Fetal MRI in the Third Dimension
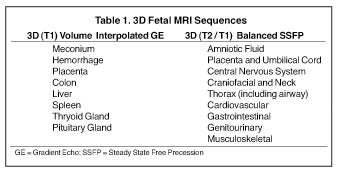
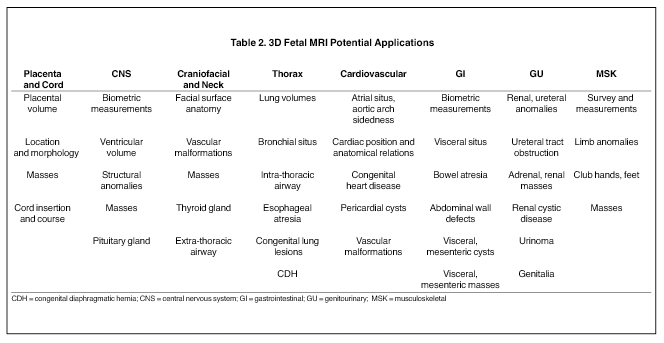
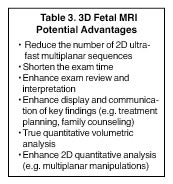
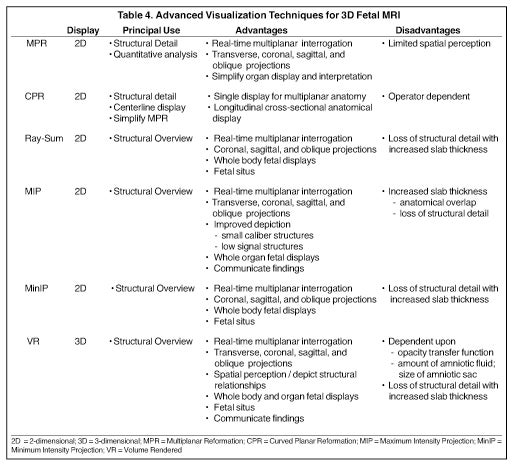
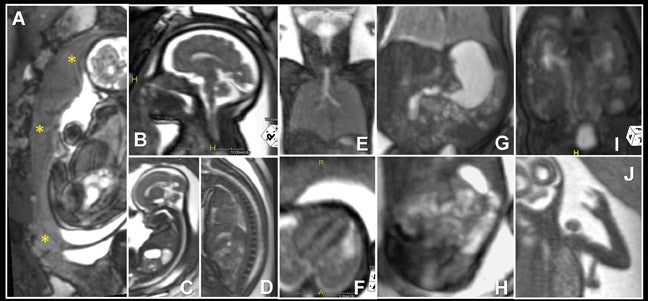
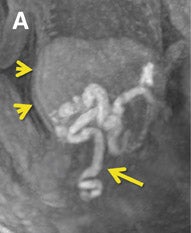
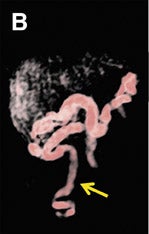
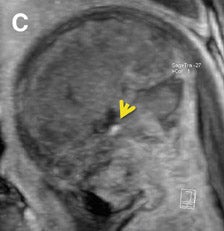
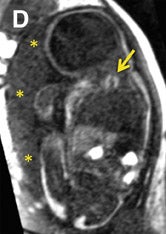
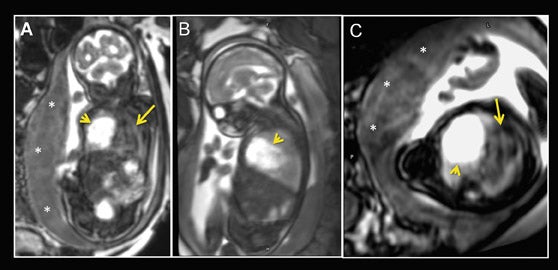
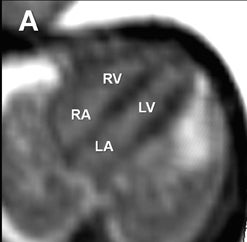
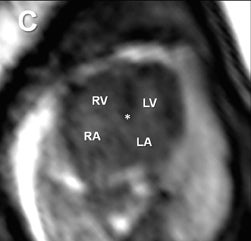
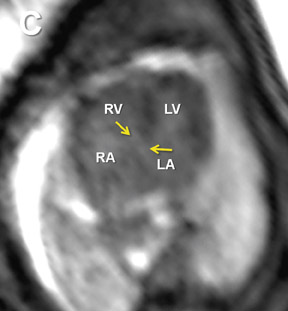
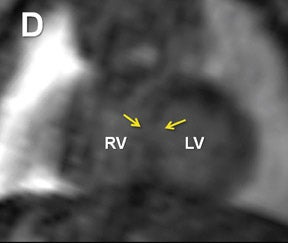
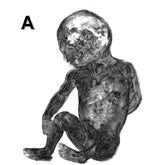
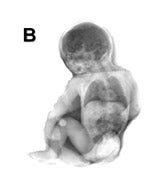
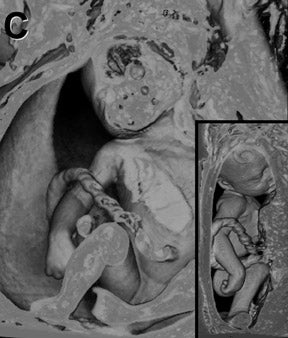
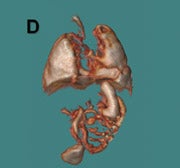
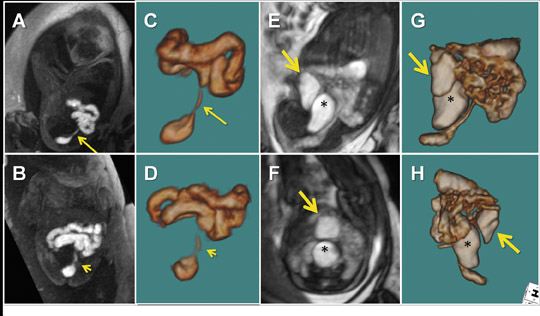
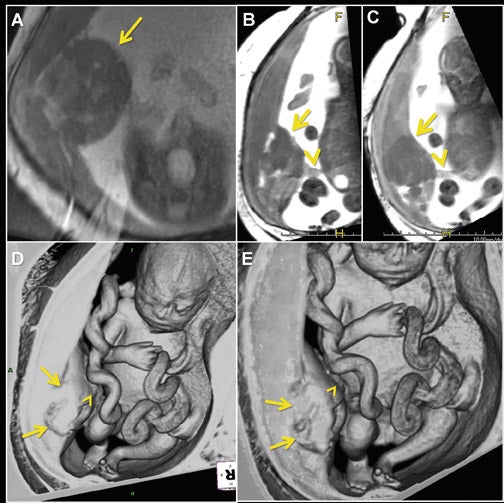
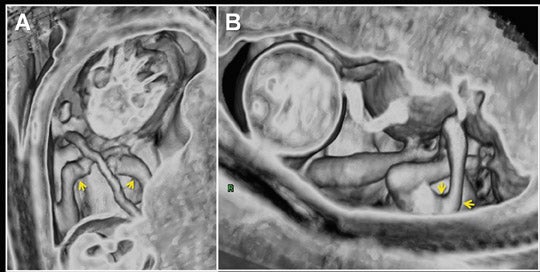
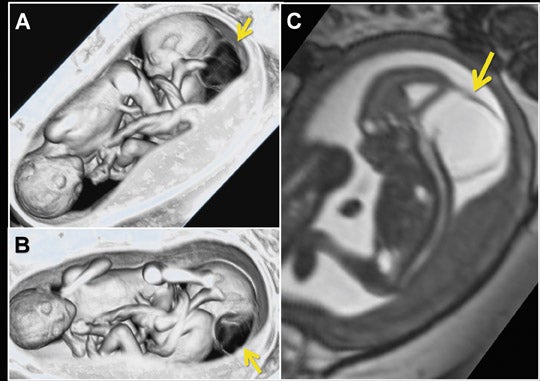
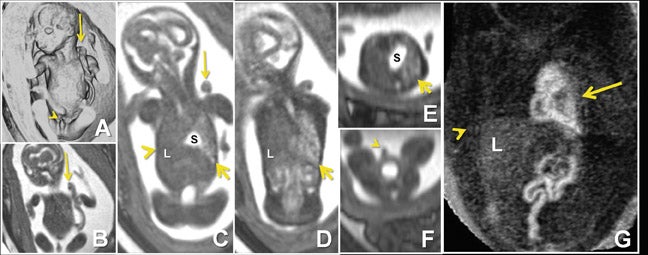
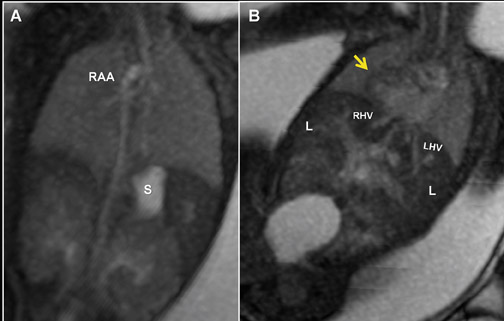
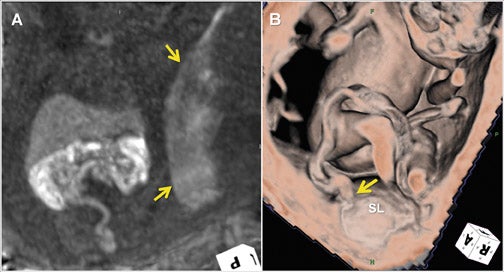
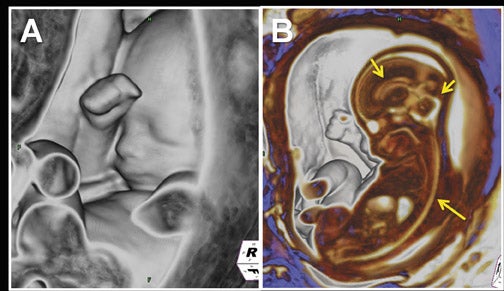
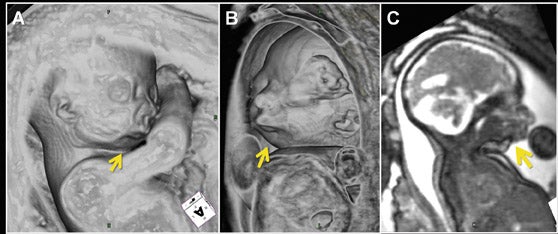
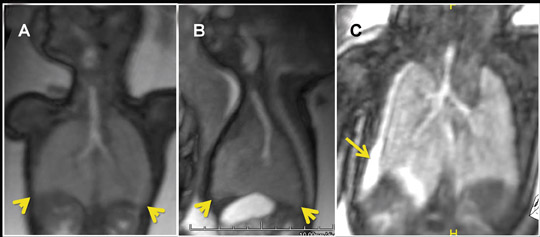
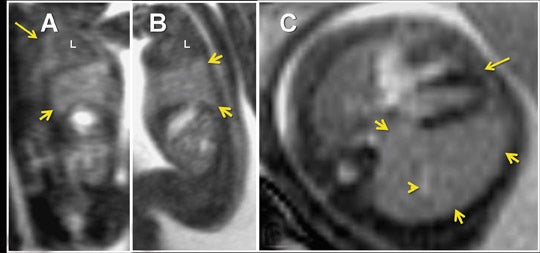
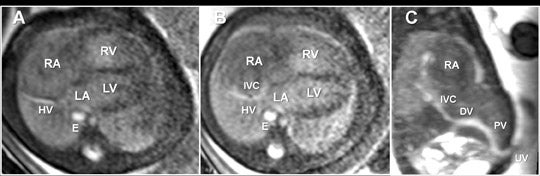
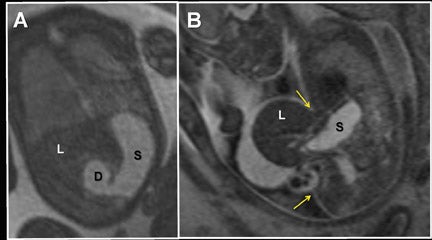
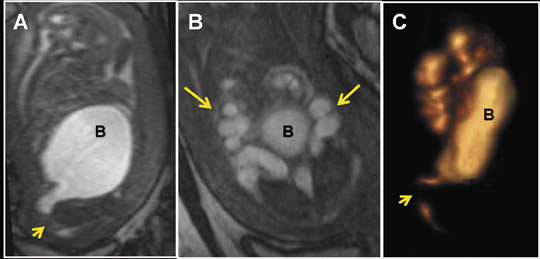
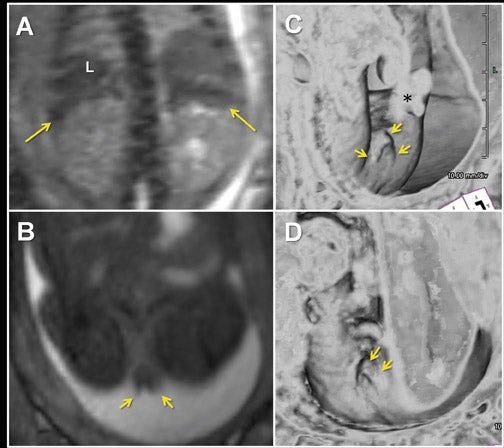
Dr. Hellinger is Associate Professor of Radiology and Pediatrics and Director of the Advanced Imaging and Informatics Laboratory, Departments of Radiology and Pediatrics, Stony Brook Long Island Children’s Hospital, Stony Brook University School of Medicine, Stony Brook, NY; and Dr. Epelman is Assistant Professor of Radiology, Director of Neonatal Imaging, Department of Radiology, The Children’s Hospital of Philadelphia, University of Pennsylvania School of Medicine, Philadelphia, PA.
Diagnostic fetal imaging is an essential requirement for pre- and post-natal care and outcome. Imaging may be applied for screening,targeted evaluations and surveillance of fetal abnormalities. Ultrasound (US) historically has been and remains the principle obstetrical imaging modality. It is readily performed in outpatient and inpatient settings at minimal inconvenience to the mother, yielding reliable information for immediate clinical counseling and management. Initial sonographic screening of all body systems occurs between 20 and 24 weeks. Standard protocols are performed with 2-dimensional grayscale and Doppler static and cine techniques to generate structural and functional information. Inherent limitations include operator and acoustic window dependence. In addition, interpretation is based upon a summation of the images and cine loops from multiple real-time projections. Three-dimensional ultrasound has gained increased application over the past several years, improving structural display1 and affording volumetric quantitative analysis.2 Clinical benefit in diagnosis, treatment planning, and family counseling has been reported for evaluation of facial abnormalities, neural tube defects, skeletal defects,congenital heart disease and the central nervous system1 as well as fetal biometry.3 With improved operator experience, clinical workflow is enhanced.4
Magnetic resonance imaging (MRI) has become an important secondary obstetrical modality for both the fetus and the mother. Compared with ultrasound, it offers superior spatial resolution and structural detail, affording comprehensive anatomical displays from single acquisitions. The high cost, potentially long exam time in a typical closed bore unit, and the limited ability to perform comprehensive functional evaluations, however, relegates MRI to an adjunctive role, after ultrasound has been performed. MRI is often requested to provide more definitive anatomical understanding and diagnosis for abnormalities identified on the screening ultrasound,as well as to assess for concomitant abnormalities.5 The literature has also advocated its use for anatomical screening in the setting of congenital heart disease (CHD)6 and predisposed families.7
Standard MRI protocols utilize high resolution, ultra-fast static and dynamic cine 2-dimensional T1- and T2-weighted (T1W and T2W) sequences.8,9 Using 2-dimensional MRI, multiple acquisitions, in multiple planes are often required to ensure complete anatomical coverage. The number of acquisitions and the exam time are directly dependent upon fetal movement, with the total number of sequences often ranging between 20 to 25, yielding an average exam time of 30 to 55 min. As with ultrasound, interpretation is often based upon mental reconstruction of the anatomy and pathology from the multiple acquisitions; communication, treatment planning and family counseling frequently require displaying multiple sequences.
Three-dimensional MRI sequences can also be acquired through the gravid pelvis (Table 1, Figures 1 and 2) with potentially broad clinical applications (Table 2). These volumetric fetal sequences enable maternal, fetal, placental and cord review from any projection without loss of spatial or visual detail. A single acquisition can be reconstructed into diagnostic quality transverse, coronal, sagittal or oblique sections, relative to the fetus. Alternatively, the sequence can be interrogated real-time in any plane on an imaging workstation using flexible, advanced visualization techniques (Figure 1). Depending upon the image quality, the 3-dimensional sequences may potentially reduce or obviate the need for similar 2-dimensional sequences, shortening the overall exam time and improving patient throughput and satisfaction. Similarly, they may also enhance exam review, improving diagnostic confidence and interpretation workflow. Image display is simplified for communication, treatment planning and family counseling, as a single high-quality 3-dimensional sequence, in conjunction with workstation visualization techniques, may depict all key findings, rather than relying upon multiple 2-dimensional sequences. There is also the potential for greater confidence in planning fetoscopic interventions. Additional advantages of 3-dimensional fetal MRI (FMRI), include true volumetric quantitative analyses (e.g. total lung and fetal body volumes) and enhanced fetal biometry analyses (e.g. multiplanar manipulations, Table3).10
Technique of image acquisition
Clinical utilization of FMRI sequences have 2 essential requirements: 1) fast temporal resolution to account for fetal movement; 2) high, tissue signal-to-noise ratio (SNR), given the inability to administer gadolinium-based contrast agents. Of the commercially available 3-dimensional MRI sequences, 2 meet these criteria. One is gradient echo imaging with balanced steady state preservation of residual transverse magnetization (steady state free precession, SSFP).11 The other is volume interpolated (VI) gradient echo imaging.12 Three-dimensional SSFP (Figure1) is applied to depict fluid-sensitive material and structures, including amniotic fluid, placenta, cord, brain, ventricles, spinal canal, airway, lungs, pleural space, heart, aorta, kidneys, ureters and bladder. When using this sequence, the relative T2/T1 hyperintensity provides high tissue contrast between the viscera, placenta and amniotic fluid. Three-dimensional SSFP is also useful for depiction of the bowel, liver, spleen, the spine and extremities. Three-dimensional VI (Figure 2) is useful for depiction of T1-sensitive material and solid viscera including meconium, hemorrhage, placenta, bowel, liver, spleen, thyroid gland and the pituitary gland.
For all 3-dimensional FMRI acquisitions, it is imperative to prescribe the parameters and image coverage such that the scan time is <10 to 15 sec, while spatial resolution and SNR are maximized. Depending on the gestational age, potential space in the amniotic cavity and the maternal fasting status, scan times >10 to 15 sec will increase the potential opportunity for fetal motion (Figure 3). Exam coverage should be restricted to no more than the boundaries of the uterus. Thin-section images are ideal for high spatial resolution, but at the expense of longer scan times and reduced SNR. Increasing the matrix and repetition time will improve SNR, but will also increase the scan time.
Image display and post-processing
Advanced visualization techniques are fundamental to 3-dimensional FMRI clinical applications (Table 4). To maximize the clinical potential of these sequences, it is essential that either the interpreting radiologist or a trained technologist has the understanding and skill to employ workstation techniques and tool functions to manipulate the datasets in multiple projections and formats and perform accurate 2- and 3-dimensional quantitative analyses. The post-processing operator should also understand the unique challenges in applying workstation techniques and tool functions to fetal and related gestational anatomy.
The 3-dimensional MRI datasets are transferred to a workstation, which may be integrated into a picture archiving and communications system (PACS) or exist as independent systems (thin-client or standalone units). For state-of-the-art workstations, there are 6 visualization techniques which can be applied for 3-dimensional FMRI post-processing (Table 4): multiplanar reformation (MPR), curved planar reformation(CPR), thick MPR (Ray-Sum), volume rendering (VR), maximum intensity projection (MIP) and minimum intensity projection (MinIP). Each are applied in a complementary manner, based upon the operator’s need for structural detail vs. structural overview, and the advantages that each particular technique provides — applications are avoided in situations which expose the disadvantages of a technique. From this perspective, interactive use of the techniques forms the basis for real-time interpretation and display, while generation of protocol-driven post-processed images and batch reconstructions enables PACS review of static fetal anatomical displays along with review of reformatted coronal, sagittal and oblique fetal projections. It is important to keep in mind that the success of applying each of these techniques is dependent upon the image quality of the 3-dimensional FMRI sequence (Figure 3).
MPR is the core technique for multiplanar assessment of structural detail and generation of 2-dimensional and volumetric (organ,lesion or whole body) measurements (Figures 3 and 4). By nature of this technique, the operator “slices” through the volumetric data with imited spatial perception. Longitudinal cross-sectional 2-dimensional CPR static or rotational displays can improve spatial resolution of gestational anatomy, without loss in detail. CPR images are produced by manually drawing a centerline through the anatomical region of interest.
VR, MIP, MinIP and Ray-Sum are applied to convey fetal spatial detail and structural relationships (Figures 5 and 6). All are dependent upon either pre-rendering editing to remove structures which may degrade visualization, using cut-planes (slabs) to slide through targeted gestational anatomy with interactive rotation, magnification, and window and level settings, or a combination of both approaches. Of these four techniques, VR is a true 3-dimensional technique, generating comprehensive fetal spatial displays, with operator-defined transfer of color, light and intensity, as a function of the voxel signal. Its clinical utility and image quality are directly dependent upon the degree of SNR and the amount of “free” amniotic sac space (Figures 5C, 7-9), and indirectly upon the degree of fetal motion. Reduced space is found with older gestational age (e.g. 28 to 30 weeks), oligohydramnios, and multiple gestations. In these situations, a smaller size cut-plane and narrower window-level parameters are required, restricting the anatomical extent and detail of VR displays. The other techniques project a summation of volumetric data into 2-dimensional displays. MIP projects voxels with the most intense signal, MinIP projects those with the lowest signal, and Ray-Sum projects the average signal for voxels. Based upon these principles, MIPs with a 3-dimensional SSFP are most useful when applied with thin-slabs (5 to 10 mm) for depiction of extended fetal anatomical regions and assessment of organ integrity and position (Figures 9C, 10C-D, 11). With a 3-dimensional VI sequence, a thick slab (25 to 75 mm) is recommended. MinIP and Ray-Sum are best applied with 3-dimensional SSFP using moderately thin to thick slabs for whole-body fetal displays, providing gross structural localization, position and contour. Thick-slab Ray-Sum is unique in that it affords radiographic-like fetal displays.13
Clinical applications
Fetal position, situs and anatomical relations
Determining fetal position, abdominal and thoracic situs, and visceral localization are key to recognizing and understanding fetal abnormalities. Ray-Sum, MinIP and VR techniques can be applied to determine fetal position. Manipulation of VR window-level settings or alternatively applying MPR or thin-slab MIP are the techniques of choice to then assess thoracic and abdominal situs and anatomical relations (Figures 5, 10, 11).
Placenta and umbilical cord
Three-dimensional visualization with MPR, thin-slab MIP and/or VR techniques is particularly useful in evaluating the placenta and umbilical cord (Figures 1, 2, 5). Spatial understanding of these structures is fundamental to recognizing their normal structure and potential pathology. Placental evaluation (3-dimensional VI and 3-dimensional SSFP sequences) should assess its location with regards to the amniotic sac and cervix (e.g. placenta previa) and exclude hemorrhage (Figure 12A), abnormal implantation (e.g. placenta accreta, increta or percreta), accessory lobes (e.g. succenturiate lobe, Figure 12B), and masses (e.g. chorioangioma, Figure 7). Cord evaluation (3-dimensional SSFP) should address its insertion site (Figure 7, 12B), length, course (Figures 7, 10), number of vessels, and potential masses.7,9
Central nervous system
Multiplanar, anatomical projections are essential for assessment of the fetal supratentorium, posterior fossa, brain stem and spinal cord and canal. The 3-dimensional SSFP sequence is valuable in this regard to manipulate 1 sequence and swiftly depict gross anatomy (Figure 1, 13B) and pathology, as well as quantify fetal biometry. Depending upon the acquisition’s spatial resolution and degree of motion, as well as the size of the abnormality, dorsal induction (encephalomyelocele); ventral induction (holoprosencephaly); cellular division and differentiation; migration anomalies; and ventricular system abnormalities may all be defined more efficiently with MPR and thin-slab MIP (Figure 9C).14 The 3-dimensional VI sequence is reserved for evaluation of the pituitary gland (Figure 2).
Craniofacial and neck
Similar to 3-dimensional US, 3-dimensional SSFP sequences can generate detailed VR surface images of normal craniofacial anatomy(Figure 13A) and anomalies, including cleft lip, cleft jaw, cleft palate, micrognathia (Figure 14), hypertelorism and hypotelorism.14 Once localized with VR, the abnormality is assessed with MPR, thin-slab MIP or both. Fetal neck pathologies which may lend themselves to 3-dimensional FMRI evaluations include vascular malformations (e.g. lymphangiomas), masses (e.g. teratomas) and thyroid goiters.7,14 The fluid-filled airway in the neck is readily assessed with 3-dimensional SSFP to exclude intrinsic (e.g. congenital high airway obstruction syndrome,CHAOS; Figures 15A-B) or extrinsic obstruction (e.g. masses). Three-dimensional VI can be applied to evaluate the thyroid gland (Figure 2)and potentially could be relied upon to exclude hemosiderin deposition in the neck (e.g. hemorrhage, vascular malformation).
Thorax
Common thoracic fetal pathology evaluated with MRI includes space-occupying lesions e.g. congenital pulmonary airway malformation (Figure 3), congenital diaphragmatic hernia (Figure 10), and bronchial atresia (Figure 16). Along with the intrathoracic airway and pleural spaces (Figure 15C), each can be evaluated with the 3-dimensional SSFP sequence using MPR and thin-slab MIP. This sequence can also be used for qualitative and quantitative evaluation of fetal lung volumes and the position of the liver, providing prognostic information for pulmonary development and fetal survival.7
Cardiovascular system
Fetal echocardiography remains, at present, the modality of choice for evaluation of fetal congenital heart and vascular disease. Two-dimensional SSFP has been shown to be a feasible means to evaluate CHD along with extracardiac anomalies, serving a complementary role to echocardiography.6,15 By acquiring the SSFP sequence volumetrically (e.g. 3-dimensional SSFP), cardiac and vascular morphology can be displayed in anatomical planes using MPR and thin-slab MIP, without projection artifacts (Figures 4, 11, 17).
Gastrointestinal system
For complete 3-dimensional FMRI evaluation of the gastrointestinal system, both the volume-interpolated and SSFP sequences should be acquired. Using a combination of thin-slab MIP and VR techniques, 3-dimensional VI is applied exclusively to assess the meconium filled colon (Figures 2, 10, 12A).7,12,16 Three-dimensional SSFP is used for all other applications, including fetal biometry (Table 2), with MPR, MIP and/or VR techniques (Figures 6, 18).17
Genitourinary system
Due to the inherent nature of fluid in the genitourinary system, 3-dimensional evaluation of the kidneys, ureters, bladder and urethra is readily achieved with 3-dimensional SSFP (Figure 19). High tissue contrast yields robust MPR, MIP and VR reconstructions, such that renal hypoplasia, agenesis, duplication and urinary tract obstructions with hydro-ureter, hydronephrosis, or both, can all be assessed in 3-dimensional sequences.18 Additional applications include evaluation of adrenal and renal masses, renal cystic disease, urinoma and gender morphology (Figures 10 and 20).
Musculoskeletal system
Evaluation of the spine, long bones, hands and feet is important in the assessment and exclusion of skeletal dysplasias and limb anomalies. A 3- dimensional SSFP sequence is manipulated to display complete and continuous anatomic axial and appendicular segments using Ray-Sum, MinIP, VR, MPR and/or MIP techniques (Figures 1D, 1J, 5A-C, 7D-E, 8). As demonstrated with 3-dimensional fetal ultrasound,19 the anatomical displays enhance skeletal visualization and surveys, providing greater diagnostic confidence and understanding of the type and extent of abnormalities. Three-dimensional SSFP is also valuable for the assessment of soft-tissue masses.
Conclusion
Volumetric 3-dimensional FMRI sequences are useful for displaying fetal anatomy and recognizing pathology in a wide spectrum of clinical applications. Successful utilization requires optimized image quality and understanding of advanced visualization techniques. Continued evidence-based investigation is required to determine the impact of 3-dimensional FMRI on fetal diagnosis and management.
REFERENCES
- Goncalves LF, Lee W, Espinoza J, Romero R. Three- and 4-dimensional ultrasound in obstetric practice: Does it help? J Ultrasound Med. 2005;24:1599-1624.
- de Paula CF, Ruano R, Campos JA, Zugaib M. Placental volumes measured by 3-dimensional ultrasonography in normal pregnancies from 12 to 40 weeks’ gestation. J Ultrasound Med. 2008;27: 1583-1590.
- Chan LW, Fung TY, Leung TY. Volumetric (3D) imaging reduces inter- and intraobserver variation of fetal biometry measurements. Ultrasound Obstet Gynecol. 2009;33:447-452.
- Merce LT, Barco MJ, Bau S. Three-dimensional volume sonographic study of fetal anatomy: Intraobserver reproducibility and effect of examiner experience. J Ultrasound Med.2008;27:1053-1063.
- Behairy NH, Talaat S, Saleem SN, El-Raouf MA. Magnetic resonance imaging in fetal anomalies: What does it add to 3D and 4D US? Eur J Radiol 2010;74:250-255.
- Manganaro L, Savelli , di Maurizio M, et al. Assessment of congenital heart disease (CHD):Is there a role for fetal magnetic resonance imaging (MRI)? Eur J Radiol 2009;72:172-180.
- Pugash D, Brugger PC, Bettelheim D, Prayer D. Prenatal ultrasound and fetal MRI: The comparative value of each modality in prenatal diagnosis. Eur J Radiol. 2008;68:214-226.
- Prayer D, Brugger PC, Prayer L. Fetal MRI: Techniques and protocols. Pediatr Radiol. 2004;34: 685-693.
- Levine D. Obstetric MRI. J Magn Reson Imaging. 2004;24:1-15.
- Hatab MR, Zaretsky MV, Alexander JM, Twickler DM. Comparison of fetal biometric values with sonographic and 3D reconstruction MRI in term gestations. AJR Am J Roentgenol.2008;191:340-345.
- Anquez J, Angelini E, Bloch I, et al. Interest of the steady state free precession (SSFP) sequence for 3D modeling of the whole fetus. Conf Proc IEEE Eng Med Biol Soc. 2007:771-774.
- Inaoka T, Sugimori H, Sasaki Y, et al. VIBE MRI for evaluating the normal and abnormal gastrointestinal tract in fetuses. AJR Am J Roentgenol. 2007;189:W303-308.
- Huisman TA, Solopova A. MR fetography using heavily T2-weighted sequences: Comparison of thin- and thick-slab acquisitions.Eur J Radiol. 2009;71: 557-563.
- Herman-Sucharska I, Bekiesinska-Figatowska M, Urbanik A. Fetal central nervous system malformations on MR images. Brain Dev. 2009;31:185-99.
- Manganaro L, Savelli S, Maurizio M, et al. Fetal MRI of the cardiovascular system: Role of steady-state free precession sequences for the evaluation of normal and pathological appearances. Radiol Med. 2009;114:852-870.
- Rubesova E, Vance CJ, Ringertz HG, Barth RA. Three-dimensional MRI volumetric measurements of the normal fetal colon. AJR Am J Roentgenol. 2009;192:761-765.
- Veyrac C, Couture A, Saguintaah M, Baud C. MRI of fetal GI tract abnormalities. Abdom Imaging. 2004;29:411-420.
- Hormann M, Brugger PC, Basassy C, et al. Fetal MRI of the urinary system. Eur J Radiol. 2006;57: 303-311.
- Nelson TR, Pretorius DH. Visualization of the fetal thoracic skeleton with three-dimensional sonography: A preliminary report. AJR Am J Roentgenol. 1995;164:1485-1488.