CT Angiography: Essential for evaluating atherosclerotic cardiovascular disease
Images
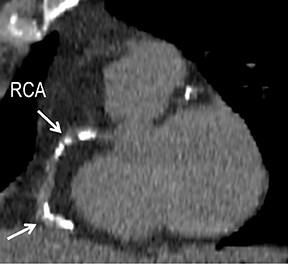
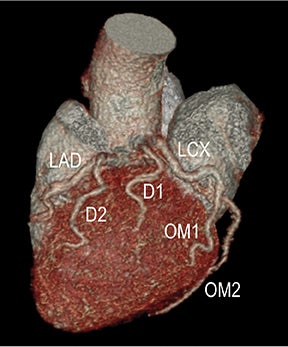
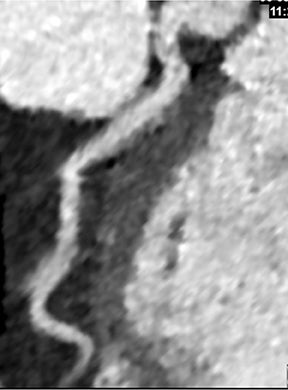
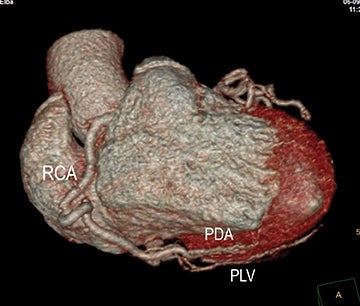
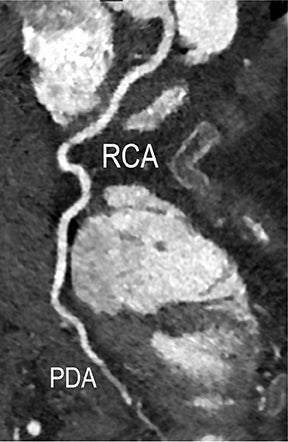
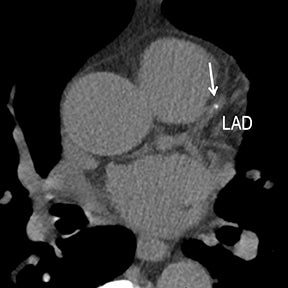
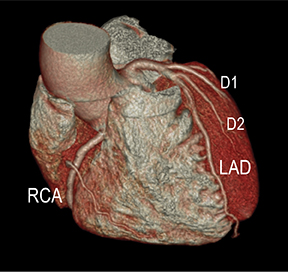
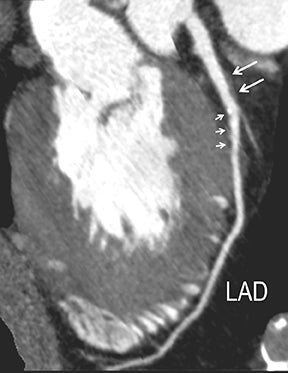
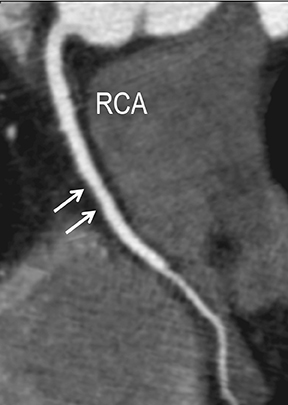
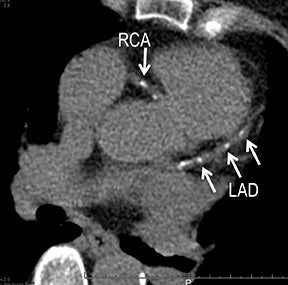
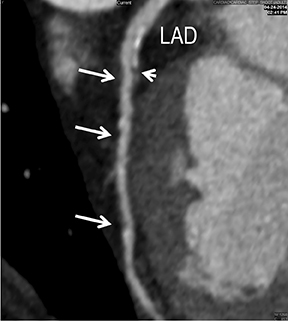
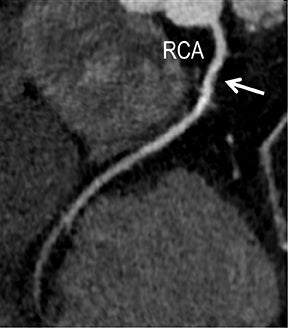
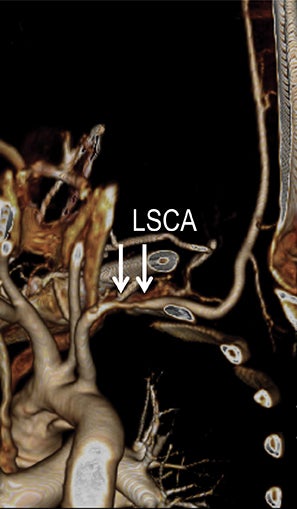
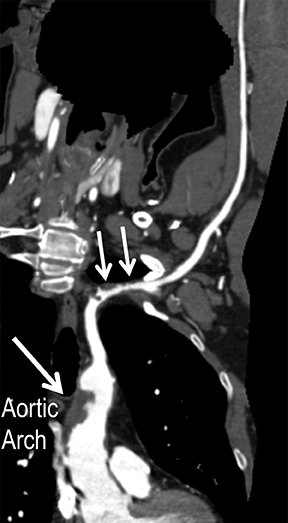
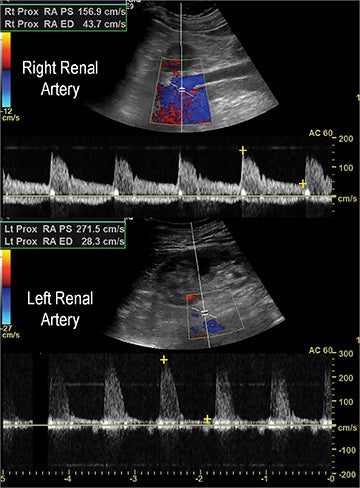
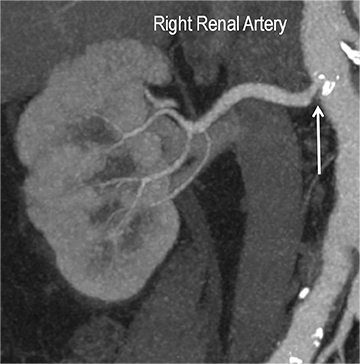
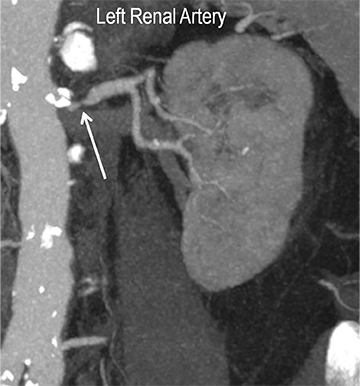
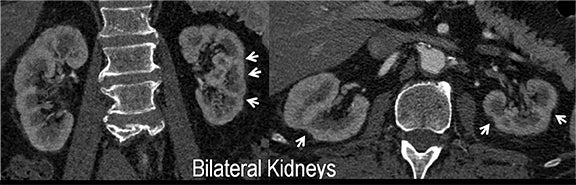
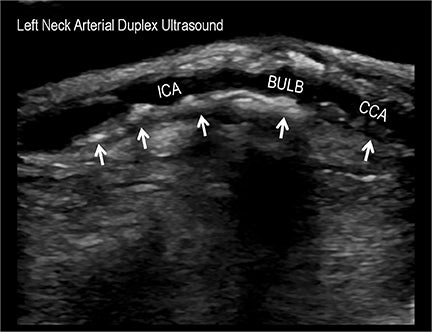
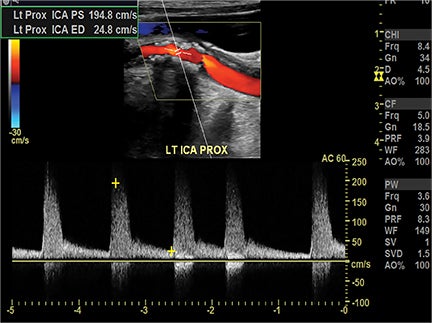
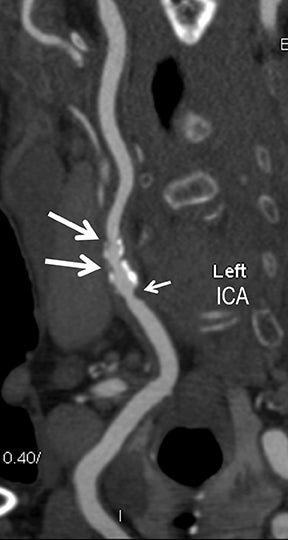
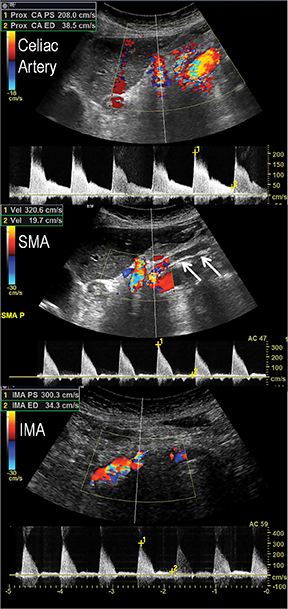
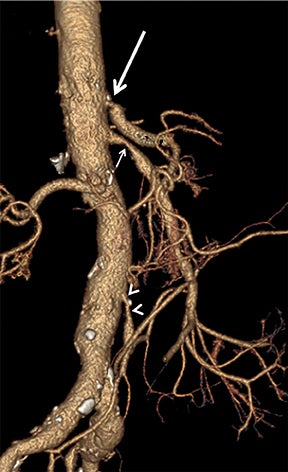
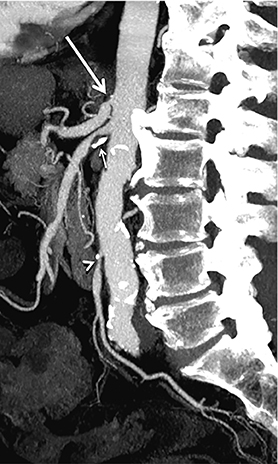
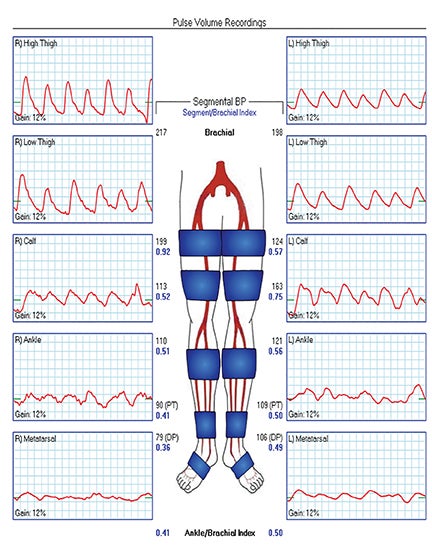
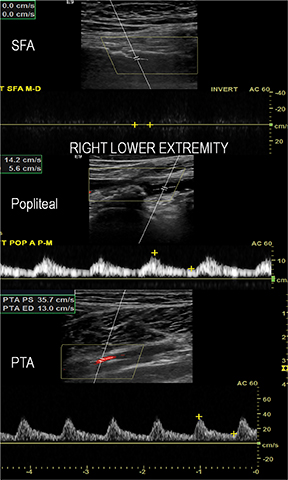
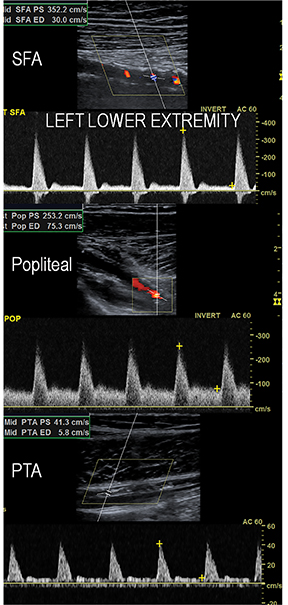
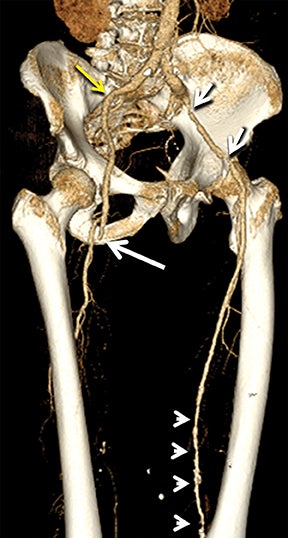
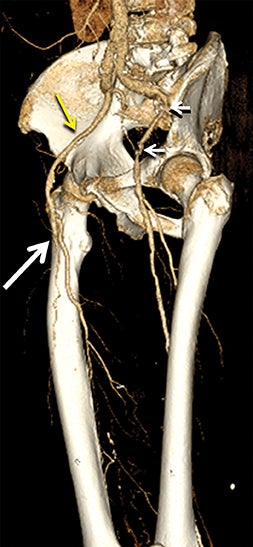
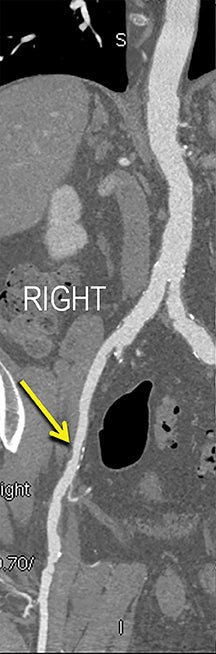
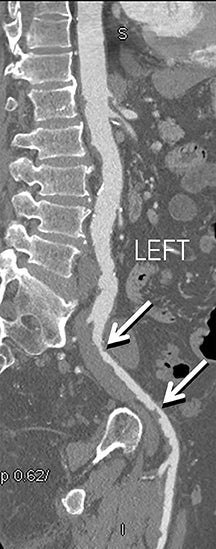
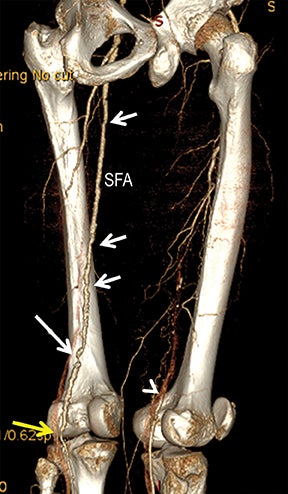
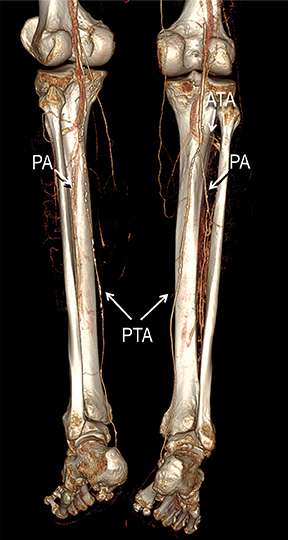
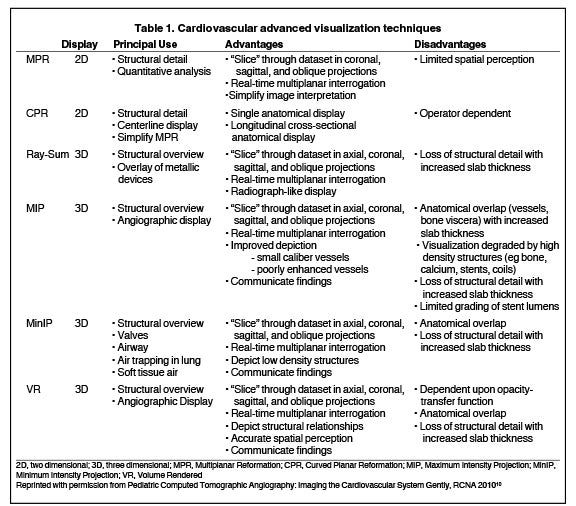
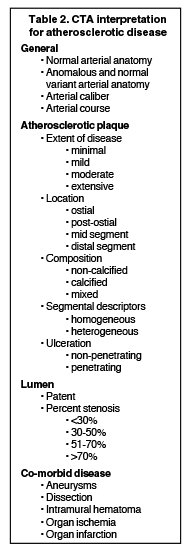
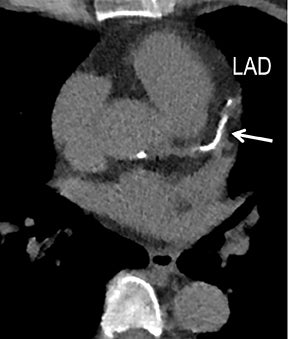
As a global arterial pathologic process, atherosclerotic cardiovascular disease (ACVD) affects all body systems. The heart, brain, abdominopelvic viscera, and limb structure and function are all at risk for secondary disease, depending on a patient’s inheritable genetic and acquired risk factors.1-2 With this potential extent of disease, atherosclerosis continues to be a leading cause of patient morbidity and mortality and a significant underlying source of hospitalizations and healthcare expenditures, particularly in patients with a higher number of involved cardiovascular territories as well as in patients with late presentation of disease and end-organ damage with impaired function.1,3-5 In recent years, preventive medicine, early diagnosis, and advances in effective medical, endovascular, and surgical strategies have led to decreasing cardiovascular disease morbidity and mortality. Between 2000 and 2010, the American Heart Association has reported, mortality per 100,000 people declined 30%, falling from 0.34% to 0.24%.1 With early diagnosis and primary and secondary preventive management, there is also a trend towards decreasing intensity of healthcare services for cardiovascular disease,2-4 which in the long term can potentially reduce healthcare expenditures and generate more effective utilization of scarce healthcare resources.
Diagnostic imaging plays a fundamental role in screening, accurately diagnosing, and planning effective management for ACVD. In current practice guidelines for the evaluation of coronary arterial disease (CAD), cerebrovascular disease (CVD), and peripheral arterial disease (PAD), echocardiography and arterial duplex sonography are cornerstone first-line imaging modalities.6-8 Assessing arterial pressure indices, segmental pressures, and pulse volume recording (PVR) are additional primary diagnostic tests in evaluating upper- and lower-extremity PAD.8 For advanced arterial imaging requirements beyond echocardiography and arterial duplex sonography, until 20 years ago, catheter-based angiography had been the standard practice for diagnostic evaluation of ACVD. With rapid, continuous technological advancements since the 1990s, current guidelines now recommend computed tomographic (CT) or magnetic resonance (MR) angiography for most clinical scenarios, with catheter angiography being reserved for when noninvasive imaging is non-diagnostic, indeterminate, or not available.6-8 Concurrently, non-contrast coronary artery calcium scoring (CCS) CT has evolved as a core tool for detecting and risk stratifying cases of CAD.6,9
Initially, the relatively high radiation dose exposure was a main obstacle to the adoption of CT angiography (CTA). For this reason, despite CTA’s superior spatial resolution, faster patient throughput, greater patient comfort and satisfaction, and lower cost, early consensus statements recommended MR over CT angiography. The aggressive application of dose-reduction strategies and the introduction of iterative reconstruction both at the CT acquisition and image workstation levels have dramatically changed this position10-12 and are leading to continued growth in CTA clinical use. Reliable high-quality CTA can now be achieved routinely at low and ultra-low dose exposure in evaluating CAD, CVD, and PAD without hurting diagnostic performance. By using dose-reduction strategies and iterative reconstruction with a 64-channel multidetector-row CT (MDCT) in our CTA practice, patients receive as low as 1-chest-radiograph-equivalent dose exposure for coronary CCS (Figure 1), 3-radiograph-equivalent dose exposure for coronary CTA (CCTA, Figure 2), and 3-to-10-radiograph-equivalent exposure for vascular CTA (VCTA) depending upon coverage. This is significantly lower than early simulated and actual performance, when authors reported as high as 50- to 100- and 30- to 50-radiograph-equivalent exposure, respectively, for CCTA and VCTA.13
With appropriate dose-reduction strategies and iterative reconstruction, cardiovascular CTA has the potential to dramatically impact ACVD detection and management with the goal of increasing preventive strategies, intervening with less invasive therapies, and reducing rates of myocardial infarction, stroke, visceral ischemia and infarction, and limb tissue loss and amputation. To enhance disease detection while controlling healthcare costs, imagers must understand how cardiovascular CTA is integrated into clinical practice guidelines. This article presents an overview of atherosclerosis pathophysiology and CTA technique, followed by a clinical review of diagnostic CTA with an emphasis on CAD, CVD, and PAD guidelines for diagnosing ACVD.
Atherosclerosis pathophysiology
Whether in the coronary, cerebrovascular, or peripheral arterial circulations, the pathophysiology of atherosclerosis is similar. Lipoprotein deposition in the arterial intima leads to accumulation of lipid-laden macrophages and an inflammatory response with the release of cytokines, oxidants, and matrix metalloproteinases. This leads to smooth muscle cell (SMC) migration into the intima and proliferation of an extracellular matrix (ECM) that surrounds the lipid material. The result is the formation of atheromatous plaque, defined by its central lipid core and an encapsulating fibrous cap of connective tissue. Calcium forms in the fibrous cap during the healing process and typically signifies remote stable plaque with more organized SMC and ECM, and associated arterial wall stiffening. In distinction, vulnerable (eg, unstable) plaque has a greater degree of lipid-laden macrophages and a less robust ECM fibrous cap (eg, non-calcified), increasing the risk for plaque rupture and in-situ thrombus formation.7-8
In the early phase, plaque initially causes positive arterial wall remodeling (Figure 3). However, as a lesion expands, negative remodeling and luminal stenosis will occur (Figures 3-4). This process is accentuated by intra-plaque hemorrhage and plaque rupture with resultant thrombus formation. When there is critical obstructive stenosis (eg, progression in luminal narrowing) or occlusion (eg, from plaque rupture and lumen thrombosis), symptoms will manifest from impaired organ perfusion (eg, ischemia), cellular hypoxia, and tissue damage (eg, infarction). Alternatively, plaque thromboembolism may occur, leading to obstructed flow in the downstream circulation and secondary impaired organ perfusion. While this review is limited to discussion of obstructive atherosclerotic disease, additional manifestations of atherosclerosis include penetrating ulcers, dissection, and aneurysms.
Intrinsic risk factors for ACVD include a family history for atherosclerosis, hyperlipidemia, hypertension, and diabetes. Behavioral risks include tobacco use, obesity, sedentary lifestyle, and an unbalanced, “unhealthy” cardiometabolic diet.1-2,8,14 Primary and secondary management aim to promote cardiovascular health and reduce cardiovascular risk. Behavioral strategies include cessation of smoking, adherence to recommended cardiovascular dietary and physical activity guidelines, and reduction of body weight to an ideal body mass index. Key pharmacologic strategies target the control of hyperlipidemia, hypertension, and diabetes.1-2,8 Secondary pharmacologic management includes antiplatelet therapy to reduce the potential for thrombus formation.8
Computed tomographic angiography
Technical acquisition
Current standard practice CTA for CAD, CVD, and PAD is achieved by synchronizing a high-flow, intravenous, iodinated contrast medium injection with a rapid, submillimeter, isotropic resolution MDCT acquisition timed to the cardiac or arterial phase of interest. From this volumetric dataset, two-dimensional (2D) and three-dimensional (3D) images are generated, the quality of which inherently depends on precise implementation of the CTA protocol and success of the acquisition. Coronary CTA is acquired with either prospective (eg, structure) or retrospective (eg, structure and function) ECG gating, while routine vascular CTA is acquired with non-ECG-gated helical technique. Per practice guidelines, cardiac CTA should be performed on an MDCT scanner with at least 64 channels of image data and a gantry rotation no greater than 420 milliseconds.15 While vascular CTA can be performed on MDCT scanners with fewer than 64 channels, utilizing at least 64-channel technology is recommended to optimize core radiation dose-reduction strategies and to employ the lowest possible volume of contrast, as demanded by patient weight. Appropriate CTA use mandates patients have normal renal function, have no allergies to iodinated contrast, and have a body weight which will not impair CTA quality or lead to excessive radiation dose exposure. For cardiac CTA, patients should also have a regular heart rate and rhythm, should have no allergies or other contraindications to ß-blockers or nitroglycerin, and should be able to suspend respiration upon command.15
CCS MDCT (Figure 1) is performed without contrast, acquiring standardized prospective ECG-gated 3.0 mm images to detect and score coronary calcium. CCS acquisitions may be performed independently or as part of a coronary CTA examination. Before acquiring a vascular CT arteriogram, unenhanced preliminary non-ECG-gated thicker images (1.25-5.0 mm) may be obtained. These images aid CTA interpretation by revealing atherosclerotic and luminal thrombus calcifications, blood products (eg, intramural hematomas), endovascular stents and stent grafts, surgical clips, and surgical grafts.
Dose-reduction strategies should always be employed to maximize CTA benefit over radiation risk while maintaining diagnostic quality with acceptable noise. Acceptable noise (and thus how low radiation can be targeted) will vary among providers.Reaching a balance is important, as excessive noise may diminish the accuracy of interpretation as well as confidence in using the exam for management decisions. Radiation dose-reduction strategies include iterative reconstruction (eg, statistical or modeled-based iterative techniques, Figures 1-2), the lowest possible voltage (eg, 70- 80kVp vs 100kVp vs 120kVp; Figure 2), the lowest possible amperage (eg, weight-based mAs), the minimum amount of coverage, and the shortest possible scan time (eg, fastest scan rotation, high-pitch helical, wide-collimation helical, and volumetric MDCT techniques).10-12 Contrast-medium delivery is an important way to accommodate increased noise and maintain a diagnostic contrast-to-noise ratio.10
Interpretation and advanced visualization
CTA image display and interpretation for ACVD are most effectively achieved when applying advanced visualization techniques (Table 1). This is particularly important with large-volume datasets (eg, upper- and lower-extremity CTA), complex atherosclerotic plaque (eg, high calcium burden with mixed composition plaque), and tortuous arterial segments (Figure 5). As detailed in Table 2, the interpretive objectives in evaluating ACVD are to recognize and characterize levels of luminal patency, obstructive stenosis, and occlusion while 1) recognizing aortic and arterial anomalies and normal variant anatomy, 2) defining the caliber and course of normal and abnormal arterial segments, 3) characterizing atherosclerotic plaque severity, composition, and potential ulcerations, and 4) excluding aneurysms, dissection, intramural hematomas, and other potential aortic and arterial complications. When obstructive disease is detected, the presence and extent of collateral pathways (eg, downstream reconstitution and perfusion) should be defined. Evaluating end organs in a cardiovascular territory is equally important. Abnormal tissue enhancement in a normal-size organ would indicate active ischemia and decreased tissue perfusion, while global atrophy with diminished enhancement would indicate chronic ischemia and decreased function (Figure 6). Loss of segmental parenchyma in a defined arterial territory would indicate prior infarction (Figure 6). Identification of fat attenuation in an infarcted region with hypoenhancement would confirm scar and not peri-infarct ischemia.
Advanced visualization techniques are selected according to their strengths, utilizing adjustable angiographic window and level settings. This includes a wide window setting to account for noise and high arterial contrast, which may be encountered with low-dose CTA protocols. For highest accuracy and performance, it is recommended that the interpreter engage the CTA dataset in real time on a workstation with user-defined techniques and tool functions as necessary for visualization and interpretation, rather than relying upon static processed images. 3D volume rendering (VR) and maximum intensity projections (MIP) provide an understanding of the spatial relationships and global arterial anatomy in a circulatory territory (Figure 5). Structural detail is interrogated using 2D multiplanar (MPR) and curved planar (CPR) reformations (Figure 7). Orthogonal views should be generated to precisely grade the severity of luminal stenosis, characterize atherosclerotic plaque, and confirm the presence of ulcerations. This is most efficiently achieved with rotating CPRs (Figure 7). Interpretations based on advanced visualization techniques should always be confirmed by cross referencing back to the source images to minimize false-positive or false-negative interpretations. Although minimum intensity projection (MinIP) and Ray-Sum (thick MPR) have limited CTA applications, MinIP is useful for showing cardiac valves (eg, the bicuspid aortic valve), while Ray-Sum is useful for overlaying cardiovascular metallic devices with a 3D display.
CTA clinical utilization
Coronary arterial disease
Angina symptoms related to obstructive CAD may include chest pain, chest tightness, chest burning, shoulder pain, jaw pain, radiating arm pain, epigastric discomfort, nausea, and new electrocardiographic abnormalities. Dyspnea and worsening exercise tolerance are considered as non-chest-pain angina equivalents. Patients presenting with suspected ischemic heart disease secondary to obstructive CAD are assessed for their overall probability of having obstructive disease. Based upon their cardiovascular risk factors and clinical presentation, patients may be categorized as having a low pretest (<10%), intermediate pretest (10%-90%), or high pretest (>90%) probability for obstructive CAD.9
Noninvasive diagnostic evaluation for ischemic heart disease secondary to obstructive CAD may include stress ECG testing, stress echocardiography, rest-stress myocardial perfusion nuclear scintigraphy, stress cardiac MR imaging, coronary CTA, and CCS CT. Coronary CTA is recommended for low- and intermediate-risk patients. In non-acute patients, stress ECG, echocardiography or nuclear scintigraphy has often been performed prior to undergoing CTA. In acute chest pain patients, coronary CTA is becoming an effective primary modality for the exclusion of obstructive CAD.16 Non-contrast CCS CT is recommended in both low-risk patients with a family history for ischemic heart disease and in intermediate-risk patients. For an extensive overview of recommended appropriate clinical scenarios for coronary CTA and CCS, consult the 2010 multidisciplinary appropriate use document for cardiac CT.9 Appropriate clinical utilization of coronary CTA and CCS is further illustrated in Figures 1-4.
Cerebrovascular disease
Symptomatic intra- and extracranial carotid and vertebral ACVD may manifest clinically as acute focal stroke, transient ischemia, monocular blindness, cerebral ischemia, or a combination thereof. Additional nonfocal symptoms that could suggest abnormal intracranial perfusion may include transient global amnesia, acute confusion, syncope, isolated vertigo, dizziness, weakness, and paresthesias. Extracranial carotid and vertebral ultrasound is indicated in symptomatic patients to assess for obstructive arterial atherosclerotic disease.7 Duplex arterial sonography is indicated in asymptomatic patients with known or suspected extracranial disease based upon the presence of risk factors, documented CAD or PAD, and/or the presence of a bruit.7 As illustrated in Figure 7, CT angiography is indicated to evaluate extracranial carotid and vertebral arteries when duplex sonography is either equivocal or nondiagnostic. In addition, CTA may be performed to evaluate the intracranial circulation, when ultrasound has excluded obstructive disease in the extra-cranial arteries.
Peripheral arterial disease
Mesenteric ischemia
Acute and chronic mesenteric ischemia occur secondary to compromised blood flow and oxygen delivery to the bowel. The impact of decreased intestinal perfusion may range from transient ischemia to significant tissue necrosis with bowel-wall thickening, pneumotosis and portal venous gas, depending upon burden of disease, degree of luminal obstruction, extent of collateral networks, and timeliness of diagnosis. In patients with ACVD, acute mesenteric ischemia results from either plaque rupture and in-situ complete thrombosis, or occlusive thromboembolism from proximal plaque. Patients will present with severe mid-abdominal pain, often with nausea and vomiting. If diagnosis and intervention are not promptly made, ischemia will rapidly lead to infarction, perforation, and peritonitis, as there is no sufficient collateral supply. In distinction, patients with chronic mesenteric ischemia secondary to ACVD typically present with weight loss, abdominal angina, and food fear. Plaque results in hemodynamically significant stenosis, occlusion, or both, involving at least two of the three mesenteric arteries supplying the intestines. Disease progresses gradually, allowing collateral circulation to develop and support intestinal perfusion. As physiologic demands outweigh collateral flow, symptoms will progressively become clinically significant.
In the acute setting, CT angiography has emerged as the recommended primary imaging modality for prompt and thorough evaluation; duplex arteriography is regarded as an inappropriate imaging modality.8 With MDCT angiography, mesenteric arterial occlusion, arterial embolism, absence of bowel-wall enhancement, pneumotosis intestinalis, venous gas, bowel-wall thickening, and solid organ infarctions can all be defined with high sensitivity and specificity.16 In chronic mesenteric ischemia, duplex arteriography, CTA, and MRA are all recommended as potential initial diagnostic options.8 Early performance with MDCT angiography established its utility to identify critical obstructive disease and occlusions, map out key collateral pathways, and evaluate organ structure.17 The case illustrated in Figure 8 highlights the integrated clinical roles of duplex arteriography and CTA in evaluating chronic mesenteric ischemia.
Renal stenosis
Renal artery stenosis (RAS) accounts for approximately 1%-5% of cases of hypertension, with most cases resulting from atherosclerosis (90%).18 Uncontrolled RAS can lead to progressive deterioration in renal function, loss of renal parenchyma, and ultimately, chronic and end-stage renal disease (Figure 6). Early diagnosis is essential so that appropriate endovascular or surgical interventions can restore perfusion, control hypertension, and prevent further loss in renal mass and function. Guidelines recommend screening with duplex arteriography, CTA or MRA to diagnose RAS, when there is 1) new onset hypertension in a patient less than 30 years of age, 2) severe hypertension in a patient greater than 55 years of age, 3) accelerated, resistant, or malignant hypertension, 4) new renal dysfunction, 5) worsening renal function after the administration of an ACE inhibitor, 6) unexplained unilateral or bilateral renal atrophy, and 7) unexplained pulmonary edema.8
Early performance with MDCT established its role for CTA in the evaluation for renal arterial ACVD and RAS. Willman et al found a sensitivity of 86–93%, specificity of 99–100%, and an accuracy of 98–99%.19 The clinical role and value of MDCT angiography in the evaluation of RAS secondary to atherosclerosis is illustrated in Figure 6.
Upper-, lower-extremity disease
Upper- and lower-extremity atherosclerotic arterial disease may manifest clinically as acute or chronic ischemia. Acute ischemia results from primary arterial thrombosis (eg, plaque rupture) or occlusive thromboembolism typically in the outflow distribution, in the absence of sufficient collateral flow. Chronic ischemia arises from progressive obstructive disease in inflow and/or outflow arterial segments. Symptoms may range from impaired limb use and claudication to threatened limbs with ischemic rest pain, ulceration, and gangrene (eg, critical limb ischemia with tissue loss).
Diagnostic anatomic and physiologic techniques include pressure indices (eg, ankle- and toe-brachial indices), segmental pressure measurements, pulse volume recordings, duplex arterial sonography with Doppler waveform analysis, and exercise testing. CTA is recommended to confirm the presence and level of obstructive disease..8 High-resolution CTA 3D reconstructions of the inflow and outflow upper- and lower-extremity circulations are also highly valuable for planning endovascular and surgical revascularization procedures (Figures 5,9).20-21 The authors have reported lower-extremity MDCT angiography sensitivity as high as 96-99% and specificity of 98-99%.21 The clinical utility of lower-extremity CTA integrated with the other diagnostic tests is illustrated in Figure 9.
Conclusions
While early development of MDCT technology broadened clinical application of CT angiography, the drive for patient safety and low radiation dose exposure in this post-modern MDCT era has catapulted CTA to the front lines in the diagnostic evaluation of ACVD. With its ability to produce high-quality low-dose and ultra-low-dose datasets, CTA will continue to grow in clinical utilization. In this manner, CTA will play a greater role in diagnosing ACVD across all systemic arterial circulations and provide an opportunity to increase preventive management, reduce ACVD morbidity and mortality, and enhance the efficient utilization of healthcare resources.
References
- Go AS, Mozaffarian D, Roger VL, et al. Heart disease and stroke statistics – 2014 update. Circulation. 2013;129:e28-e292.
- MMWR Morb Mortal Wkly Rep. Million hearts: prevalence of leading cardiovascular disease risk factors – United States, 2005-2012. 2014; 63: 462-467.
- Smoldern KG, Bell A, Lei Y, et al. One -year costs associated with cardiovascular disease in Canada: Insights from the reduction of atherothrombosis for continued health (REACH) registry. Can J Cardiol. 2010;26:297-305.
- Smoldern KG, Wang K, de Pouvourville G, et al. Two-year vascular hospitalisation rates and associated costs in patients at risk of atherothrombosis in France and Germany: Highest burden for peripheral arterial disease. Eur J Vasc Endovasc Surg. 2012;43:198-207.
- Ademi Z, Liew D, Gorelik A, et al. Predictors of healthcare use among patients with or at high risk of atherothrombotic disease: Two-year follow-up data. Int J Cardiol. 2014;175:72-77.
- Wolk MJ, Bailey SR, Doherty JU, et al. ACCF/AHA/ ASE / ASNC / HFSA / HRS / SCAI / SCCT / SCMR / STS 2013 multimodality appropriate use criteria for the detection and risk assessment of stable ischemic heart disease. J Am Coll Cardiol. 2014;63:380-406.
- Brott TG, Halperin JL, Abbara S, et al. 2011 ASA / ACCF / AHA / AANN / AANS / ACR / ASNR / CNS / SAIP / SCAI / SIR / SNIS / SVM / SVS guidelines on the management of patients with extracranial carotid and vertebral artery disease. Circulation. 2011; 124:e54-e130.
- . Anderson JL, Halperin JL, Alvert NM, et al. Management of patients with peripheral artery disease (compilation of 2005 and 2011 ACCF/AHA guideline recommendations): A report of the American College of Cardiology Foundation/American Heart Association Task Force on Practice Guidelines. Circulation. 2013;127;1425-1443.
- Taylor AJ, Cerqueira M, Hodgson JM, et al. ACCF / SCCT / ACR / AHA / ASE / ASNC / NASCI / SCAI / SCMR 2010 Appropriate Use Criteria for Cardiac Computed Tomography. J Am Coll Cardiol.2010; 56:1864-1894.
- Hellinger JC, Pena A, Poon M, Epelman M. Pediatric CT angiography: Imaging the cardiovascular system gently. Radiol Clin North. 2010;48: 439-467.
- . Hellinger JC, Jacobs S, Salazar P, et al. Image quality improvement using a 3D edge-sensitive noise reduction filter in low dose pediatric retrospective ECG-gated cardiac CT angiography. J Cardiovasc Comput Tomogr. 2011; 5(4S):S6-7.
- Kassam Z, Arifi B, Nura G, et al. Ultra-low dose coronary calcium CT with iterative reconstruction: Enhanced image quality with accurate risk stratification. J Cardiovasc Comput Tomogr. 2013; 7:S52.
- Einstein AJ, Henzlova MJ, Rajagopalan S. Estimating risk of cancer associated with radiation exposure from 64-slice computed tomography coronary angiography. JAMA. 2007; 298:317-323.
- Creager MA, Lüscher TF. Diabetes and vascular disease: Pathophysiology, clinical consequences, and medical therapy: Part I. Circulation. 2003;108:1527-1532.
- Abbara S, Arbab-Zadeh A, Callister TQ, et al. SCCT guidelines for performance of coronary computed tomographic angiography: A report of the Society of Cardiovascular Computed Tomography Guidelines Committee. J Cardiovasc Comput Tomogr. 2009;3:190 –204
- Poon M, Cortegiano M, Abramowicz A, et al. Routine coronary computed tomography angiography reduces unnecessary hospital admissions, length of stay, recidivism rates, and invasive coronary angiography in the emergency department triage of chest pain. J Am Coll Cardiol. 2013 Aug 6;62:543-552
- Hellinger JC. Evaluating mesenteric ischemia with multidetector-row CT angiography. Tech Vasc Interv Radiol. 2004;7:160-166.
- Hellinger JC. Rubin G.D. “Multidetector-row CT Angiography: Technique and Clinical Application.” in: Baum S, (Ed.) Abram’s Angiography, 7th Edition. Lippincott-Williams, 2005.
- Willmann JK, Wildermuth S, Pfammatter T, et al. Aortoiliac and renal arteries: Prospective intraindividual comparison of contrast-enhanced three-dimensional MR angiography and multidetector row CT angiography. Radiology. 2003;226:798–811.
- Hellinger JC, Epelman M, Rubin GD. Upper extremity CT angiography: State of the art techniques and applications in 2010. Radiol Clin North. 2010; 48: 397-421.
- Hiatt MD, Fleischmann D, Hellinger JC, Rubin GD. Angiographic imaging of the lower extremities with multidetector CT. Radiol Clin North Am. 2005;(43): 1119-1127.