Body MR venography: The new gold standard
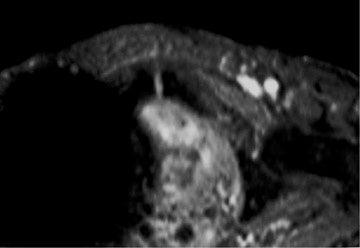
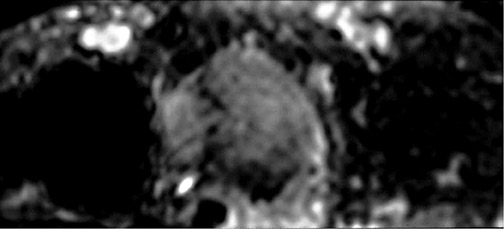
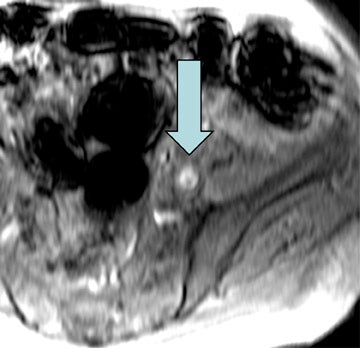
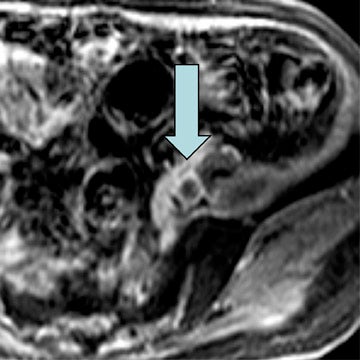
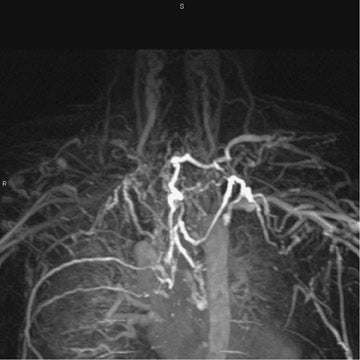
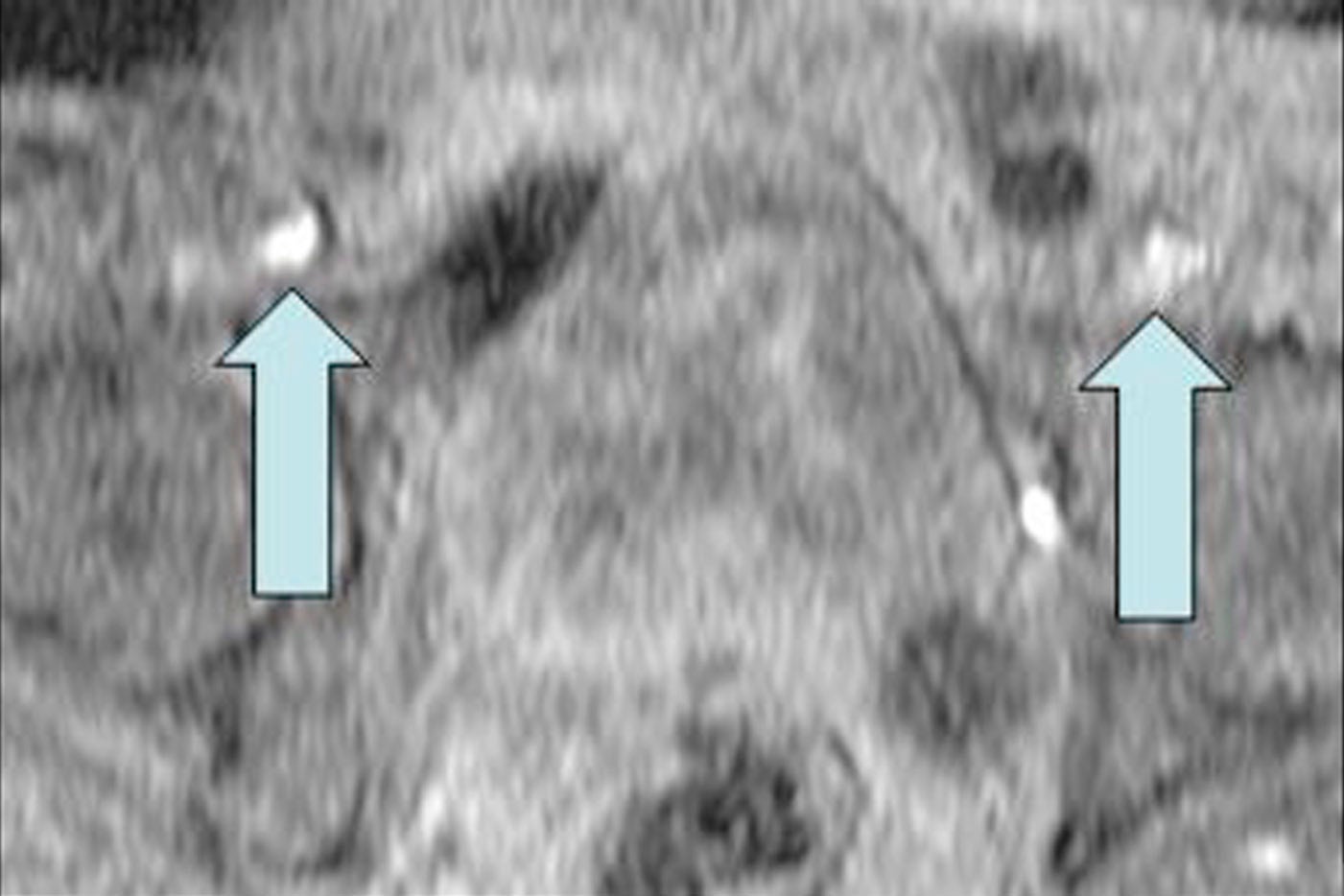
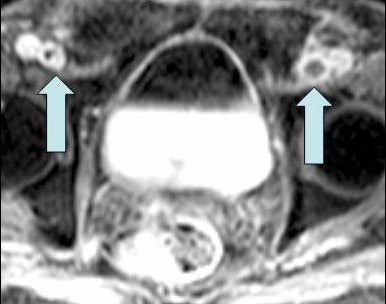
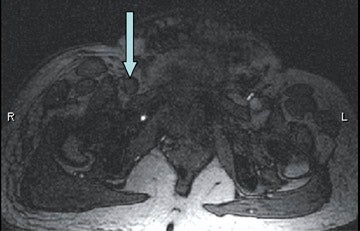
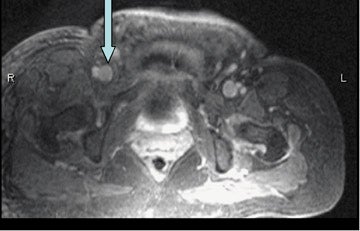
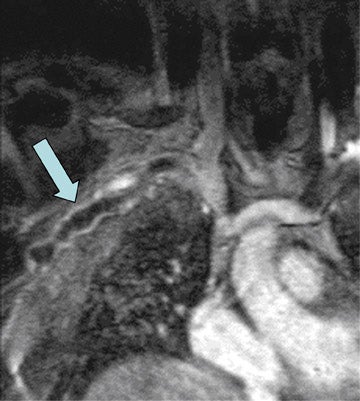
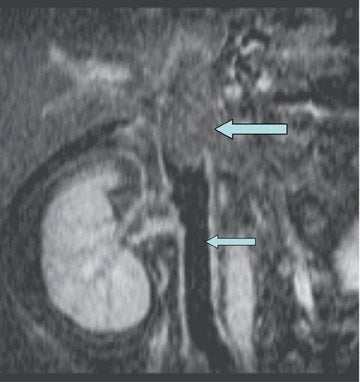
Dr. Krinsky is an Associate Professor of Radiology, New York University School of Medicine, New York, NY.
Magnetic resonance (MR) venography is uniquely suited for evaluation of the veins of the abdomen, thorax, and extremities, as no ionizing radiation is used and contrast agents are non-nephrotoxic. 1-4 MR imaging of the venous system is less hindered by technical limitations and bolus timing that are commonly encountered with imaging the arterial system. In addition, veins deep in the chest and pelvis are readily evaluated with this robust technique.
Conventional contrast-enhanced veno-graphy is an invasive procedure that requires cannulation of a small vein, iodinated contrast, and ionizing radiation. The main role of this technique today is less for diagnosis and more for therapeutic purposes (prior to lytic or interventional therapy). Duplex sonography has become the first-line examination for evaluation of the venous system, especially in the extremities. Sonography, however, is limited by acoustic access, especially in the evaluation of the deep veins of the pelvis, thorax, and calf.
MR venography now plays an important clinical role in the evaluation of venous disease, despite its higher cost and limited availability. In some clinical instances, such as evaluation of the pelvic veins, it has become the test of choice. Advances in local coils, gradient systems, and other system hardware and software have enabled faster scanning, reduced artifacts, increased signal-to-noise, and reduced examination times. Comprehensive examinations of the entire venous system can be performed in <30 minutes.
MR venography techniques
Two-dimensional (2D) time-of-flight (TOF) MR angiography is a widely used, noninvasive technique for evaluation of the venous sys- 5-14 However, due to saturation and flow effects, which may result in nondiagnostic studies, three-dimensional (3D) gadolinium-enhanced gradient-recalled echo (GRE) imaging may be used for problem solving. Alternatively, many centers now forego TOF and go directly to a 3D contrast-enhanced approach.
Time-of-flight
Stationary protons within an imaging volume, such as a vessel wall and adjacent connective tissue, quickly become saturated by the application of rapidly repeated radiofrequency (RF) pulses. Flowing blood that enters the imaging volume does not have the same "excitation history" as stationary tissue. Because it has not been exposed to prior RF pulses, this unsaturated flowing blood generates greater signal intensity than saturated stationary tissues. As a result, the flowing blood appears bright against a dark background.
When a saturation pulse is applied adjacent to the imaging volume, on the side from which arterial blood is flowing, the arterial signal is saturated before it reaches the imaging slice. Venous blood will generate high signal intensity while both the stationary tissue and arterial blood are suppressed and remain dark. The presaturation pulse, known as a traveling saturation band, moves as the 2D imaging slice changes position.
Slice orientation is critical with TOF imaging. Optimally, the imaging plane is positioned orthogonally to the vessel. If positioned parallel to the imaging plane, flowing blood will be exposed to the RF pulses for a longer period of time and may become saturated, a process known as in-plane saturation. This is especially problematic when imaging the great veins of the chest, as the subclavian and axillary veins are "in-plane" with an axial acquisition performed for evaluation of the superior vena cava or jugular veins. Careful attention to selection of slice thickness, orientation, repetition time (TR), echo time (TE), and flip angle will minimize saturation effects. In particular, thinner slices and a longer TR may lessen saturation effects. 1
Time-of-flight imaging may also be degraded by patient motion, magnetic field inhomogeneity, and susceptibility artifacts from adjacent air or metal (Figure 1). Under these circumstances, the short TE (<2 msec ) and small voxel size achievable with gadolinium-enhanced 3D techniques can minimize these causes of artifactual signal loss. The hyperintense signal from acute venous thrombosis may also mimic flow-relat-ed enhancement with TOF imaging, resulting in a false-negative diagnosis of deep venous thrombosis (DVT) (Figure 2). Finally, imaging large blocks of anatomy may result in lengthy examination times.
Contrast-enhanced MR venography
The use of the T1-shortening effect of gadolinium within circulating blood has had dramatic effect on MR angiography of the arterial sys-tem. 15 A similar technique can be used to image the venous system. Because this approach does not rely on flow-related enhancement (only on the decreased T1 of enhanced venous blood), large field-of-view, time-efficient coronal "in-plane" imaging can be performed without saturation effects. This volumetric approach (without imaging gaps) provides high spatial resolution, high signal-to-noise studies resulting in near isotropic 3D data sets that can be reconstructed in any plane. This is an accurate method of evaluating the arterial system, but this method has not been exploited as much in venous imaging, probably because 2D TOF often results in robust image quality and does not require intravenous (IV) access or the additional cost of contrast agents.
Excellent opacification of the venous system is attainable if a longer scan delay time is used (when compared with "first pass" arterial imaging) following injection of gadolinium. Because this is a recirculation technique (vein to artery to vein), a dose of up to 0.2 mmol/kg of gadolinium may be required when imaging multiple anatomic stations (chest/ abdomen/pelvis). For evaluation of a single anatomic station (ie, iliofemoral veins), 0.1 mmol/kg is usually sufficient, injected at 1 to 2 mL/sec. The body coil is adequate for imaging the chest, abdomen, and pelvis and provides faster data reconstruction times than phased-array coils. The TR should be as short as possible, to minimize acquisition time and provide reasonable breath-holds (<25 seconds). The TE should be as short as possible, to minimize magnetic susceptibility effects that can result in signal loss. The flip angle can vary between 15˚ and 60˚. Fat-suppression is helpful in the abdomen and pelvis but may be deleterious in the chest. Magnetic susceptibility effects from aerated lung may result in frequency shifts in adjacent fat- and water-containing tissues. 16 Thus, when a spectrally selective fat-saturation technique is used, regions of water, and not adjacent fat, may be inadvertedly suppressed, resulting in intravascular signal loss. 16
When performing dynamic gadolinium- enhanced 3D GRE studies, several acquisitions are acquired sequentially. The initial acquisition is timed with the gadolinium's first pass through the arterial system but prior to substantial venous enhancement. Multiple acquisitions are performed following the arterial phase; these images usually have both arterial and venous enhancement. A selective venous study can be generated by subtracting the arterial-phase study from a mixed venous-arterial phase study. 17,18 The arterial signal is nullified, while the subtracted data set contains only venous signal.
Direct venography is a novel technique similar to conventional catheter venography in which very dilute gadolinium (5 mL in 250 mL of saline) is injected directly into the distal extremity of expected pathology and imaged with a 3D GRE sequence. 19,20 This can be incorporated with a moving table technique to image from the feet to the inferior vena cava (IVC). 20 However, this technique requires venous cannulation of the affected extremity and cannot demonstrate alternative sites of intravenous access if thrombus or obstruction is identified.
Postprocessing and image interpretation
Evaluation of the source data and reformatted images is essential, as low signal intensity thrombus may not be seen with the maximum intensity projection algorithm. The latter should be reserved for providing images that demonstrate collateral vessels in the setting of extensive thrombosis (Figure 3) or demonstrating alternative sites for achieving central venous access. Differentiating acute from chronic venous thrombosis with TOF imaging may be problematic. However, with gadolinium-enhanced venography, source images demonstrate intense periadventitial enhancement with acute thrombosis (Figure 4). 21 When the clot becomes chronic, this enhancement may no longer be seen, vessel size decreases, and intravascular webs may appear.
Clinical implications
Deep venous thrombosis
The literature supports the claim that MR venography is the "new gold standard" for the diagnosis of suspected DVT. Using 2D TOF, Laissy et al 12 demonstrated that MR venography was as accurate as conventional venography, and more accurate than color Doppler sonography for the diagnosis of lower extremity deep venous thrombosis in 37 patients. In a larger study with 101 patients, Fraser et al 13 confirmed the high accuracy of 2D TOF for iliofemoral and femoropopliteal DVT with sensitivities of 100% and 97%, respectively. However, TOF imaging may be indeterminate for DVT when flow is stagnant, resulting in poor intravascular signal from saturation effects (Figure 5).
Evaluation of the chest and upper extremity veins for venous access
Spontaneous thrombosis of the deep chest veins can be seen in patients with hypercoagulable states and thoracic outlet syndrome. However, the widespread use of long-term indwelling central venous catheters for alimentation, chemotherapy, and hemodialysis has resulted in a marked increase in the number of patients with upper extremity and thoracic DVT. The ideal imaging test would not only detect and quantify clot burden, but also provide alternative sites of access (Figure 6). These goals can be readily achieved with gadolin-ium-enhanced venography. Multiple studies with small numbers of patients have demonstrated the efficacy of this technique for upper extremity DVT. 22-24
Differentiating bland from tumor thrombus
Differentiating bland from tumor thrombus may be critical when staging neoplasms with a propensity for venous extension and for preoperative surgical planning. Direct visualization of thrombus enhancement is a reliable sign for malignancy as bland thrombus will not enhance (Figure 7). Time-of-flight imaging cannot reliably differentiate bland from tumor thrombus, especially when the vein is not expanded.
Conclusion
Contrast-enhanced MR venography is a robust technique that can evaluate the deep veins of the chest, abdomen, and pelvis without the flow artifacts, saturation effects and lengthy examination times inherent in 2D-TOF imaging. Because it does not expose the patient to ionizing radiation or potentially nephrotoxic contrast agents, it is an attractive alternative to computed tomography. Further improvements in hardware and pulse sequences will render this technique the "gold standard" for imaging the deep venous system, especially in anatomic regions that cannot be evaluated with sonography.