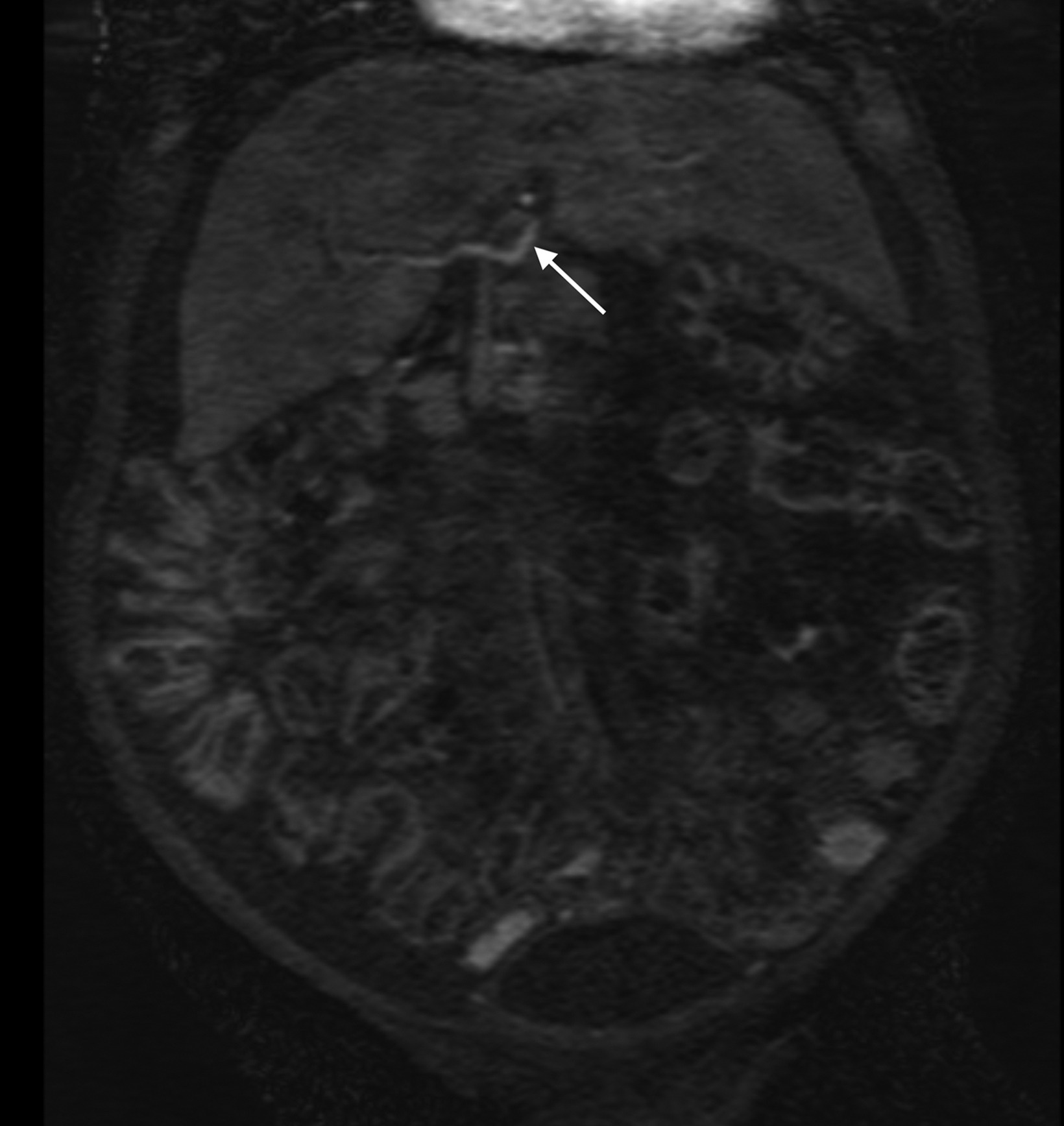
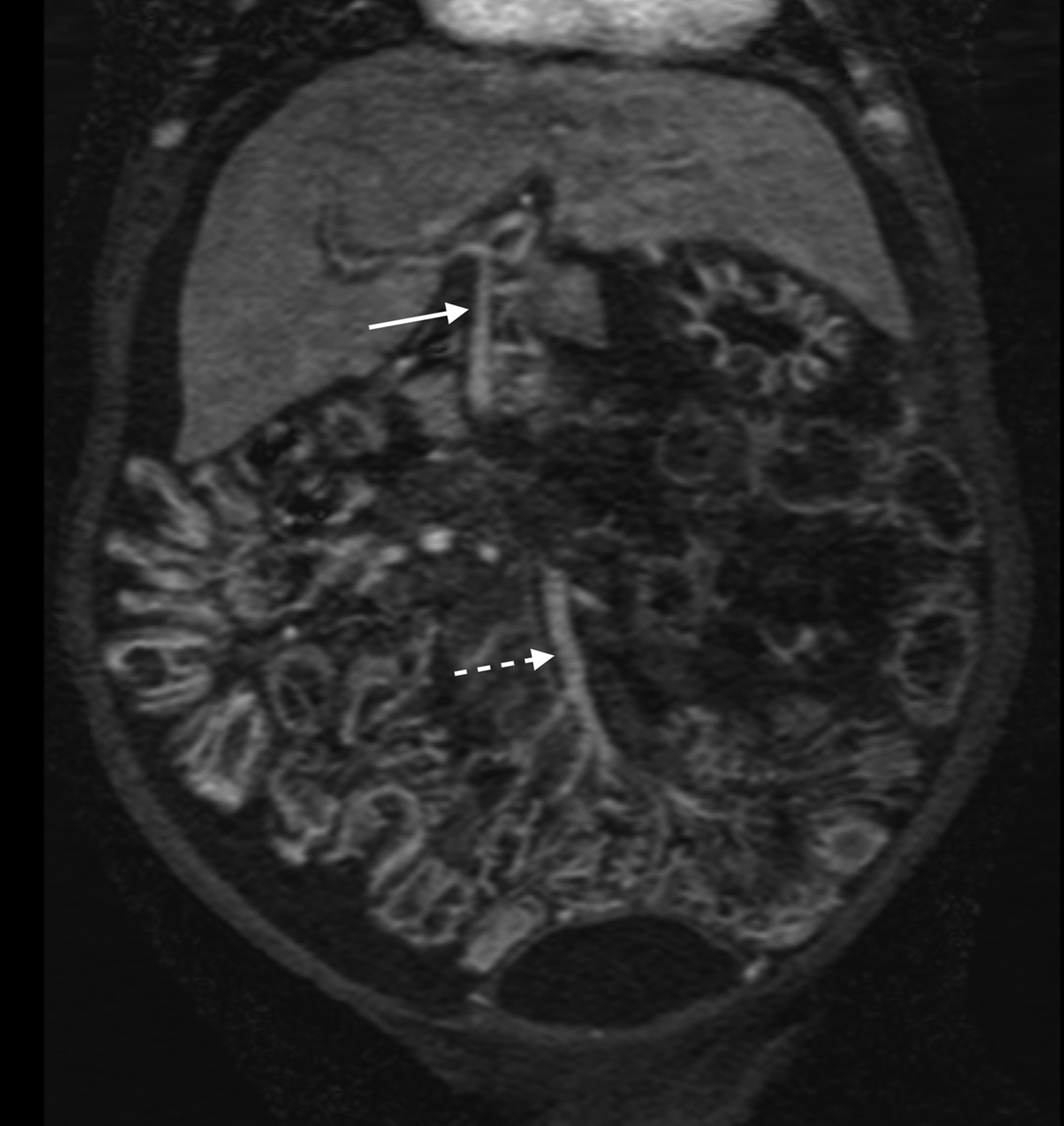
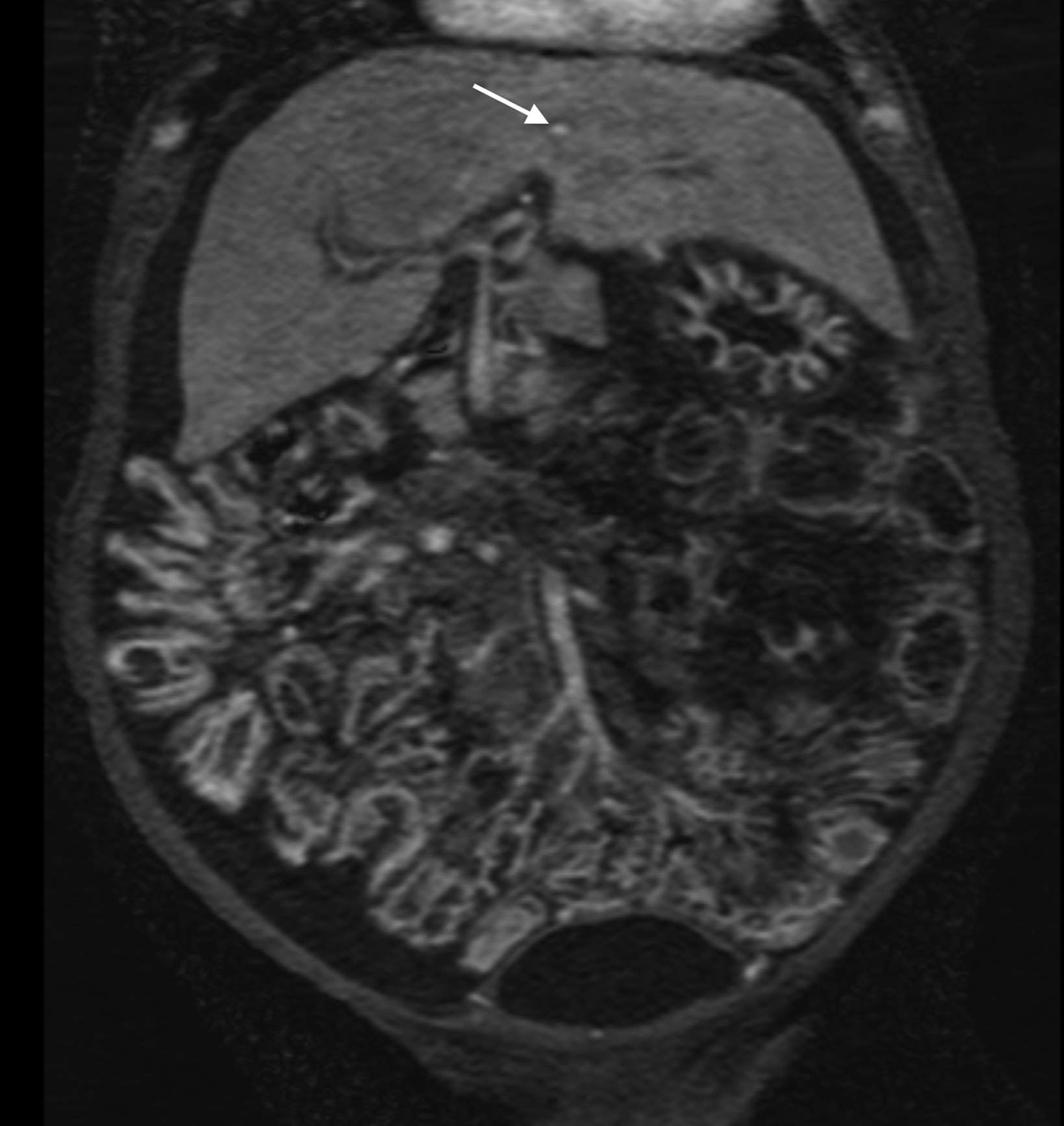
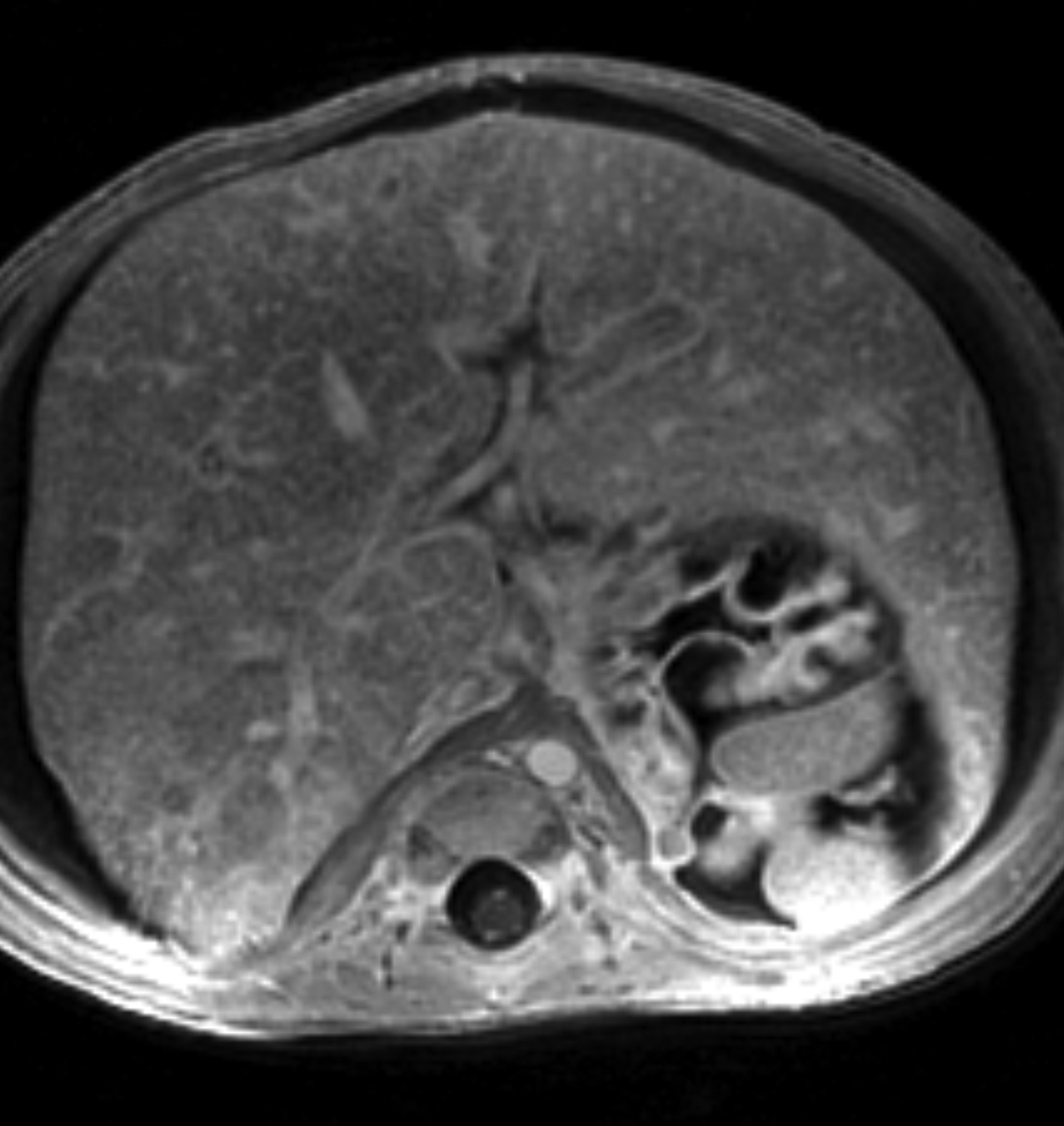
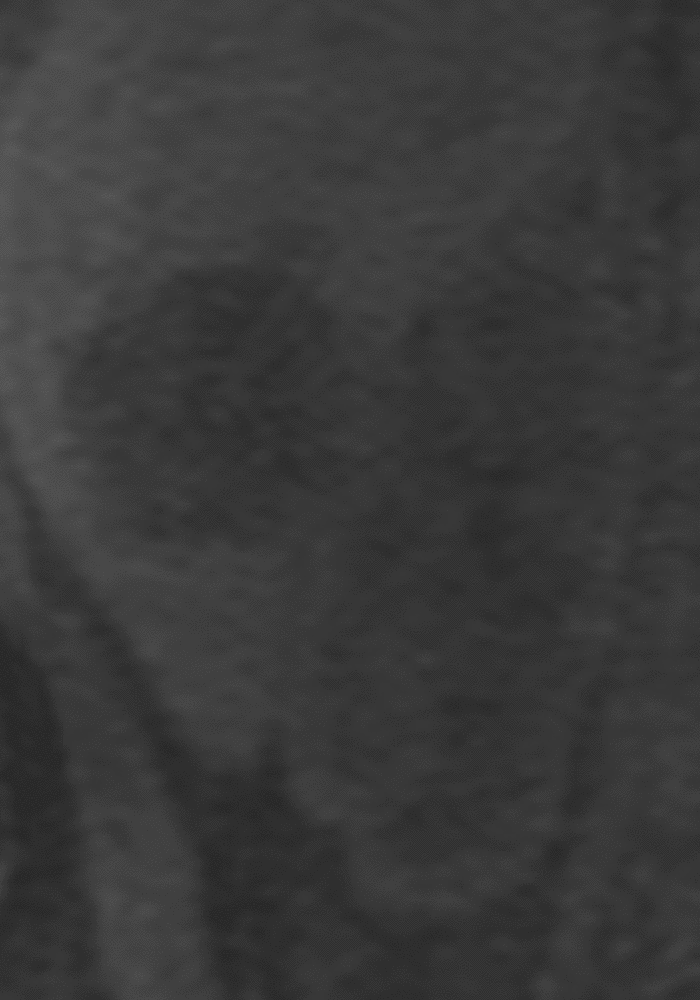
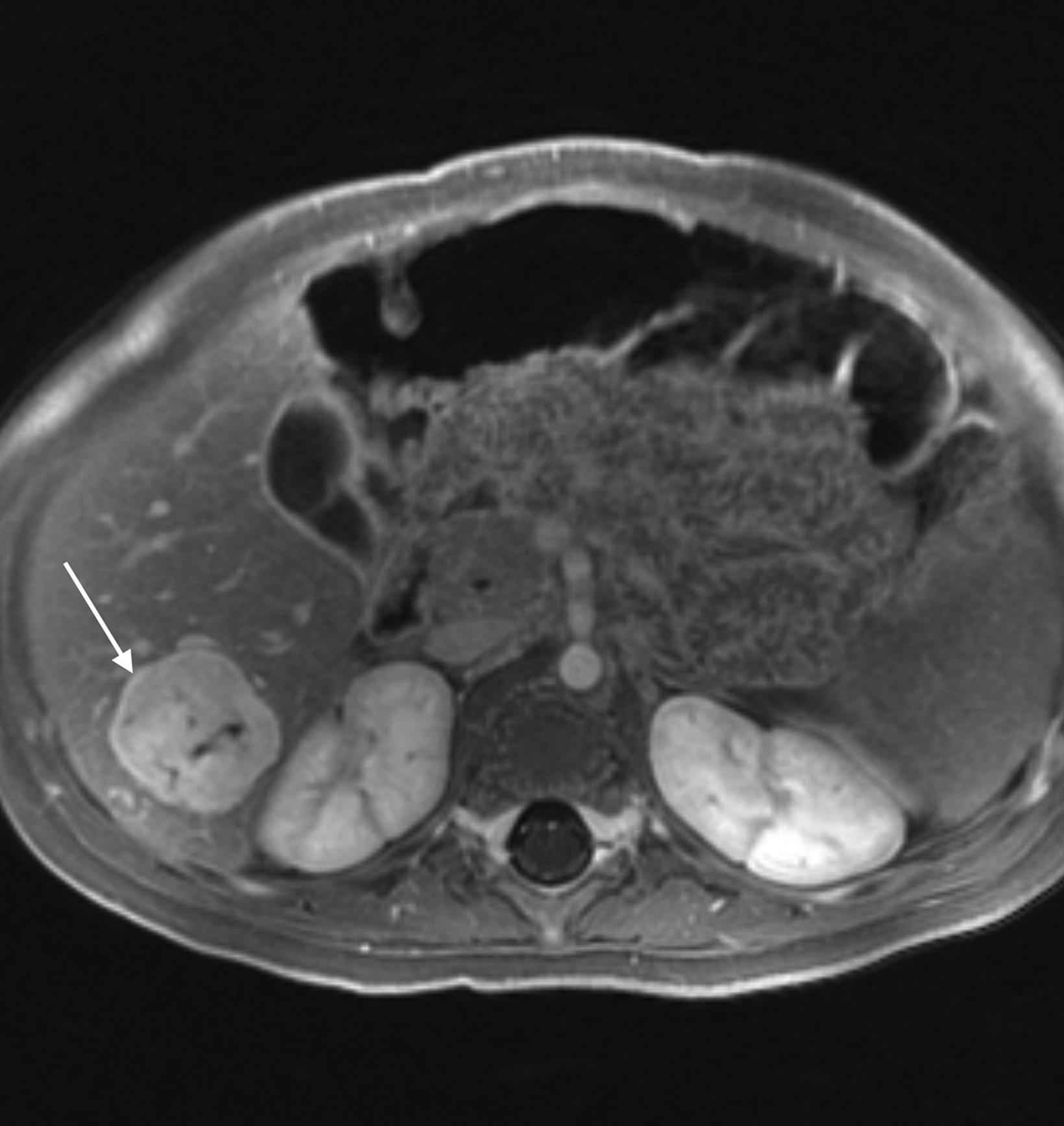
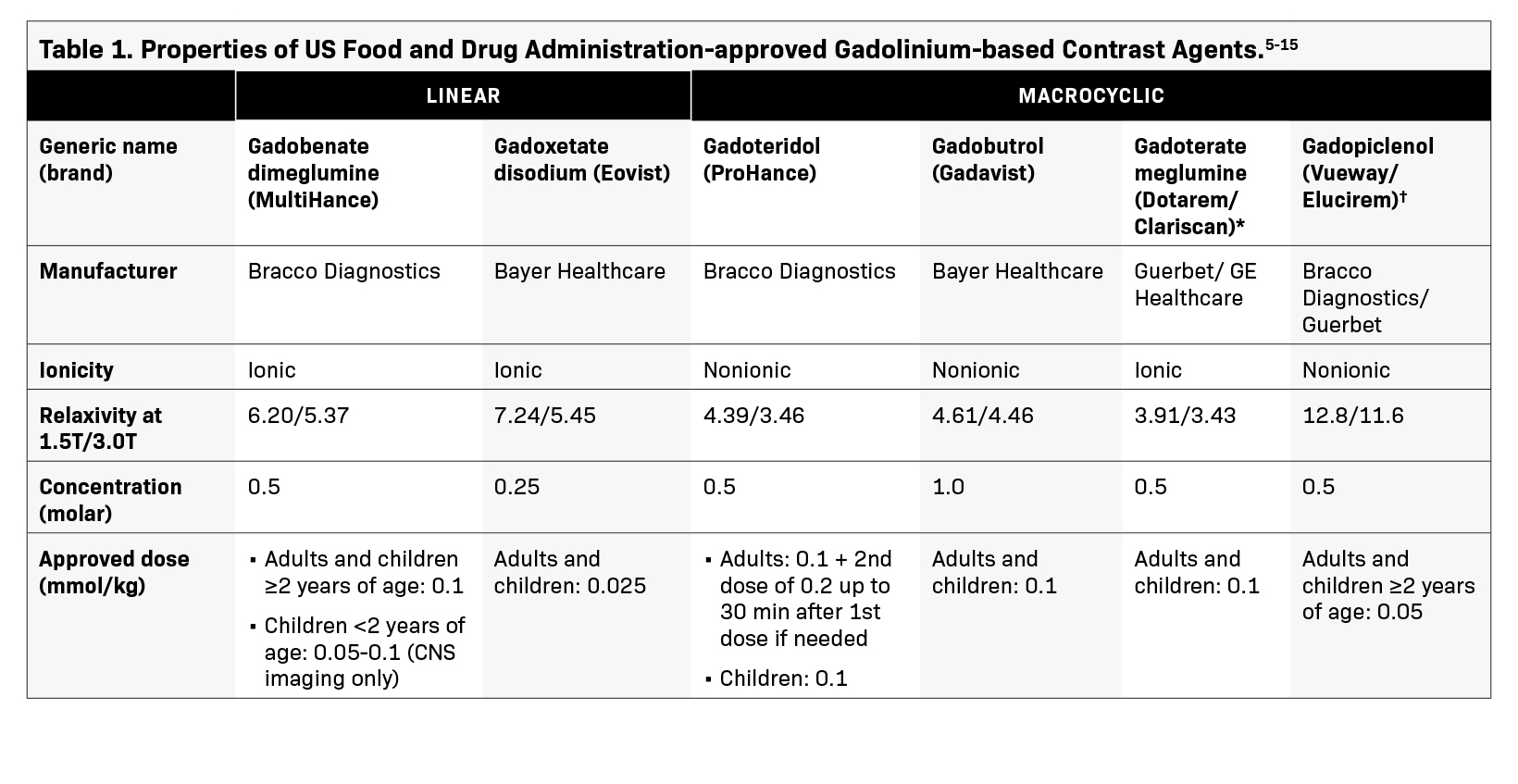
CE and CRA credit are available for this article here.
Gadolinium-based contrast agents (GBCAs) have been a mainstay in contrast-enhanced magnetic resonance imaging (MRI) for over 30 years owing to their ability to improve the diagnostic value of examinations.1 Their broad utility is evident in their diverse indications, including lesion detection and characterization, cancer staging, delineating vasculature and associated diseases, and assessing infection and inflammation.2
Although GBCAs have a favorable safety profile and are used routinely across MRI indications, recent concerns have led to the voluntary marketing suspension of the less stable agents—gadopentetate dimeglumine (Magnevist), gadodiamide (Omniscan), and gadoversetamide (OptiMARK).3 Given that pediatric patients, in particular, may receive multiple doses over their lifetime, the careful selection and prudent use of GBCAs are paramount to optimizing diagnostic efficacy and ensuring patient safety for this group.2 This paper reviews considerations for selecting and utilizing GBCAs in pediatric MRI, focusing in particular on the new macrocyclic agent, gadopiclenol.
GBCAs: A Historical Perspective
Since its introduction in the 1980s, MRI has become an indispensable tool for diagnostic imaging, accelerated by the development of GBCAs.1 Current estimates suggest that GBCAs are used in 30% to 45% of the approximatel 40 million MRI examinations performed annually in the US and in 40% of all pediatric MRI studies.1,4
The six GBCAs currently available for use in children differ in their physicochemical properties, such as structure, stability, relaxivity, ionicity, and viscosity, all of which contribute to their clinical utility and risk profiles (Table 1).5-14 When used at therapeutic doses, these agents are generally considered safe; however, caution should be exercised when imaging certain patient groups.15 The first disease to be linked to GBCA utilization was nephrogenic systemic fibrosis (NSF), a rare, debilitating, scleroderma-like condition that often progresses to joint contractures and decreased mobility.15,16 Nephrogenic systemic fibrosis was first reported in 2000 in a publication that described patients on renal dialysis with scleromyxoedema symptoms; however, the condition’s link to GBCAs was not established until 2006.16,17 While the exact mechanism remains unclear, acute or chronic renal impairment is widely believed to increase the risk of NSF in patients exposed to GBCAs.18 Furthermore, it is believed that the prolonged GBCA clearance time in renally impaired patients increases the opportunity for gadolinium ions to dissociate from the chelate and bind with other anions to create insoluble precipitates that deposit in various tissues.15
Increased awareness and the regulatory actions implemented since the first report of NSF have led to limited use of GBCAs in at-risk patients, effectively eliminating the occurrence of new cases.15 In fact, a recent systematic review reported that only seven GBCA-associated NSF cases have occurred since 2008.19 Additionally, the vast majority of the 639 biopsy-confirmed cases are among adult patients, suggesting that NSF is less common among children.19 This was confirmed in a 2014 review that identified only 23 pediatric NSF cases reported through September 2012, none of which occurred in patients younger than six years of age.20
GBCA safety has garnered renewed interest in recent years. A 2013 report demonstrated that multiple exposures to GBCA in some patients led to increased signal intensity in specific regions of the brain on unenhanced MR images.21 This finding was attributed to the retention of gadolinium compounds in these regions. Since that report, many articles have been published on the topic, with some studies quantifying the levels of gadolinium in different areas of the brains of patients exposed to GBCAs.22-24 These studies confirmed that gadolinium retention occurs to some extent with all GBCAs, regardless of structure or the number of doses administered.22-24 Moreover, gadolinium retention occurs in patients of all ages, with similar distribution and levels in pediatric and adult patients.25
It is important to emphasize that, to date, no reports suggest an association of gadolinium retention with neurotoxicity, even among GBCAs with the highest rates of retention.15 Although the clinical implications of gadolinium retention remain unknown, that GBCAs provide crucial, even lifesaving, information is well established.15
Selecting a GBCA for Pediatric MRI
Carefully weighing the benefits of a contrast-enhanced MRI study versus its risks is vitally important in younger patients, as they may undergo repeat exams over their lifetime. As a general principle, the unnecessary exposure of children to GBCAs should be avoided; when a GBCA is necessary, the lowest dose to achieve a diagnosis should be administered.15 While GBCA selection is guided by its indications and ability to reveal specific structures and pathologies, two key factors, structure and relaxivity, should be considered, as they can influence efficacy and patient safety.
Structure
GBCAs contain the paramagnetic ion gadolinium (Gd3+) bound to a ligand to form a chelate, the structure and ionicity of which influence the overall stability of the chelate-Gd3+ complex.15 Linear nonionic agents demonstrate the lowest stability, whereas linear ionic agents are moderately stable.26 Macrocyclic GBCAs have the highest stability, imparted by a cage-like configuration that allows the chelate to bind Gd3+ more tightly than in linear chelates.26 Macrocyclic agents can be postulated to have a lower potential for gadolinium dissociation from the chelate structure, reducing the risk of NSF development or gadolinium retention.27-32 While human data are limited, animal studies have shown that gadopiclenol behaves similarly to other macrocyclic agents, with less gadolinium retention in tissues compared to linear GBCAs.33,34
With the totality of data supporting the high stability of macrocyclic GBCAs, the shift towards their use in pediatric settings is unsurprising. According to a 2017 survey of children’s hospitals in the US, 80% of respondents reported using macrocyclic GBCAs, with 58% reporting having switched in the prior year. An additional 19% said they were considering a switch to a macrocyclic agent.35
Relaxivity
The ability of GBCAs to increase the relaxation rates of the surrounding water proton spins is quantified in terms of r1 relaxivity.36 An agent with a higher r1 relaxivity creates better contrast between abnormal lesions and background tissues, which can help guide diagnosis and treatment planning.36
Of the six available GBCA molecules, three agents—gadoteridol (ProHance), gadoterate meglumine (Dotarem/Clariscan), and gadobutrol (Gadavist)—demonstrate what may be referred to as standard relaxivity values.5 The remaining three agents all possess unique properties that contribute to higher relaxivity values. Gadobenate dimeglumine (MultiHance) has a hydrophobic side chain that allows it to transiently interact with serum albumin, slowing the tumbling rate, allowing the Gd3+ ion to influence more of the surrounding water protons, and translating into a higher relaxivity.36 The second-higher relaxivity agent, gadoxetate disodium (Eovist), is selectively taken up by hepatocytes for better visualization of liver tissue.8 Gadopiclenol (Vueway/Elucirem) is a macrocyclic agent designed to improve Gd3+ ion access to water molecules, resulting in the highest relaxivity of all GBCAs, even at high magnetic field strengths.6 In clinical studies, a 0.05 mmol/kg dose of gadopiclenol has demonstrated improved diagnostic performance versus conventional GBCAs administered at a standard 0.1 mmol/kg dose while maintaining an acceptable safety profile.37-39
In the first phase 3 study, PICTURE, 0.05 mmol/kg of gadopiclenol demonstrated noninferiority to 0.1 mmol/kg of gadobutrol for all evaluated parameters for imaging central nervous system (CNS) lesions.38 The percentage of enhancement and lesion-to-background ratio were significantly higher for gadopiclenol across all blinded readers, and the contrast-to-noise ratio was significantly higher for two of the three readers. Gadopiclenol-enhanced images were preferred by all readers for nearly half of the evaluations.38 In the second phase 3 study, PROMISE, which enrolled 273 adults with at least one suspected focal lesion in one of three different body regions (head and neck; breast, thorax, abdomen, or pelvis; or musculoskeletal system), 0.05 mmol/kg of gadopiclenol was comparable to mmol/kg gadobutrol for lesion evaluation.39 Despite being administered at half the gadolinium dose of gadobutrol, gadopiclenol provided a comparable degree of contrast enhancement and quality of morphologic characteristics of lesions.39
Pediatric MRI Experience
There are often multiple challenges to obtaining reliable, high-quality images in pediatric imaging. For instance, our practice at Stanford encounters patients of varying weight, ranging from less than two kilograms to more than 100 kilograms. Establishing an MR protocol that optimally delineates anatomy across that range with reliable signal-to-noise ratio (SNR) can be challenging. Using a high- relaxivity agent like gadopiclenol can enable more robust protocols to reliably achieve a higher SNR for better quality images.
Similarly, for various reasons, repeated image acquisition may be required. For instance, children may fidget, resulting in motion artifacts that may necessitate repeat imaging. Moreover, imaging over extremity lengths or other regions with complex air-tissue interfaces may require a second attempt to obtain optimal fat suppression. The higher relaxivity of gadopiclenol may facilitate repeat acquisition without requiring additional contrast dosing (Figure 1).
A significant fraction of pediatric MR exams entails evaluation of multiple body parts. In these cases, administering a 0.1 mmol/ kg dose of gadopiclenol—which delivers the same number of gadolinium atoms as standard dosing of most other GBCAs—in two 0.05 mmol/kg increments can enable high-quality imaging across the multiple body parts (Figure 2). This can be pushed further by splitting a 0.05 mmol/kg dose to maintain high-quality imaging of two body parts (Figure 3). As an alternative example, an evaluation of the liver with gadoxetate disodium and an evaluation of myocardial scarring with gadopiclenol can be combined in a single appointment (Figure 4). This is particularly helpful in patients undergoing MRI under anesthesia, as dividing the exam across multiple days would be impractical.
Another challenging pediatric scenario consists of a comprehensive cardiac MRI assessment of anatomy, blood flow, ventricular function, and myocardial tissue characterization. Here a 0.1 mmol/kg dose of gadopiclenol enables a complete exam within ten minutes in a preteen child (Figure 5) or an infant (Figure 6). In the case of an uncooperative child, that can mean the difference between obtaining a study with sufficient image quality and one without.
A shorter scan with the ability to capture rapid kinetics in children is especially useful in abdominal imaging. Similarly, in angiography exams, the high relaxivity of gadopiclenol can capture the first pass at high spatiotemporal resolution, thus facilitating higher matrix size and thinner slices for improved arterial delineation (Figures 7–9).
Summary Points
The development of new GBCAs continues to advance the diagnostic value of MRI. Given that children may undergo repeat exams over their lifetime, weighing the benefits of contrast with its associated risks is crucial. Below is summary of key considerations for GBCA use in children:
- The unnecessary exposure of children to GBCAs should be avoided; when a GBCA is neces- sary, the lowest dose to achieve a diagnosis should be administered.
- Two physicochemical properties should be considered when selecting a GBCA:
- Stability: Macrocyclic agents demonstrate the highest stability, with a lower propensity for de-chelation and subsequent risk of NSF development or gadolinium retention.
- Relaxivity: A high relaxivity agent demonstrates better diagnostic performance and ability to guide diagnosis and treatment planning than a standard relaxivity agent.
- Gadopiclenol is a new, high-relaxivity GBCA with high diagnostic accuracy and efficacy at a lower dose of 0.05 mmol/kg compared with other conventional GBCAs, thus minimizing safety risks without sacrificing diagnostic outcomes.
References
- Kanal E. Gadolinium based contrast agents (GBCA): safety overview after 3 decades of clinical experience. Magn Reson Imaging. 2016;34(10):1341-1345.
- Anderson MA, Harrington SG, Kozak BM, Gee MS. Strategies to reduce the use of gadolinium-based contrast agents for abdominal MRI in children. AJR Am J Roentgenol. 2020;214(5):1054-1064.
- Heshmatzadeh Behzadi A, McDonald J. Gadolinium-based contrast agents for imaging of the central nervous system: a multicenter European prospective study. Medicine (Baltimore). 2022;101(34):e30163.
- McDonald JS, McDonald RJ, Jentoft ME, et al. Intracranial gadolinium deposition following gadodiamide-enhanced magnetic resonance imaging in pediatric patients: a case-control study. JAMA Pediatr. 2017;171(7):705-707.
- Shen Y, Goerner FL, Snyder C, et al. T1 relaxivities of gadolinium-based magnetic resonance contrast agents in human whole blood at 1.5, 3, and 7 T. Invest Radiol. 2015;50(5):330-338.
- Robic C, Port M, Rousseaux O, et al. Physicochemical and pharmacokinetic profiles of gadopiclenol: a new macrocyclic gadolinium chelate with high T1 relaxivity. Invest Radiol. 2019;54(8):475-484.
- MultiHance (gadobenate dimeglumine) injection. Prescribing information. Bracco Diagnostics Inc.; August 2018.
- Eovist (gadoxetate disodium). Prescribing information. Bayer HealthCare Pharmaceuticals; December 2021.
- ProHance (gadoteridol) injection. Prescribing information. Bracco Diagnostics Inc.; June 2022.
- Gadavist (gadobutrol) injection. Prescribing information. Bayer HealthCare Pharmaceuticals; April 2022.
- Dotarem (gadoterate meglumine). Prescribing information. Guerbet LLC; April 2012.
- Clariscan (gadoterate meglumine) injection. Prescribing information. GE Healthcare Inc.; February 2020.
- VueWay (gadopiclenol) injection. Prescribing information. Bracco Diagnostics Inc.; September 2022.
- Elucirem (gadopiclenol). Prescribing information. Guerbet LLC; September 2022.
- American College of Radiology (ACR) Committee on Drugs and Contrast Media. ACR Manual on Contrast Media, Version 2023. ACR website. https://www. acr.org/-/media/ACR/Files/Clinical-Resources/Contrast_Media.pdf. Accessed December 1, 2023.
- Cowper SE, Robin HS, Steinberg SM, Su LD, Gupta S, LeBoit PE. Scleromyxoedema-like cutaneous diseases in renal-dialysis patients. Lancet. 2000;356:1000–1001.
- Grobner T. Gadolinium—a specific trigger for the development of nephrogenic fibrosing dermopathy and nephrogenic systemic fibrosis? [published correction appears in Nephrol Dial Transplant. 2006;21(6):1745]. Nephrol Dial Transplant. 2006;21(4):1104-1108.
- Shellock FG, Spinazzi A. MRI safety update 2008: part 1, MRI contrast agents and nephrogenic systemic fibrosis. AJR Am J Roentgenol. 2008;191(4):1129-1139.
- Attari H, Cao Y, Elmholdt TR, Zhao Y, Prince MR. A systematic review of 639 patients with biopsy-confirmed nephrogenic systemic fibrosis. Radiology. 2019;292(2):376-386.
- Nardone B, Saddleton E, Laumann AE, et al. Pediatric nephrogenic systemic fibrosis is rarely reported: a RADAR report. Pediatr Radiol. 2014;44(2):173-180.
- Kanda T, Ishii K, Kawaguchi H, Kitajima K, Takenaka D. High signal intensity in the dentate nucleus and globus pallidus on unenhanced T1-weighted MR images: relationship with increasing cumulative dose of a gadolinium-based contrast material. Radiology. 2014;270(3):834-841.
- Kanda T, Fukusato T, Matsuda M, et al. Gadolinium-based contrast agent accumulates in the brain even in subjects without severe renal dysfunction: evaluation of autopsy brain specimens with inductively coupled plasma mass spectroscopy. Radiology. 2015;276(1):228-232.
- McDonald RJ, McDonald JS, Kallmes DF, et al. Intracranial gadolinium deposition after contrast-enhanced MR imaging. Radiology. 2015;275(3):772-782.
- Murata N, Gonzalez-Cuyar LF, Murata K, et al. Macrocyclic and other non-group 1 gadolinium contrast agents deposit low levels of gadolinium in brain and bone tissue: preliminary results from 9 patients with normal renal function. Invest Radiol. 2016;51(7):447-453.
- Stanescu AL, Shaw DW, Murata N, et al. Brain tissue gadolinium retention in pediatric patients after contrast-enhanced magnetic resonance exams: pathological confirmation. Pediatr Radiol. 2020;50(3):388-396.
- Idée JM, Port M, Robic C, Medina C, Sabatou M, Corot C. Role of thermodynamic and kinetic parameters in gadolinium chelate stability. J Magn Reson Imaging. 2009;30:1249-1258.
- Quattrocchi CC, Mallio CA, Errante Y, Beomonte Zobel B. High T1 signal intensity in dentate nucleus after multiple injections of linear gadolinium chelates.Radiology. 2015;276(2):616-617.
- Errante Y, Cirimele V, Mallio CA, Di Lazzaro V, Zobel BB, Quattrocchi CC. Progressive increase of T1 signal intensity of the dentate nucleus on unenhanced magnetic resonance images is associated with cumulative doses of intravenously administered gadodiamide in patients with normal renal function, suggesting dechelation. Invest Radiol. 2014;49(10):685-690.
- Tedeschi E, Palma G, Canna A, et al. In vivo dentate nucleus MRI relaxometry correlates with previous administration of Gadolinium-based contrast agents.Eur Radiol. 2016;26(12):4577-4584.
- Kanda T, Matsuda M, Oba H, Toyoda K, Furui S. Gadolinium deposition after contrast-enhanced MR imaging. Radiology. 2015;277(3):924-925.
- Radbruch A, Weberling LD, Kieslich PJ, et al. High-signal intensity in the dentate nucleus and globus pallidus on unenhanced T1-weighted images: evaluation of the macrocyclic gadolinium-based contrast agent gadobutrol. Invest Radiol. 2015;50(12):805-810.
- Stojanov D, Aracki-Trenkic A, Benedeto-Stojanov D. Gadolinium deposition within the dentate nucleus and globus pallidus after repeated administrations of gadolinium-based contrast agents-current status. Neuroradiology. 2016;58(5):433-441.
- Fretellier N, Rasschaert M, Bocanegra J, et al. Safety and gadolinium distribution of the new high-relaxivity gadolinium chelate gadopiclenol in a rat model of severe renal failure. Invest Radiol. 2021;56(12):826-836.
- Funke SKI, Factor C, Rasschaert M, et al. Long-term gadolinium retention in the healthy rat brain: comparison between gadopiclenol, gadobutrol, and gadodiamide. Radiology. 2022;305(1):179-189.
- Mithal LB, Patel PS, Mithal D, Palac HL, Rozenfeld MN. Use of gadolinium-based magnetic resonance imaging contrast agents and awareness of brain gadolinium deposition among pediatric providers in North America. Pediatr Radiol. 2017;47(6):657-664.
- Kanal E, Maravilla K, Rowley HA. Gadolinium contrast agents for CNS imaging: current concepts and clinical evidence. AJNR Am J Neuroradiol. 2014;35(12):2215-2226.
- Bendszus M, Roberts D, Kolumban B, et al. Dose finding study of gadopiclenol, a new macrocyclic contrast agent, in MRI of central nervous system. Invest Radiol. 2020, 55:129-137.
- Loevner LA, Kolumban B, Hutóczki G, et al. Efficacy and safety of gadopiclenol for contrast-enhanced MRI of the central nervous system: the PICTURE randomized clinical trial. Invest Radiol. 2023;58(5):307-313.
- Kuhl C, Csőszi T, Piskorski W, Miszalski T, Lee JM, Otto PM. Efficacy and safety of half-dose gadopiclenol versus full-dose gadobutrol for contrast-enhanced body MRI. Radiology. 2023;308(1):e222612