One-stop shop cardiovascular imaging
Images
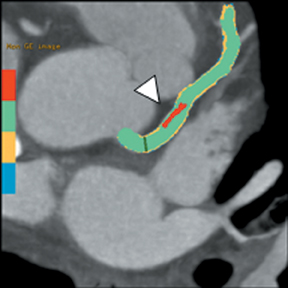
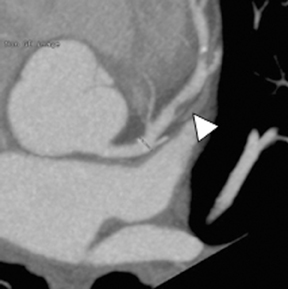
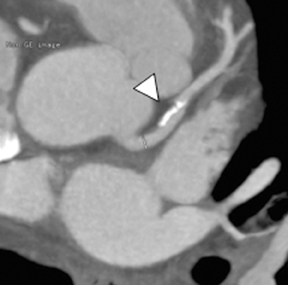
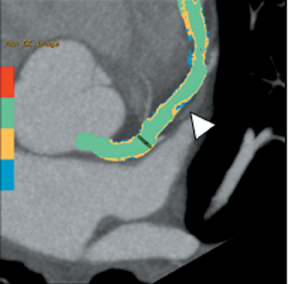
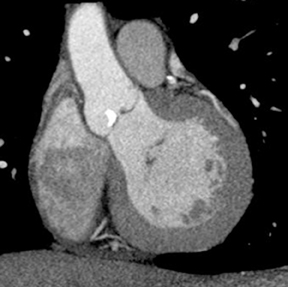
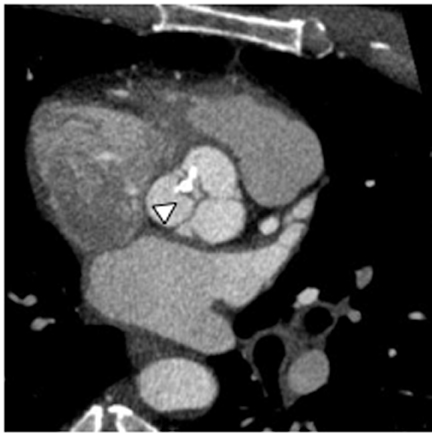
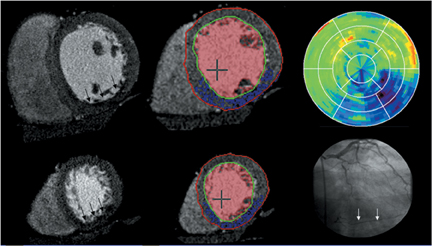
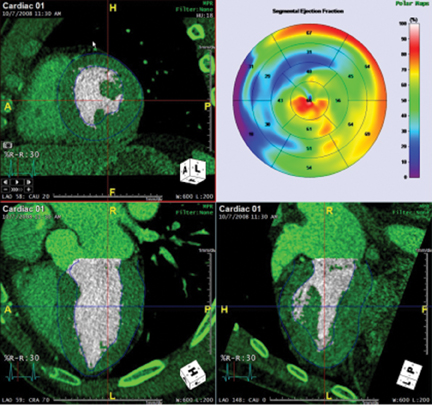
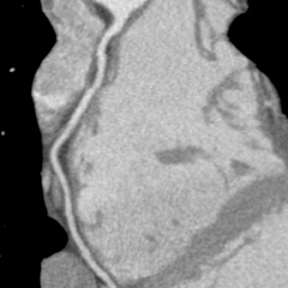
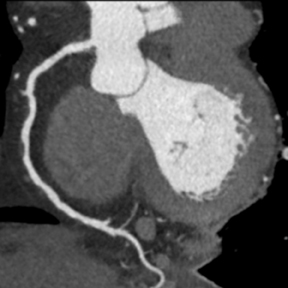
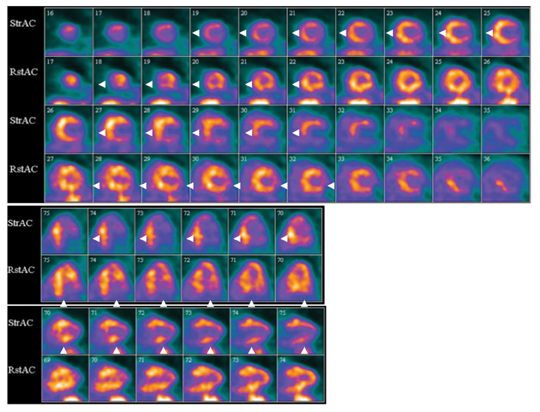
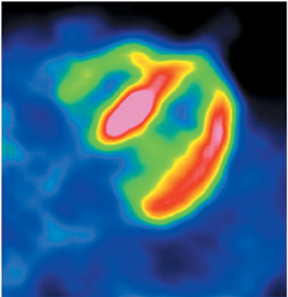
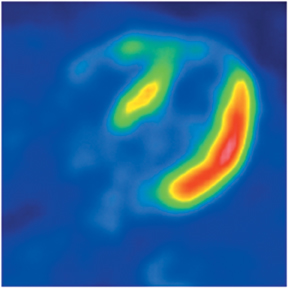
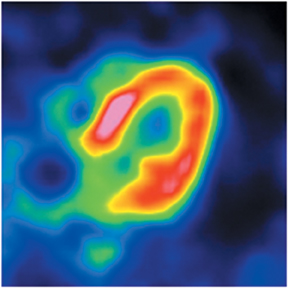
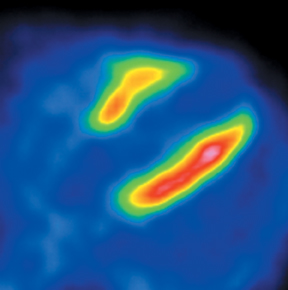
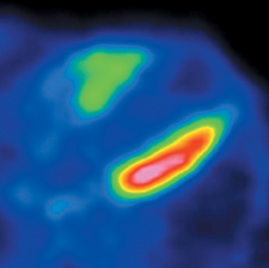
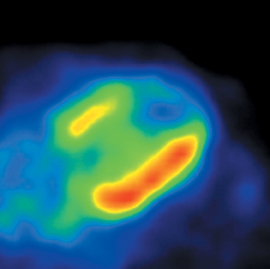
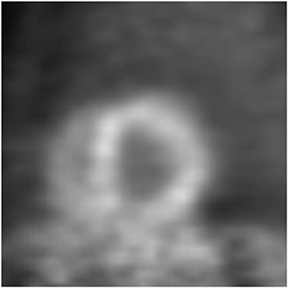
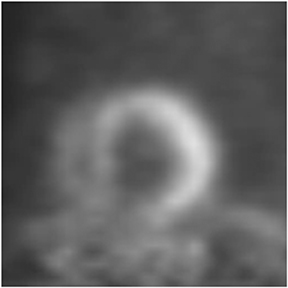
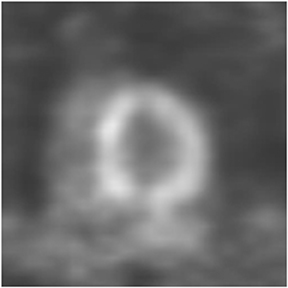
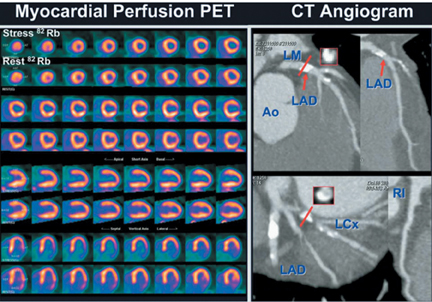
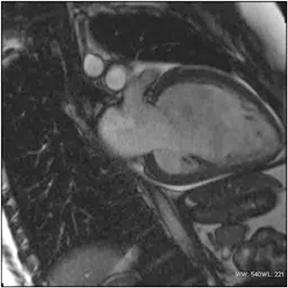
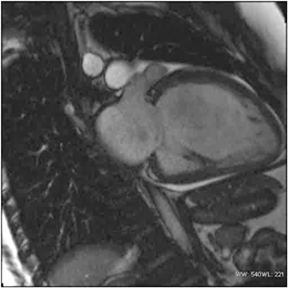
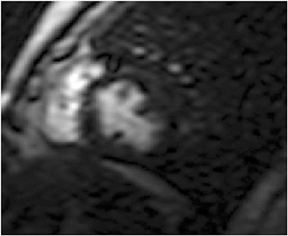
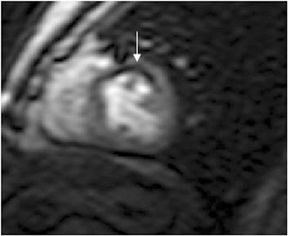
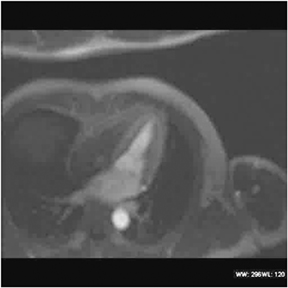
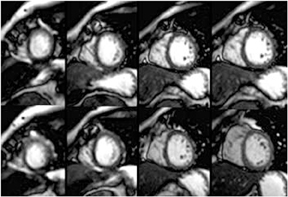
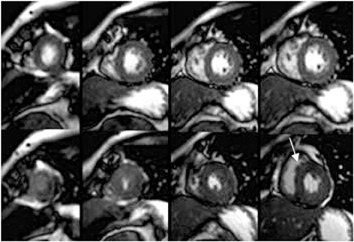
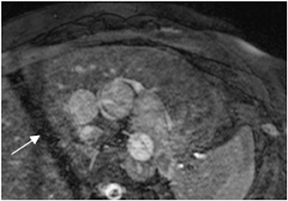
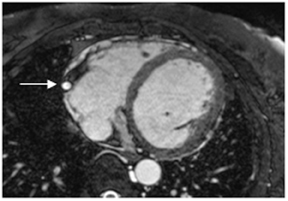
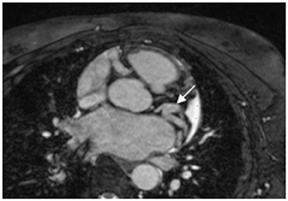
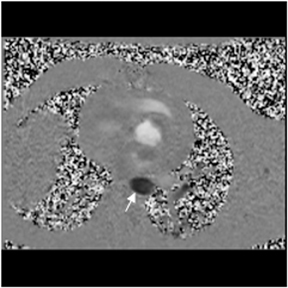
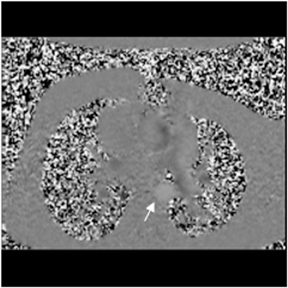
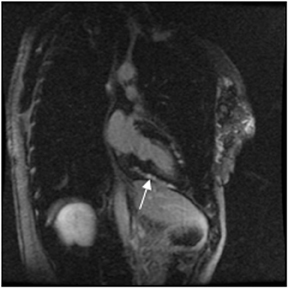
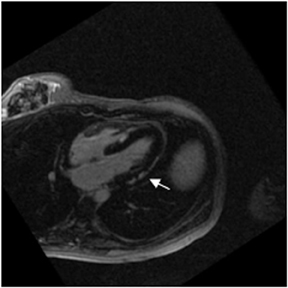
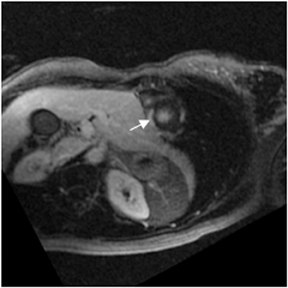
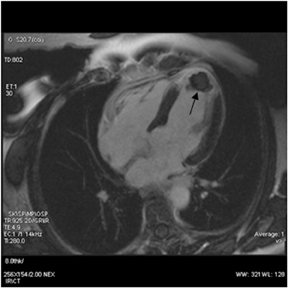
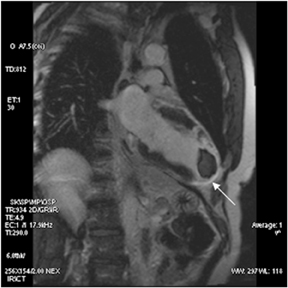
Dr. Zimmerman is a Fellow in Cardiovascular Imaging and Dr. Jha is an Assistant Professor of Radiology at the Hospital of the University of Pennsylvania, Philadelphia, PA.
Disclosure: This work was supported by a grant from General Electric.
Imaging has a clear role to play in establishing diagnosis, predicting prognosis and guiding therapy in patients with symptoms of ischemic or structural heart disease. Several established imaging technologies are available to answer diverse anatomical and physiological questions about the heart.
Traditionally, nuclear scintigraphy with single photon emission computed tomography (SPECT) or echocardiography combined with stress testing (physiological or pharmacological) assesses myocardial reserve (contractile/ perfusion) to detect potential functional impairment secondary to a diseased coronary artery. Coronary catheter angiography (CCA) confirms the precise anatomical nature of the vascular pathology and helps assess suitability for medical or surgical therapy, and, if surgical intervention is indicated, it identifies the type of intervention (angioplasty or bypass) most likely to produce the best outcome. Echocardiography, in addition, imparts information about ventricular and valve function.1
Emerging imaging modalities, including cardiac magnetic resonance (CMR), cardiac computed tomography (CT), and positron emission tomography (PET), are able to investigate the heart just as well and perhaps with greater breadth than more traditional methods mentioned above.2,3 Can CMR provide the requisite information about cardiac structure and function in one diagnostic setting—a one stop shop? A comprehensive single examination of the heart is desirable. It would save the patient multiple different examinations with the potential to provide an early distinction between and contribution of ischemic, non-ischemic, and valvular heart disease to the symptoms. Whereas coronary artery disease, by virtue of its overwhelming prevalence and classic symptomology is more commonly etiologic, clinical presentation occasionally overlaps with valvular heart disease and non-ischemic cardiomyopathy. The resulting diagnostic ambiguity may result in unnecessary coronary catheter angiography—a procedure with the potential for major complications.
The purpose of this article is to review anatomical and physiological questions that arise in patients with suspected heart disease, specifically ischemic heart disease, and to assess the relative strengths and weaknesses of cardiac CT, with and without hybrid PET, and CMR in providing a comprehensive cardiac examination.
Coronary artery disease—functional and anatomical imaging
For patients with suspected coronary artery disease (CAD) the purposes of imaging are to establish the presence of inducible ischemia and depict the coronary artery pathology producing hemodynamic compromise.2 The first goal constitutes functional imaging and the latter anatomical imaging. Anatomical imaging by CCA has necessarily followed functional imaging for 2 reasons; CCA is invasive and carries a small but not insignificant risk of complications.4 A therapeutic benefit of revascularization either by angioplasty, with or without stenting, or bypass grafts, occurs in patients with impaired cardiac function as a consequence of obstructive CAD.5,6 A comprehensive cardiac imaging modality would, at the very least, concurrently provide anatomical and functional information.
Traditionally the diagnosis of CAD has required catheter angiography. Significant disease is defined as left main stenosis >50% or epicardial coronary arteries stenosis >70%.7 Patients without ischemia may not require anatomical imaging. Thus, a negative functional imaging test would not be able to distinguish the patient with sub-clinical atherosclerotic coronary arteries from those with pristine coronary arteries.
Reliance solely on anatomical imaging might predict therapeutic efficacy of revascularization in a patient with obstructive CAD without inducible ischemia in the segment supplied by the diseased coronary artery due to the presence of adequate collateral flow. A corollary is that purely anatomical imaging does not consider cardiac hemodynamics, such as left ventricular-end diastolic pressure, the state of the microcirculation, and the nature of coronary artery stenosis—factors that may diminish the effect of the lesion below a functionally significant threshold.8 Regardless of these permutations, it is increasingly evident that the anatomical and functional coronary tree imaging are complementary not competitive diagnostic components.
Principles of ischemia induction
Functional imaging captures part of the ischemic cascade where progressive duration and severity of ischemia leads to perfusion abnormality, followed by diastolic myocardial dysfunction, followed by systolic myocardial dysfunction, before changes in the electrocardiogram (ECG) are evident.2 All of these developments occur before the appearance of angina and are initially not evident during rest. The goal of functional imaging is to increase myocardial blood flow (stress) by physiological or pharmacological means to uncover the myocardial segments supplied by diseased coronary arteries. Abnormalities in perfusion and systolic wall motion tend to occur with stress when a major coronary artery lumen is narrowed by >70%.8
Broad principles to detect ischemia
Stenotic arteries are already maximally dilated, whereas non-stenotic arteries are still able to respond to a vasodilator (adenosine or dypyridamole). The result is relative ischemia in the distribution of the diseased artery and reduced myocardial flow reserve.
Stenotic arteries are unable to meet the requirements of increased myocardial oxygen demand to the same extent as a non-stenotic artery,resulting in hypokinesis of the myocardial segment supplied by the diseased artery with reduced myocardial contractile reserve. The stress may be induced physiologically (exercise) or pharmacologically (dobutamine).
As perfusion limitations occur before systolic wall motion abnormalities in the ischemic cascade, searching for reduced myocardial flow reserve is a more sensitive method to diagnose hemodynamic compromise than eliciting reduced myocardial contractile reserve. The choice between the two is also influenced by patient co-morbidities. Some caveats to consider are the use of adenosine is contraindicated in asthmatics and left bundle branch block will yield non-diagnostic examinations due to asymmetric septal relaxation, making patients with this abnormality unsuitable for stress testing for wall motion abnormality.2 Dobutamine has the advantage of uncovering hibernating myocardium at low doses. Hibernating myocardium is akinetic because of chronic high-grade ischemia rather than infarction and is likely to respond well to revascularization. Certain musculoskeletal conditions make pharmacological stress preferable over physiological stress.
Most studies for assessing perfusion have been done with SPECT imaging obtained at stress and rest with reported sensitivity of 86% and specificity of 74% using the definition of CCD described above.9 ECG-gated SPECT allows assessment of left ventricular function. Despite widespread experience with SPECT, 2 major limitations remain. First are common and predictable attenuation artifacts that create false-positive results, which can be reduced by correlation with wall motion. The second is false negative results in those with severe 3-vessel disease, creating balanced ischemia where the perfusion defect is global and symmetric, reducing the conspicuity of ischemia in any one segment. Dobutamine stress echocardiography has sensitivity and specificity of 85% and 77%, respectively.10 Echocardiography is occasionally plagued by a sub-optimal acoustic window and drop out of the anterior and lateral walls.
Myocardial viability
Once it is determined that the patient has functional compromise from obstructive CAD, the choice of revascularization is usually straightforward assuming the myocardium is viable. Put another way, there is no point in revascularizing the myocardium segment that is entirely or mostly infarcted (scar tissue), as the chances of functional recovery is minimal. If a scar constitutes <25% of mural thickness,there is a high probability of restoring myocardial segment function with revascularization, but low probability if scarring involves >75% of wall thickness.11 Imaging of myocardial viability is an important, though not routine, assessment in the management of CAD, particularly in patients with a depressed ejection fraction, history of myocardial infarction or bypass grafts or in those presenting with heart failure.
Assessment beyond the coronary arteries
In addition to the anatomical and functional assessment of the coronary arteries, comprehensive cardiac assessment should assess regional and global ventricular function, the valves, and the myocardium for non-ischemic pathology. There are many reasons to assess left ventricular (LV) function qualitatively and to derive ejection fraction (EF) quantitatively. Postrevascularization functional improvement inpatients with an EF <35% exceeds that of patients with a normal or slightly subnormal EF.12 Reduced LV function can be a deciding factor for prophylactic cardiac resynchronization.13 Medical therapy is altered for depressed ejection fraction.
Aortic valve pathology can cause ischemia with a lower atherosclerotic burden.14 Cardiac amyloidosis15 and aortic stenosis1 are known to present with chest pain. Nonischemic cardiac pathology, such as hypertrophic cardiomyopathy, can co-exist with ischemic heart disease. It is important that these entities are uncovered before committing to medical and/or surgical therapy for ischemic disease.
Cardiac CT
Multidetector computed tomography (MDCT) could one day fulfill the requirements of a one-stop shop, providing a comprehensive cardiac imaging evaluation. MDCT’s strength lies in its ability to obtain isotropic, high spatial resolution studies that permit multiplanar evaluation of the coronary arteries and myocardium. In addition, MDCT is rapid to perform, widely available, can accurately assess ventricular function, and often reveals important extracardiac findings. Emerging applications of MDCT include plaque characterization (Figure 1), valve evaluation (Figure 2), myocardial perfusion imaging, and myocardial viability, expanding the utility of MDCT in the work-up of patients with suspected cardiac disease. New wide area detector scanners also have the capacity to perform CT stress perfusion imaging (Figure 3).
The accuracy of MDCT for the diagnosis of CAD is well established. In a meta-analysis by Hamon, et al, of 12 studies comparing 64-detector MDCT to conventional coronary angiography, coronary CT angiography (CCTA) had a sensitivity of 97%, specificity of 90%, and negative predictive value of 96% for stenoses >50% on a per-patient basis.16 Results from a recent multicenter trial involving 9 hospitals in 7 countries, the CORE 64 study, yielded an overall sensitivity of 85%, specificity of 90%, and a negative predictive value of 83% for stenoses>50%17 using coronary arteriography as the reference standard. Because patients already selected for cardiac catheterization were enrolled, subjects in both studies had a high pre-test probability of significant CAD. In a meta-analysis performed by Hamon, et al, the prevalence of CAD ranged from 29% to 90% and was 56% in the CORE 64 trial.16, 17 In the Hamon study, on average only 4% of assessed coronary artery segments were of poor quality, and in the multicenter trial of Miller, et al, 99% of segments were adequate for visual assessment.16, 17
Although CCTA can accurately quantify the degree of luminal narrowing secondary to atherosclerotic plaque, mere quantification ofstenosis may not provide an accurate estimate of coronary risk. Acute myocardial infarctions are frequently associated with lesions composed of noncalcified plaque (vulnerable plaque) that initially cause positive vessel remodeling to a greater extent than luminal stenosis.18 A distinct advantage of CCTA over catheter-based angiography is its ability to assess the arterial wall, as well as the lumen, and characterize noncalcified plaque. The quantification of vulnerability of plaque and differential management based on vulnerability is an area of ongoing research.
CCTA can evaluate LV function and regional wall motion (Figure 4) to assess the physiologic significance of lesions and aid in risk stratification.19 An advantage of MDCT is its ability to provide functional information using the same dataset obtained for the purpose of CTA.There is excellent correlation (r=0.85-0.99) of left ventricular ejection fraction (LVEF) obtained at 16-detector MDCT with CMR, the current reference standard.9 One potential limitation of MDCT is consistent underestimation of LVEF, on the order of 2% to 3%, as compared to other modalities, including 64-detector MDCT.20 This finding is likely related to the lower (82-250 ms) temporal resolution as compared to echocardiography and MRI (50 ms), decreasing resolution in end systole. Another advantage of MDCT for evaluation of ventricularfunction is low inter-observer variability, superior to echocardiography.19
MDCT is accurate for the detection of perfusion deficits in patients with known acute or chronic myocardial infarction.21 The ability of rest MDCT to identify symptomatic perfusion defects in outpatients with suspected CAD and no history of myocardial infarction is less certain. In a study comparing stress CMR and rest MDCT, only 3 of 6 patients with symptoms suggesting CAD and perfusion defects on stress CMR showed matched perfusion defects on rest 16-MDCT.21 However, a recent study demonstrated that resting 64
detector CT imaging will detect perfusion defects in myocardial territories supplied by vessels with >70% stenosis without need for stress
imaging, possibly because of the vasodilatory effect of iodine on coronary arteries.22 Additionally, reduced myocardial perfusion of ischemic myocardium during systole has been demonstrated with resting 64-MDCT and validated against rest-stress 201-Tl perfusion imaging.23
Although stress MDCT examinations are impractical with 4-detector through 64-detector scanners because of temporal inhomogeneity, recent advances in MDCT technology have led to the development of wide-area detector scanners with up to 320 detectors in the z-axis, permitting imaging of the entire heart in one gantry rotation (Figure 5). Wide-area detector scanners have many advantages over traditionalMDCT, such as improved temporal uniformity and elimination of stair step artifacts.24 These improvements result in accurate myocardial perfusion imaging without the limitation of different portions of the myocardium being scanned at different times in relation to the contrastbolus. These advantages have allowed for practical rest-stress perfusion imaging protocols. Typical examinations begin with a diagnostic rest coronary scan followed by adenosine infusion and repeat CT with iodine injection at peak stress. The stress scan must cover two R-R intervals for multisegment reconstruction to be performed, requiring improved temporal resolution at higher heart rates. Preliminary studies have shown good correlation between reversible perfusion defects seen on rest-stress MDCT imaging compared to SPECT studies with a sensitivity of 86% and specificity of 92%.25 The radiation dose for a typical rest-stress CT examination is 21.6 mSv on a 256-row scanner,25 which is still prohibitively high.
MDCT’s ability to assess myocardial viability has been evaluated in animal studies that have verified that delayed enhancement occurs in necrotic myocardial tissue following administration of iodine contrast similar to the mechanism seen with gadolinium-enhanced MRI.26 The ability of MDCT to detect delayed enhancement has been compared to contrast enhanced MRI, the reference standard, with good correlation. Ina study of patients with acute and chronic MI, 34 of 37 patients with delayed enhancement on MRI demonstrated similar findings on MDCT.27 The main limitation of MDCT for viability imaging is poor image quality related to a low contrast-to-noise ratio. In a study by Nieman, et al, the overall contrast between normal and hyperenhanced segments was 29% for MDCT compared to 272% for delayed enhancement MRI.28
An ancillary benefit of the CCTA examination is its ability to provide valuable information on structures beyond the coronary arteries and myocardium without additional imaging time or radiation. MDCT can accurately measure aortic valve area, the key parameter for determining the timing of aortic valve replacement, with high levels of correlation between MDCT and transesophageal/transthoracic echocardiography.29 Assessment of mitral valve area can also be performed with MDCT and shows no significant difference in measurements compared to echocardiography.30 Extracardiac abnormalities are commonly identified by CCTA and have been extensively studied.31 One recent study detected abnormalities in 25% of patients examined, 5% of these were considered major, and included pulmonary embolism and bronchogenic carcinoma.32 CCTA can also provide useful pre-operative information concerning the presence of emphysema, aortic aneurysms, or valvular pathology that may alter surgical management in patients who will undergo revascularization.
Hybrid PET-CT
The strength of cardiac PET lies in its ability to provide highly accurate evaluation of myocardial bloodflow and viability (Figures 6-9). Hybrid PET-CT imaging adds diagnostic coronary arterial angiography to the functional assessment (Figure 10). PET is used in clinical practice to assess myocardial perfusion, quantify myocardial blood flow, or determine myocardial viability. Rubidium-82 and nitrogen-13 ammonia (N-13 ammonia) are the most commonly used radiotracers for evaluating perfusion, while the glucose analogue fluorine-18 deoxyglucose (FDG) is used to assess myocardial viability.Rubidium-82 has the distinct advantage of being generator produced, which obviates the need for an on-site cyclotron, one of the major limitations of N-13 ammonia.
PET is accurate for evaluation of myocardial perfusion in patients with an overall sensitivity and specificity for detection of at least one coronary artery with a >50% stenosis of approximately 90% and 89%, respectively, and with positive and negative predictive values of 94% and 73%.33 PET had higher diagnostic accuracy than SPECT owing to its superior spatial resolution and attenuation correction.33 The superior attenuation correction of PET makes it particularly useful for obese patients.33 Similar to SPECT imaging, PET relies on regional differences in radiotracer uptake to determine the presence of perfusion defects. Therefore, one limitation of PET perfusion imaging is reduced sensitivity for detection of multivessel disease that has resulted in decreased perfusion in multiple myocardial territories.33 A recent study demonstrated only 55% concordance of PET with coronary angiography for patients with multi-vessel disease.34 This limitation can be overcome by taking advantage of the ability of N-13 ammonia PET to directly quantify myocardial blood flow. An absolute decrease in blood flow is more accurate for detecting 3-vessel disease than the typical qualitative imaging methods used in SPECT.35
Myocardial viability imaging is excellent with cardiac FDG PET due to its high metabolic resolution.36 FDG PET evaluates myocardial uptake of glucose, an indicator of metabolism. FDG PET examinations are combined with blood flow imaging. Areas of non-viable myocardium will demonstrate reduced perfusion and metabolism, whereas ischemic but viable tissue will show reduced perfusion and preserved metabolism. One of the main disadvantages of FDG PET is the difficulty of standardization of myocardial glucose uptake, which depends on many factors, including dietary state, cardiac workload, sympathetic drive, and ischemia and can be particularly problematic in diabetic patients.11
Imaging with combined PET-CT systems can increase diagnostic utility by performing CCTA at the conclusion of a PET examination. Clinical PET-CT systems have been developed with up to 64-detector CT coverage, capable of performing diagnostic quality CCTA with all the advantages and disadvantages already described. Adding a coronary CTA to the PET-CT examination has been successful and could potentially provide a one-stopshop solution for perfusion, viability, and anatomic imaging.37 One inherent limitation of the combination of PET and CT angiography is increased radiation. The estimated dose for a combined N-13 ammonia PET-CT with coronary CT angiography is 10-12 mSv.37
Rubidium-82 PET-CT would be expected to have a higher dose because its shorter half-life requires higher amounts of injected activity. An average dose of 13.8 mSv for Rb-82 PET-CT study alone has been reported and an additional CT coronary angiography could be expected to add another 12 mSv for a total of approximately 26 mSv.38, 39 For comparison, estimated dose for a one day rest-stress Tc-99m sestamibi examination is 12 mSv whereas thallium-201 stress and reinjection protocols have a relatively high dose of 25 mSv.40
Combined PET-CT using CT based attenuation correction has several advantages over traditional PET machines including reduced exam time, reduced transmission scan noise, and added anatomic information. However, one potentially significant limitation of PET-CT imaging is misregistration of the PET emission scan with the CT transmission scan.41 This problem stems from the fact that the heart is often in a different location on the rapidly acquired CT transmission scan compared to the PET examination, which is acquired during quiet respiration over a much longer time. Misregistration of cardiac position can result in artifactual defects in myocardial perfusion. In one study, misregistration was worse in the anterior and anterolateral segments and occurred in up to 20% of patients.41 Further work is clearly necessary to optimize CT-based attenuation correction for these studies.
Cardiac MR (CMR)
A combination of factors has improved the ability of MRI to assess the heart’s structure and function. CMR had been plagued more than the imaging of other body regions by the compromises that need to be made among spatial resolution, temporal resolution, signal-to-noise,and acquisition time.42 However, improvements in gradients (strength, slew rate, and rise time), development of parallel imaging techniques,and novel k-space short cuts have enabled studies to be achieved in reasonable time. The inherent advantages of MRI, notably excellent soft tissue contrast (Figure 11) and multiplanar capabilities, have now been realized in the cardiovascular system. MRI is operator-dependent as echocardiography and does not produce artifacts related to body habitus as does nuclear SPECT. CMR can assess contractility and perfusion reserve with dobutamine and adenosine/dypyridamole, respectively.43, 44 Physiological exercise-induced stress testing is cumbersome, but has been performed with creative room design.
First pass perfusion with rapid passage of gadolinium requires high temporal and spatial resolution, along with rapid k-space coverage with T1 weighting (Figures 12 and 13). To achieve this trilogy of goals, the pulse sequence utilizes a saturation recovery prepulse to tip the longitudinal magnetization by 90 degrees (to allow differences in T1 recovery times to manifest), followed by a low flip angle gradient echo,and a single-shot read out. Echoplanar and balanced steady-state free precession sequences have also been used. Perfusion may be tested under both stress and rest conditions or only with stress. Rest perfusion is performed to distinguish between a reversible subendocardial perfusion defect (present only at stress) and artifact secondary to concentrated gadolinium (a defect present at both stress and rest). An alternative explanation for a rest defect is a region of nonreversible perfusion. In this situation there are two explanations, an infarct or hibernating myocardium. These are distinguished by inversion recovery myocardial delayed enhancement imaging. If the myocardium is hibernating,there is no enhancement.
Studies have shown that perfusion CMR at 1.5 Tesla (T) is comparable, if not superior, to nuclear SPECT in revealing myocardial segments supplied by arteries with critical stenosis with sensitivity of 91% and specificity of 81%.43 These statistics are likely to be amplified at 3T, which has inherent signal advantages. Importantly, CMR is not qualitatively dependent on regional differences in signal and can pick upbalanced ischemia of triple vessel disease and coronary vasospasm. Functional cine imaging comparing wall motion at rest and under stress with dobutamine unmasks hibernating myocardium at low doses. Regional wall-motion abnormality manifested at higher dobutamine doses indicates that the artery that supplies the dysfunctional wall has a critical stenosis (Figure 14). Dobutamine stress MRI has a sensitivity of 83% and specificity of 86%.43
Reliable assessment of small caliber coronary arteries that are tortuous and surrounded by subepicardial fat with isotropic resolution using a single breath hold is the ultimate goal of coronary MRA. The absence of iodinated contrast and ionizing radiation makes this an attractive clinical alternative to CCA to exclude CAD. Imaging starts with multislice 2-dimensional gradient echo sequences, progressing to slab specific 3-dimensional sequences targeted to capture the course of the coronary arteries utilizing the fewest partitions. Whole-heart 3-dimensional coronary MRA commonly uses respiratory synchronization or gating by means of a navigator pulse (Figure 15),the fidelity of which is highly dependent on the consistency of the respiratory pattern.45 This method can add considerably to scan time. The approach generally utilizes gradient echo sequences. Formerly, spoiled gradient echo took advantage of flow-related enhancement to provide arterial imaging (bright-blood coronary MRA). Currently, balanced steady-state free precession sequences (b-SSFP) are used in which blood signal is intrinsically bright because of its T2/T1 ratio. Fat is also bright on this sequence, so some form of fat suppression is mandatory. A T2 prepulse suppresses signal from veins and is particularly useful for visualizing the circumflex artery as the great cardiac vein travels in close proximity. A limitation of bright-blood coronary MRA is assessment of coronary calcification. The signal from calcium is unpredictable in MR and depends on the degree of water chelation with the ion. Black-blood coronary MRA can assess the coronary wall and give an indication of plaque burden, which causes positive as well as negative remodeling. Evaluation of plaque is an exciting area of ongoing research, based on the quantification of vulnerable plaque. How this will alter patient management remains to be seen.
Coronary MRA has been shown to have high negative predictive value for excluding CAD in the proximal coronary arteries. A recent meta-analysis shows that the sensitivity and specificity of detection of CAD using CCA as a reference standard is 73% and 86%. However, the studies analyzed varied considerably in test characteristics.46 It would be fair to say that coronary MRA is a promising but evolving technology as an alternative to CCA. It may be added to the comprehensive evaluation of the heart, but reliance solely on this aspect of the examination may be premature. Further improvement in coronary MRA is expected with the development of intravascular contrast agents and the use of 3T magnets.
The use of b-SSFP for functional imaging has tremendously improved the accuracy of CMR assessment of ventricular function. This sequence has an advantage over spoiled-gradient echo, its predecessor, by an intrinsically high signal-to-noise ratio that is augmented by a short repetition time. The sequence can withstand reduction in the SNR due to parallel imaging without compromising the blood-endocardial border. Also, since the high signal of blood is due to its intrinsic properties, it is not reduced in
the face of turbulent flow, such as might occur towards the base of the left ventricle. Indeed, b-SSFP has made CMR the reference standard for ventricular function and volumes.47 Nonetheless, the sequence is susceptible to variation in the local magnetic field that may disrupt the balancing of gradients. This is particularly germane to the heart as it is surrounded by lung. Regular shimming and use of the shortest TR possible reduces inhomogeneity. The sensitivity of functional imaging to early signs of myocardial sickness can be increased by use of grid tagging or strain imaging.42
CMR provides excellent evaluation of the valves both qualitatively and quantitatively (Figure 16). Phase velocity imaging uses a bipolar gradient and measures phase shifts incurred by moving blood to provide information on flow and peak velocity. By measuring the peak velocity, the pressure gradient across a stenotic valve may be determined, which allows the quantification of the degree of valvular stenosis.48 Finally, CMR can uncover a nonischemic explanation of myocardial dysfunction. The most valuable sequence is the inversion-recovery prepped myocardial delayed enhancement (MDE). Nonischemic cardiomyopathies have a scar pattern that does not conform to a coronary artery territory and may spare the subendocardium (Figure 17).49
The use of MDE in identifying ischemic insult is important for guiding therapy and determining prognosis. Myocardial segments that are akinetic/hypokinetic but which have no scar or scar occupying <50% of transmural tissue respond favorably to revascularization. The success of CABG/angioplasty diminishes as the transmurality of the scar exceeds 50%. Thus, MDE can direct appropriate revascularization to the coronary artery most likely to benefit and obviate futile intervention (Figure 18).50
Conclusion
A comprehensive cardiac evaluation is desirable from both clinical and economic perspectives. The latter is underscored by the center-stage occupied by cardiac imaging in the overall growth of medical imaging costs. Studies have shown that the rate of coronary angioplasty seems to correlate with the number of coronary angiograms performed. Additionally, a significant proportion of patients who undergo revascularization have not had functional imaging, that is, the decision to revascularize has been solely based on anatomical imaging by CCA.Clearly this practice could lead to inappropriate revascularization in the absence of inducible ischemia.
CMR provides excellent evaluation of ventricular function, myocardial reserve, and assessment of myocardial viability. The technique assesses valve function and the presence and contribution of nonischemic pathology. Its Achilles’ heel appears to be the evaluation of the coronary artery, with a long distance to go before it can replace catheter angiography. MRI must achieve the spatial resolution of 64-detectorCTA with acquisition of 3-dimensional datasets in a single breath hold. For a patient in heart failure with history of myocardial infarction or nonischemic cardiomyopathy, CMR, by virtue of high soft-tissue contrast and scar detection, is undoubtedly the most appropriate initial study.
Cardiac CT has a high negative predictive value for CAD based on its assessment of the coronary artery lumen, which occurs with concomitant assessment of the arterial wall for plaque characteristics. CT can assess ventricular function with high interobserver reproducibility. The evaluation of myocardial viability remains an evolving technology. Further validation of CT perfusion with wide detector scanners is required, although initial results are promising. Routine use of CTA without a functional component could produce many false positive results, particularly in patients with increased coronary calcium accentuating the problem with anatomical imaging only. The major disadvantage of CT and PET-CT is the radiation dose that increases with the comprehensiveness of the cardiac examination. The metabolic advantage of cardiac PET is unquestionable in the assessment of myocardial viability and coronary blood flow, although a separate scan forCTA and PET components defeats the concept of a one-stop shop.
It is unlikely in the foreseeable future that cardiac catheterization will be out of the diagnostic loop, particularly in patients that are candidates for revascularization. In such a situation, an argument can be made that the modality that offers the best functional and viability information is the one-stop shop of choice.
REFERENCES
- Lee TH, Boucher CA. Clinical practice: noninvasive tests in patients with stable coronary artery disease. N Engl J Med. 2001;344:1840-1845.
- Schuijf JD, Shaw LJ, Wijns W, et al. Cardiac imaging in coronary artery disease: Differing modalities. Heart. 2005; 91:1110-1117.
- Hossein Jadvar, H. William Strauss, and George M. Segall, SPECT and PET in the evaluation of coronary artery disease. Radiographics. 1999; 19:915-926.
- Lozner EC, Johnson LW, Johnson S, et al. Coronary arteriography 1984-1987: a report of the Registry of the Society for Cardiac Angiography and Interventions. II. An analysis of 218 deaths related to coronary arteriography. Cathet cardiovasc diagn. 1989; 17:11-14.
- Hachamovitch R, Berman DS, Shaw LJ, et al. Incremental prognostic value of myocardial perfusion single photon emission computed tomography for the prediction of cardiac death: differential stratification for risk of cardiac death and myocardial infarction. Circulation. 1998;97:535-543.
- Shaw LJ, Berman DS, Maron DJ, et al. Optimal medical therapy with or without percutaneous coronary intervention to reduce ischemic burden: results from the Clinical Outcomes Utilizing Revascularization and Aggressive Drug Evaluation (COURAGE) trial nuclear substudy. Circulation. 2008;117:1283-1291.
- Chaitman BR, Bourassa MG, Davis K, et al. Angiographic prevalence of high-risk coronary artery disease in patient subsets (CASS). Circulation. 1981;64:360-367.
- Di Carli M, Czernin J, Hoh CK, et al. Relation among stenosis severity, myocardial blood flow, and flow reserve in patients with coronary artery disease. Circulation. 1995;91:1944-1951.
- Underwood SR, Anagnostopoulos C, et al. Myocardial perfusion scintigraphy: the evidence. Eur J Nucl Med Mol Imaging. 2004;31:261-291.
- Fleischmann KE, Hunink MG, Kuntz KM, Douglas PS. Exercise echocardiography or exercise SPECT imaging? A meta-analysis of diagnostic test performance. J Nucl Cardiol. 2002; 9:133-134.
- Camici PG, Prasad SK, Rimoldi OE. Stunning, hibernation, and assessment of myocardial viability. Circulation. 2008;117:103-114.
- Cortigiani L, Picano E, Landi P, et al. Value of pharmacologic stress echocardiography in risk stratification of patients with single-vessel disease: a report from the Echo-
- Persantine and echo-Dobutamine International Cooperative Studies. J Am Coll Cardiol. 1998;32:69-74.
- Bristow MR, Saxon LA, Boehmer J, et al. Cardiac-resynchronization therapy with or without an implantable defibrillator in advanced chronic heart failure. N Engl J Med. 2004;350:2140-2150.
- Shahbudin H. Rahimtoola. Aortic Valve Disease. In: Hurst The Heart Manual of Cardiology. 12th ed. New York: McGraw-Hill; 2007: Chapter 75.
- Nihoyannopoulos P. Amyloid heart disease. Current Opin Cardiol. 1987; 2: 371-376.
- Hamon M, Morello R, Riddell JW, Hamon M. Coronary arteries: diagnostic performance of 16- versus 64-section spiral CT compared with invasive coronary angiography-meta-analysis. Radiology. 2007; 245:720-731.
- Miller JM, Rochitte CE, Dewey M, et al. Diagnostic performance of coronary angiography by 64-row CT. N Engl J Med. 2008;359:2324-2336.
- Kelly JL, Thickman D, Abramson SD, et al. Coronary CT angiography findings in patients without coronary calcification. AJR Am J Roentgenol. 2008;191:50-55.
- Cury RC, Nieman K, Shapiro MD, et al. Comprehensive assessment of myocardial perfusion defects, regional wall motion, and left ventricular function by using 64-section multidetector CT. Radiology. 2008;248:466-475.
- Sugeng L, Mor-Avi V, Weinert L, et al. Quantitative assessment of left ventricular size and function: side-by-side comparison of real-time three-dimensional echocardiography and computed tomography with magnetic resonance reference. Circulation. 2006; 114:654-661.
- Nikolaou K, Sanz J, Poon M, et al. Assessment of myocardial perfusion and viability from routine contrast-enhanced 16-detector-row computed tomography of the heart: preliminary results. Eur Radiol. 2005;15:864-871.
- Kachenoura N, Mor-Avi V, Gaspar T, et al. Combined assessment of coronary anatomy and myocardial perfusion using multidetector computed tomography for the evaluation of coronary artery disease: Validation against invasive coronary angiography (abstr). In: Radiological Society of North America scientific assembly and annual meeting program. 2008.
- Nagao M, Matsuoka H, Kawakami H, et al. Quantification of myocardial perfusion by contrast-enhanced 64-MDCT: characterization of ischemic myocardium. AJR Am J Roentgenol. 2008;191:19-25.
- Kitagawa K, Lardo AC, Lima JA, George RT. Prospective ECG-gated 320 row detector computed tomography: implications for CT angiography and perfusion imaging. Int J Cardiovasc Imaging: 2009; in press.
- George RT, Arbab-Zadeh A, Miller LM, et al. Adenosine stress 64- and 256-row detector computed tomography angiography and perfusion imaging: A pilot study evaluating the transmural extent of perfusion abnormalities to predict atherosclerosis causing myocardial ischemia. Circ Cardiovasc Imaging. 2009;2:174-182.
- Lardo AC, Cordeiro MA, Silva C, et al. Contrast-enhanced multidetector computed tomography viability imaging after myocardial infarction: characterization of myocyte death, microvascular obstruction, and chronic scar. Circulation. 2006;113:394-404.
- Gerber BL, Belge B, Legros GJ, et al. Characterization of acute and chronic myocardial infarcts by multidetector computed tomography: comparison with contrast-enhanced magnetic resonance. Circulation. 2006;113:823-833.
- Nieman K, Shapiro MD, Ferencik M, et al. Reperfused myocardial infarction: contrast-enhanced 64-Section CT in comparison to MR imaging. Radiology. 2008; 247:49-56.
- LaBounty TM, Sundaram B, Agarwal P, et al. Aortic valve area on 64-MDCT correlates with transesophageal echocardiography in aortic stenosis. AJR Am J Roentgenol. 2008;191:1652-1658.
- Messika-Zeitoun D, Serfaty J, Laissy J, et al. Assessment of the mitral valve area in patients with mitral stenosis by multislice computed tomography. J Am Coll Cardiol. 2005;48:411-413.
- Colletti PM. Incidental Findings on cardiac imaging. AJR Am J Roentgenol. 2008; 191:882- 884.
- Haller S, Kaiser C, Buser P, Bongartz G, Bremerich J. Coronary artery imaging with contrast-enhanced MDCT: Extracardiac Findings. AJR Am J Roentgenol. 2006;187:105-110.
- Di Carli MF, Dorbala S, Meserve J, et al. Clinical myocardial perfusion PET/CT. J Nucl Med. 2007;48:783-793.
- Sampson UK, Dorbala S, Limaye A, Kwong R, Di Carli MF. Diagnostic accuracy of rubidium-82 myocardial perfusion imaging with hybrid positron emission tomography/computed tomography in the detection of coronary artery disease. J Am Coll Cardiol. 2007;49:1052-1058.
- Parkash R, deKemp RA, Ruddy TD, et al. Potential utility of rubidium 82 PET quantification in patients with 3-vessel coronary artery disease. J Nucl Cardiol. 2004; 11:440-449.
- Schelbert HR. 18F-deoxyglucose and the assessment of myocardial viability. Semin Nucl Med. 2002;32:60-69.
- Namdar M, Hany TF, Koepfli P, et al. Integrated PET/CT for the assessment of coronary artery disease: a feasibility study. J Nucl Med. 2005;46:930-935.
- Sampson UK, Dorbala S, Limaye A, Kwong R, Di Carli MF. Diagnostic accuracy of rubidium-82 myocardial perfusion imaging with hybrid positron emission tomography/computed tomography in the detection of coronary artery disease. J Am Coll Cardiol. 2007;49:1052-1058.
- Hausleiter J, Meyer T, Hermann F, et al. Estimated radiation dose associated with cardiac CT angiography. JAMA. 2009;301:500-507.
- Thompson RC, Ullom SJ. Issues regarding radiation dosage of cardiac nuclear and radiography procedures. J Nucl Cardiol. 2006;13:19-23.
- Martinez-Moller A, Souvatzoglou M, et al. Artifacts from misaligned CT in cardiac perfusion PET/CT studies: frequency, effects, and potential solutions. J Nucl Med. 2007; 48:188-193.
- Reeder SB, Du YP, Lima JA, Bluemke DA. Advanced cardiac MR imaging of ischemic heart disease. Radiographics. 2001; 21:1047-1074.
- Kiran R. Nandalur M , Ben A, et al. Diagnostic performance of stress cardiac Magnetic Resonance Imaging in the detection of coronary artery disease: A meta-analysis. J Am Coll Cardiol. 2007;50:1343-1353.
- al-Saadi N, Nagel E, Gross M, et al. Noninvasive detection of myocardial ischemia from perfusion reserve based on cardiovascular magnetic resonance. Circulation. 2000; 101:1379-1383.
- N.M.C. So, W.W.M. Lam, D. Li, A.K.Y. Chan, J.E. Sanderson and C. Metreweli, Magnetic resonance coronary angiography with 3D TrueFISP: breath-hold versus respiratory gated imaging. Br J Radiol. 2005;78:116-121.
- Danias PG, Roussakis A, Ioannidis JPA. Diagnostic performance of coronary magnetic resonance angiography as compared against conventional X-ray angiography. J Am Coll Cardiol. 2004;44:1867-1876.
- Moon JC, Lorenz CH, Francis JM, Smith GC, Pennell DJ. Breath-hold FLASH and FISP cardiovascular imaging: left ventricular volume differences and reproducibility. Radiology. 2002; 223:789-797.
- LotzJ, Meier C, Leppert A, Galanski M. Cardiovascular flow measurement with phase-contrast MR imaging: basic facts and implementation. Radiographics. 2002;22:651-671.
- Cummings KW, Bhalla S, Javidan-Nejad C, et al. A pattern-based approach to assessment of delayed enhancement in nonischemic cardiomyopathy at MR imaging. Radiographics. 2009;29:89-103.
- Kim RJ, Wu E, Rafael A, et al. The use of contrast-enhanced magnetic resonance imaging to identify reversible myocardial dysfunction. N Engl J Med. 2000;343:1445-1453.